DEFINITION
Broadly speaking, the spinal cord is at risk of ischemic injury under any circumstances in which the supply of oxygen or nutrients is insufficient to meet metabolic demands. Thus, severe hypoxia, anemia, or hypoglycemia can cause spinal cord “ischemia” even if SCBF is normal or elevated. However, ischemia is probably more likely to occur because of reduced SCBF, such as that which may result from extrinsic compression of vessels supplying the spinal cord (e.g., epidural hematoma), elevated spinal cerebrospinal fluid (CSF) pressure (e.g., intrathecal injection of a large fluid volume), spontaneous thrombosis of spinal blood vessels, iatrogenic occlusion of vessels supplying the spinal cord (e.g., aortic surgery), and severe prolonged hypotension, among others.1 Most worrisome are situations in which multiple risk factors exist concurrently (e.g., when anemia, hypotension, epidural local anesthetic injection, and aortic occlusion occur simultaneously in a patient with occlusive vascular disease undergoing resection of an abdominal aortic aneurysm).
SCOPE
Ischemic injury to the neuraxis can occur spontaneously2,3 during surgery under general anesthesia4 and following neuraxial blocks.5 The incidence of ischemic injury to the spinal cord following spinal or epidural anesthesia is not precisely known, but it is rare. For example, Auroy et al.6 noted no ischemic complications following 41,079 spinal anesthetics and 35,293 epidural anesthetics voluntarily reported to the French SOS Regional Anesthesia Service over 10 months in 1998 and 1999. Nedeltchev et al.2 reviewed 57 cases of anterior spinal artery syndrome presenting to their institution (University Hospital of Bern, Switzerland) between 1990 and 2002 and reported 1 case associated with epidural anesthesia. These investigators made it clear that epidural anesthesia was not a proven cause but was chosen as the etiologic factor because no other clear etiology existed.
SPINAL CORD VASCULAR ANATOMY, PHYSIOLOGY, AND PHARMACOLOGY
Vascular Anatomy
Arterial System
The arterial supply to the spinal cord is both complex and variable (Fig. 12-1). Much of the variability relates to differences among individuals in terms of the exact sites of origin of vessels that perfuse the spinal cord.
FIGURE 12-1. Arterial supply to the spinal cord, transverse view. (Modified from Sliwa JA, Maclean IC. Ischemic myelopathy: a review of spinal vasculature and related clinical syndromes. Arch Phys Med Rehabil 1992;73:365–372, with permission. Ref. 7.)
In general, two posterior spinal arteries and a single anterior spinal artery supply the spinal cord. The posterior vessels originate from either the vertebral arteries or the posterior inferior cerebellar arteries. The anterior spinal artery originates from paired branches of the vertebral arteries that meet in the midline near where the vertebral arteries merge to form the basilar artery. The single anterior spinal artery supplies the anterior two-thirds of the spinal cord and the cauda equina. The posterior arteries supply the posterior third of the spinal cord but do not generally contribute to the cauda equina nerve roots. The fact that the single anterior spinal artery supplies two-thirds of the spinal cord likely accounts for the fact that the anterior spinal artery syndrome is far more common than the posterior spinal artery syndrome.
The anterior and posterior arteries connect via branches that encircle the spinal cord (vasa coronae), thereby providing a limited capacity for communication between the anterior and posterior circulations (Fig. 12-1). The limited capacity for communication between the anterior and posterior spinal circulations is demonstrated by the fact that ischemic syndromes in the anterior and posterior circulations are recognized as distinct clinical entities. The anterior, posterior, and vasa coronae arteries give rise to penetrating arterioles that supply the deeper layers of the spinal cord. There are no anastomoses between the penetrating arterioles, and thus the center of the cord represents a watershed area.
Blood flow through both the anterior and the posterior spinal arteries is augmented by segmental contributions from a variable number of “reinforcing” arteries (Fig. 12-2). The classification system used to describe these vessels varies throughout the literature, giving rise to some confusion about the underlying anatomy. For the purposes of this text, vessels arising from the aorta or its main branches and reaching the intervertebral foramen are termed segmental arteries. Those extending from the intervertebral foramen across the epidural space and along the nerve roots are termed spinal radicular arteries. The terminal portions of radicular arteries that reach the spinal cord are termed spinal medullary arteries.
FIGURE 12-2. Arterial supply to the spinal cord, longitudinal view. (Modified from Neal JM. Neurologic complications. In: Rathmell JP, Neal JM, Viscomi CM, eds. Regional Anesthesia: The Requisites in Anesthesiology. Philadelphia, PA: Elsevier-Mosby, 2004:157–163, with permission. Ref. 8.)
In the early embryo, there are bilateral segmental arteries associated with each spinal segment (62 total), but many of these degenerate during embryogenesis. The remaining segmental arteries vary greatly in number, size, and location among individuals. Those segmental arteries that persist take their origin from the closest artery adjacent to the spinal column (i.e., vertebral, costocervical trunk, thyrocervical trunk, intercostal branches of the aorta, lumbar arteries, iliolumbar arteries, and lateral sacral arteries).
The segmental arteries enter the spinal canal via the intervertebral foramina, at which point they become radicular branches. The radicular arteries divide into anterior and posterior branches that accompany the corresponding nerve roots (Fig. 12-1). Most of these radicular branches do not reach the spinal cord but terminate on the spinal nerve roots. The terminal branches of the few radicular arteries that do reach the spinal cord are termed spinal medullary arteries. Tureen9 reported that on average there are only 24 medullary arteries that reach the spinal cord, and most of these anastomose with the posterior spinal arteries. In fact, Mettler10 reported that the anterior spinal artery receives contributions from only between 4 and 10 medullary vessels.
The most important segmental artery is the arteria radicularis magna (artery of Adamkiewicz), which is responsible for the blood supply to 25% to 50% of the anterior spinal cord. The origin and course of the arteria radicularis magna is both variable and clinically important. Biglioli et al.11 have reported that the artery originates on the left 71% of the time and between L1 and L3 65% of the time. They also noted origins as high as T9 and as low as L5. In fact, origins as high as T5 have been reported. The arteria radicularis magna’s origin is clinically important because interruption of flow through this critical vessel (e.g., during aortic cross-clamping) puts the spinal cord at risk of ischemia.
In general, spinal cord segments cephalad of T2 and caudad of T8 are relatively highly vascularized, whereas the intermediate thoracic cord is less vascularized. Thus, some have suggested that the midthoracic cord is at greater risk of hypoperfusion. However, it is important to note that the midthoracic cord contains less gray matter and fewer neurons than the cervical and lumbar enlargements and therefore requires less blood flow. Thus, it is not clear that the midthoracic cord is physiologically at greater risk of reduced blood flow than are the more highly perfused regions.
Venous System
As in other organs, the venous drainage from the spinal cord is highly variable. In general, blood drains from the center of the cord centrifugally into an anterior central vein, a posterior central vein, and variously positioned peripheral veins lying on the surface of the cord. These venous channels drain into anterior and posterior radicular veins that follow the spinal nerve away from the spinal cord. Although radicular veins are more uniformly distributed than radicular arteries, they are not present at each spinal segment and their diameter is highly variable. If both anterior and posterior radicular veins are present at a given spinal cord segment, they unite to form a single radicular vein, which courses adjacent to the spinal nerve.
After traversing the dura mater, the radicular veins join the vertebral venous plexus (Batson’s plexus). This plexus lies primarily in the anterior lateral epidural space12 and runs the entire length of the spinal column. It also drains the vertebral bodies and the paraspinous muscles. In addition, the vertebral venous plexus has connections to systemic veins from the pelvis to the base of the brain.
Vascular Physiology
Technical barriers make studies of SCBF difficult in humans. However, animal models suggest that control of SCBF is qualitatively similar to the control of cerebral blood flow (CBF). For example, like the cerebral vasculature, blood flow in the spinal vasculature is autoregulated. That is, within defined limits of spinal cord perfusion pressure (SCPP) SCBF remains constant (Box 12-1). Using rhesus monkeys, Kobrine et al.13 demonstrated that SCBF was maintained constant between a mean arterial pressure (MAP) of 50 and 135 mm Hg. Above and below these limits, the flow was pressure passive. Similarly, Hickey et al.14 used a rat model to demonstrate that autoregulatory thresholds for the brain and spinal cord were identical (roughly 60–120 mm Hg). Further, they demonstrated that global CBF and global SCBF were identical (~60 mL/100 g/min) (Fig. 12-3).
BOX 12-1 Determinants of SCBF
SCBF is autoregulated in a way similar to cerebral blood flow.
Spinal cord perfusion pressure is determined by:
Mean arterial pressure
The greater of venous pressure or cerebrospinal fluid pressure
Spinal vasculature exhibits CO2 reactivity
SCBF exhibits flow-metabolism coupling
FIGURE 12-3. Simultaneous blood flow to the brain and spinal cord as a function of perfusion pressure (PP = MAP – ICP) in rats. For both brain and spinal cord, flow is essentially constant between a perfusion pressure of 60 and 120 mm Hg. (Redrawn from Hickey R, Albin MS, Bunegin L, et al. Autoregulation of spinal cord blood flow: is the cord a microcosm of the brain? Stroke 1986;17:1183–1189, with permission.)
Autoregulation has not been as well studied in humans, but both Abe et al.15 and Tsuji et al.16 measured human SCBF during PGE1-induced hypotension in humans undergoing spinal surgery. Both studies found that SCBF was maintained constant when MAP was decreased to 60 mm Hg. However, what is largely unknown is what constitutes the ischemic threshold in most humans. Using an electroencephalogram model in humans undergoing carotid endarterectomy, Sharbrough et al.17 saw no reduction in neurophysiologic function until CBF was reduced by 50% in these patients. In recent years, Drummond18 and others have questioned whether the commonly published 50 mm Hg lower limit of cerebral autoregulation (LLA) is in fact too low for a subset of humans, particularly those with underlying hypertension. This potentially meritorious theory admittedly has limited corroborating human outcome data, but has led some practitioners to rethink the lower limits of autoregulation in their patients. Thus, Drummond18 suggested in a 1997 letter-to-the-editor that the LLA for normotensive, unanesthetized adults may be closer to 65 mm Hg, while the point at which CBF reserve is exceeded might be 40% below baseline MAP. Because volatile and local anesthetics may themselves reduce the LLA because of their vasodilation and metabolic uncoupling effects (discussed below), it is possible that what we have previously considered the LLA in awake patients may more closely approximate the LLA in the anesthetized state. Further study is needed to improve our understanding of these critical relationships.
Importantly, SCPP is determined by the difference between the inflow pressure (normally MAP) and outflow resistance (the higher of venous pressure [VP] or cerebrospinal fluid pressure [CSFP]). That is, SCPP = MAP – CSFP (or VP). Thus, the spinal cord is put at risk of ischemia by systemic hypotension, occlusion of any arterial vessel(s) contributing to SCBF (e.g., aortic cross-clamp, atherosclerotic disease, traumatic vascular injury, epidural hematoma, and so on), increases in CSFP (e.g., epidural hematoma, epidural injection), occlusion of venous outflow (e.g., epidural hematoma, epidural injection), mechanical ventilation during certain pathologic states such as acute lung injury,19 and so on.
The impact of CSFP on SCBF deserves special attention because it has been shown to play a clinically significant role in SCPP during low-flow states. In particular, animal studies demonstrate that CSF drainage (i.e., reducing CSFP) significantly increases SCBF and reduces the risk of spinal cord ischemia during aortic cross-clamp.20,21 Similar studies in humans suggest the same.22 More important, epidural local anesthetic injections have been shown to produce a significant volume-related increase in CSFP.23–25 Although this increase in pressure is clearly well tolerated by most patients, it is reasonable to infer that injections into the epidural space during periods of reduced spinal cord perfusion (i.e., during aortic cross-clamp) may put patients at increased risk of spinal cord ischemia.
In addition to autoregulation, animal models demonstrate that the spinal vasculature (like the cerebral vasculature) has CO2 reactivity. Specifically, SCBF increases with increasing PaCO2 and decreases with decreasing PaCO2.26,27 Importantly, CO2 reactivity is preserved following spinal cord transection, indicating that CO2 reactivity is a local phenomenon and is not mediated by higher centers. Thus, the risk of spinal cord ischemia is increased in the presence of hyperventilation (hypocapnia), especially if there is a simultaneous decrease in SCPP.
Like the brain, the spinal cord displays flow-metabolism coupling. That is, increased or decreased metabolic activity results in a commensurate increase or decrease in SCBF. This was shown in a study by Sakamoto and Monafo,28 who found that decreasing metabolic activity by local cooling of the spinal cord resulted in a significant decrease in SCBF. Importantly, decreases in blood flow that result from decreased metabolic activity do not put the spinal cord at risk of ischemia because the flow is exactly matched to the metabolic needs of the tissue. It is crucial to keep this fact in mind when interpreting the effects of local anesthetics on SCBF.
Pharmacology
Identifying the effects of spinally administered drugs on SCBF is essential for understanding the ischemia-related risks of neuraxial blocks. In particular, it is necessary to know whether intrathecally administered drugs have direct effects on SCBF. In this respect, the scientific literature is somewhat wanting. The principal difficulty in interpreting the available data is that investigators often failed to control for, or to account for, the effects of changes in MAP, PaCO2, temperature, drug-mediated decreases in spinal cord metabolic rate (either the study drug or the concomitant general anesthetic), drug-mediated effects on autoregulation, and so on. In the absence of appropriate controls, it is often difficult to determine whether the observed effect on SCBF is a direct effect of the local anesthetic or the result of effects on MAP, metabolic rate, and so on.
Local Anesthetics
Intrathecal
Studies of the effects of local anesthetics on SCBF have produced all possible results (i.e., increased flow, decreased flow, and no change in flow). The variability of the results may reflect differences in the local anesthetic employed, the animal model studied, the method used to measure blood flow, the concomitant general anesthetic administered, whether MAP was maintained in the autoregulatory range, and so on.29,30
Kozody et al.31 used radioactive microspheres to measure the effect of intrathecal bupivacaine (20 mg) on SCBF in pentobarbitone-anesthetized mechanically ventilated dogs. They found that bupivacaine alone produced an approximately 37% decrease in SCBF and an approximately 30% decrease in MAP.
These data may be interpreted in several ways. For example, the decrease in flow could be explained by a local anesthetic–mediated loss of SCBF autoregulatory mechanisms, which in the face of the decreased MAP (i.e., decreased SCPP) would result in a decrease in SCBF comparable to the MAP decrease. However, there are data suggesting that autoregulatory mechanisms are intact during spinal anesthesia. For example, Dohi et al.32 found that tetracaine spinal anesthesia alone did not decrease SCBF despite a significant decrease in MAP from 110 to 89 mm Hg. However, when animals were hemorrhaged to a MAP of 66 mm Hg, SCBF decreased significantly. These data are consistent with the hypothesis that autoregulation was intact and that SCBF decreased only when MAP was decreased below the autoregulatory threshold.
An alternative explanation is that local anesthetic–mediated blockade of neuronal activity may have decreased neuronal metabolic rate, resulting in a commensurate decrease in blood flow because of flow-metabolism coupling. Consistent with the latter explanation is a study by Kuroda et al.,33 who measured the metabolic rate for glucose in the spinal cord of rats before and after epidural bupivacaine block. They reported that epidural bupivacaine block produced an 18% to 29% decrease in glucose metabolism. Similarly, Crosby34 has shown that intrathecal bupivacaine reduces glucose metabolism by 15% to 21% in superficial regions of the spinal cord. Using a different approach to studying local anesthetic effects on spinal cord metabolic activity, both Cole et al.35 and Breckwoldt et al.36 have shown that intrathecal tetracaine markedly increases the spinal cord’s ability to tolerate ischemia, presumably because of decreased metabolic rate.
Although they do not offer definitive proof, these studies suggest that the decrease in SCBF observed by Kozody following intrathecal bupivacaine may be the result of a decrease in spinal cord metabolic rate, and not a loss of autoregulatory mechanisms. Studies of tetracaine’s effects on SCBF are variable. Using a pentobarbitone-anesthetized mechanically ventilated dog model, Kozody et al.37 reported that tetracaine (20 mg) produced an approximately 140% increase in SCBF. The mechanism(s) responsible for the increase in blood flow is unclear, but tetracaine-mediated disruption of flow-metabolism coupling is one possibility. Loss of autoregulation is an unlikely explanation because spinal block was not associated with an increase in MAP.
In contrast, using a halothane-anesthetized mechanically ventilated dog model, Dohi et al.32 found that 5 mg of intrathecal tetracaine had no effect on SCBF. Similarly, Porter et al.38 used a pentobarbitone-anesthetized cat model to demonstrate that 5 mg intrathecal tetracaine had no effect on SCBF.
It is tempting to ascribe the differences in the effect of intrathecal tetracaine on SCBF solely to differences in the tetracaine doses used in these studies (Kozody et al., 20 mg; Dohi et al. and Porter et al., 5 mg). However, in the absence of a clear study of dose-related effects of intrathecal tetracaine on SCBF, it is difficult to unquestioningly accept this conclusion.
Dohi et al.,39 using an anesthetized dog model, performed a dose-ranging study of intrathecal lidocaine’s effect on SCBF. These investigators found that 10, 20, 30, or 50 mg of plain lidocaine did not have any statistically significant effect on SCBF despite significant decreases in MAP at all doses. Similarly, using a pentobarbitone-anesthetized cat model, Porter et al.38 found that neither intrathecal lidocaine (15 mg) nor mepivacaine (10 mg) had any effect on SCBF despite a significant decrease in MAP.
Epidural
Studies of the effects of epidural local anesthetics are fewer in number than studies of intrathecal local anesthetics. Mitchell et al.40 used an opioid-anesthetized dog model to study the effects of 100 mg of lumbar epidural lidocaine (5 mL, 2%) on SCBF. They found that blood flow was reduced in all regions of the spinal cord by 20% to 40% and that the magnitude of the reduction was generally greater the closer the measurement was made to the site of local anesthetic injection. The reduction in SCBF occurred despite the fact that MAP was maintained at baseline by infusion of lactated Ringer’s solution. In addition, they used evoked potentials to document that spinal cord neuronal activity was reduced by epidural blockade, a finding that suggests that the reduction in SCBF was in response to a reduction in spinal cord metabolic activity.
Bouaziz et al.41 used pentobarbitone-anesthetized rabbits to investigate the effects of 20 mg epidural lidocaine (1 mL; 2%) in young and adult animals. They found that lumbar SCBF was not altered in adult animals despite a significant decrease in MAP. Conversely, in young animals, lumbar SCBF decreased significantly and the decrease was correlated with the decrease in MAP. These findings suggest that adult animals maintained normal autoregulation but that autoregulation was impaired in immature animals. Whether there are similar age-related differences in humans is unknown.
Vasoactive Adjuncts: Intrathecal
Phenylephrine and epinephrine are sometimes added to local anesthetics to increase the duration and/or to improve the quality of spinal and epidural blockade. The conventional wisdom has been that these drugs exert their salutary effects on block duration by decreasing local anesthetic clearance by way of alpha1-adrenergic receptor-mediated vasoconstriction. The problem with this simplistic view is that it fails to explain why the dose of phenylephrine required to prolong spinal blockade is approximately 10 to 20 times greater than the dose of epinephrine, even though both drugs are approximately equipotent as alpha1-adrenergic agonists. One reasonable answer to this question is that the block-prolonging effects of epinephrine and phenylephrine are not mediated by vasoconstriction.
Consistent with the idea that spinal cord vasoconstriction is not the mechanism by which epinephrine and phenylephrine prolong spinal anesthesia is the observation by Kozody et al.42 (using a radioactive microsphere technique) that neither epinephrine (200 μg) nor phenylephrine (5 mg) had any effect on SCBF in pentobarbitone-anesthetized dogs. Dohi et al.32 also reported that intrathecal epinephrine (100, 300, and 500 μg) had no effect on SCBF in halothane-anesthetized dogs using a hydrogen clearance technique to measure blood flow.
In contrast to the results of Kozody et al., Dohi et al.39 reported a dose-dependent decrease (25%–45%) in SCBF following intrathecal phenylephrine doses of 2, 3, and 5 mg. A 1-mg phenylephrine dose produced a statistically insignificant decrease in SCBF. Why the effects of phenylephrine reported by Kozody and Dohi are so divergent is unclear. However, it is worth noting that Dohi et al. used the hydrogen clearance technique to measure blood flow and that Kozody used the “gold-standard” microsphere technique. The hydrogen clearance technique requires the insertion of a hydrogen-sensitive electrode into the spinal cord, and electrode insertion itself has been shown to decrease blood flow.43
Iida et al.44 attempted to determine the effects of vasoactive drugs on spinal cord circulation by applying a variety of drugs directly to pial vessels on the surface of the spinal cord and measuring the change in vessel diameter. They found that topical application of epinephrine (5 μg/mL) and phenylephrine (5 μg/mL) produced a modest reduction in the diameter of pial vessels (~8%–11%). These investigators did not measure resultant SCBF, but previous studies have shown that the diameter of superficial pial arterioles does not correlate well with blood flow in the underlying tissue.45
Thus, with respect to the direct effect of epinephrine and phenylephrine on SCBF, the available data would suggest that intrathecal epinephrine does not have a significant effect on SCBF.46 The data are not as clear for the effects of phenylephrine, but the best data (Kozody et al. and Iida et al.) would suggest that phenylephrine probably does not have a significant effect either. This begs the question as to how these drugs prolong and improve local anesthetic block if they do not decrease SCBF. One possibility is that a vascular site other than the spinal cord is the vasoconstrictor effect site. In particular, the dura mater is possibly the site, as it has an abundant vascular supply. In fact, Kozody et al. showed that although intrathecal epinephrine and phenylephrine did not affect SCBF, they did significantly reduce dural blood flow. Another possibility is that the alpha2-adrenergic receptor effects of epinephrine and phenylephrine are responsible for their ability to improve local anesthetic block. That is, these drugs may prolong the block by inhibiting nociceptive transmission just as the alpha2-agonist clonidine does. An attractive aspect of this hypothesis is that it would explain why the required dose of phenylephrine is so much greater than that of epinephrine. Specifically, because phenylephrine is a very weak alpha2 agonist at usual clinical doses, a supraphysiologic dose is necessary to produce a measurable alpha2-mediated block-prolonging effect (Box 12-2).
BOX 12-2 Mechanisms by Which Vasoconstrictors Prolong Neuraxial Local Anesthetic Block
Epinephrine and probably phenylephrine do not decrease SCBF.
Epinephrine and phenylephrine significantly decrease dural blood flow, which reduces the clearance of local anesthetics.
Epinephrine and (to a lesser extent) phenylephrine provide direct analgesia as agonists of alpha2-adrenergic receptors in the spinal cord.

Full access? Get Clinical Tree
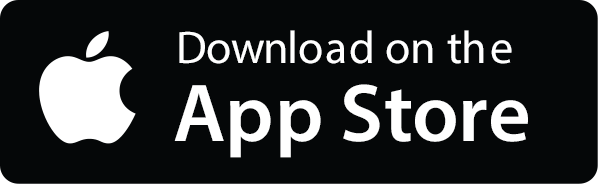
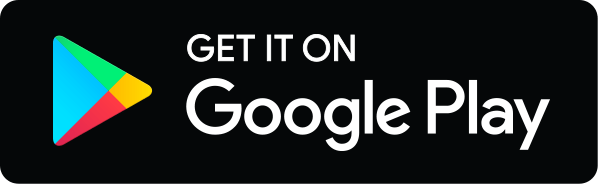