Neural Blockade for Abdominal and Thoracic (Non-vascular) Surgery
Francisco Asenjo
Francesco Carli
Regional Anesthesia for Thoracic (Noncardiac) Surgery
Thoracic surgery has benefited greatly from advances in anesthesia. Sixty years ago, intubation allowed control of the airway and ventilation. Then, the ability to separate the lungs with one-lung ventilation techniques provided better surgical conditions. Lately, regional anesthesia and analgesia have decreased morbidity and improved outcome in the perioperative period (see Chapters 6 and 7).
Neuroanatomy of the Chest and Abdomen
The somatic innervation of the chest and abdominal wall is supplied by the intercostal nerves from T2 to T12. After exiting through the intervertebral foramina, the roots divide into ventral branches, dorsal branches, and sympathetic nerves. The dorsal branches run posteriorly to innervate the dorsal paravertebral muscles as well as the skin from midline to 8 to 10 cm laterally. The ventral branches progress antero-laterally under the ribs accompanied by the intercostal artery and vein to innervate the chest wall and breast. This course of the nerves explains the inconsistency between the more superior segmental innervation found in the posterior paravertebral cutaneous innervation and the lower anterior distribution. The ventral branches give origin to two or three perforating cutaneous nerves that divide to innervate the skin from 8 to 10 cm lateral of the dorsal midline to the ventral midline. The primary sensory nerves conveying pain from skin, ribs, and soft tissues are Aδ- and C-type neurons (see Figs. 16-1 and 16-4).
The shoulder girdle is innervated by the brachial plexus. The brachial plexus supplies the motor nerve component of the chest wall muscles that stabilize the shoulder girdle (i.e., pectoralis, latissimus dorsi). The sensation to the skin below the clavicle is provided by cutaneous branches of the cervical superficial plexus (supraclavicular nerve) (see Chapter 13).
The autonomic nervous system supplies the innervation of the viscera, both in the chest and abdomen. In the chest, sympathetic nerves from T1 to T5 travel bilaterally to the heart, carrying efferent nerves that contribute to increase contractility and frequency of the heartbeat as well as to regulate the coronary circulation. In healthy coronaries, the activation of these nerves produces coronary dilatation; however, the contrary occurs in patients with coronary artery disease (CAD). Afferent sensory nerves convey ischemic pain from the heart. Other sympathetic fibers carry the afferent and efferent innervation of the bronchi, pericardium, and other thoracic visceral anatomic structures. Sympathetic nerves from T4–T6 to T10 bilaterally form the greater, lesser, and lowest splanchnic nerves that course adjacent to the spine. These fibers cross under the diaphragmatic crura to the abdominal cavity at the level of T12 or L1 to merge as a group of ganglia in front of the celiac trunk to form the celiac plexus. Fibers from the vagus nerve also course to the abdomen along the esophagus. Similar ganglia are present around the origin of the superior mesenteric artery coming from a lower origin in the spine. These ganglia contain the soma of the postganglionic sympathetic neurons providing vascular tone to the abdominal vascular structures, as well as regulation of hormonal and metabolic functions of the viscera (i.e., catecholamine release from the adrenal glands, renin-angiotensin-kallikrein system). Through these ganglia pass the preganglionic parasympathetic neurons that connect with their terminal synapses in the viscera that they are traveling to. The ganglia also include sensory afferent fibers that carry pain signals from the abdominal viscera and peritoneum. Another important visceral sensory structure is the superior hypogastric plexus located in front of the sacral promontorium. This plexus conveys the afferent and efferent innervation (sympathetic/parasympathetic) of the pelvic visceral structures (see Chapter 39, Figs. 39-1,39-2,39-3,39-4,39-5,39-6,39-7,39-8,39-9,39-10 and Figs. 39-21,39-22,39-23). The phrenic nerves originate bilaterally from C3–C4/5 and give the motor activity to the diaphragm. However, it has been recognized that the phrenic nerves also provide sensory visceral innervation to the diaphragm from both the thoracic as well as the abdominal directions. In fact, because the phrenic nerves share dermatomes with the somatic innervation of the shoulder, patients who have had intrathoracic procedures frequently complaint about ipsilateral shoulder pain in spite of excellent incisional and chest tube area analgesia. However, this pain can also result from other sources including myocardial ischemia, a chest drain impinging on the apex of the pleura and the brachial plexus, and malposition or compression of the shoulder during the surgery (see also Chapter 45).
The vagus nerve supplies parasympathetic innervation to the mediastinal and upper abdominal viscera, including the stomach, liver, kidneys, small bowel, and the ascending and proximal transverse colon (see Figs. 39-5 and 39-21).
The cervical superficial plexus (C3–C4) innervates the skin, fascia, and most of the musculature of the neck. Consideration should be given to this innervation when esophageal surgery is planned with a neck (as well as thoracic) incision (1).
Physiologic Considerations of Thoracic Epidural Blockade
Cardiovascular Effects
Cardiovascular changes occur when local anesthetics are injected into the thoracic epidural space. The extension and intensity of the changes depend upon the volume/mass of local anesthetic injected, the age of the patient, and the spinal level at which the anesthetic agents are deposited. These factors affect the impact on the sympathetic nervous system produced by the local anesthetics. The nerve roots in the intervertebral foramina contain afferent sympathetic fibers, as well as efferent fibers regulating the “tone” of the vascular structures they innervate. These are small neurons that are easily blocked by small concentrations of local anesthetics, leaving the arteries and veins to regulate their caliber in a “low-resistance state.” Therefore, the blood flow toward the regions affected by the decrease in vascular sympathetic tone increases (abdomen). Pooling of venous blood decreases central venous pressure, cardiac output, and subsequently, blood pressure (2). A compensatory vasoconstriction in the vasculature of the unblocked regions may attenuate to certain extent the drop in blood pressure and increase cardiac output in healthy individuals. Thoracic epidural anesthesia leads to compensatory reduction of myocardial work and oxygen demand. In patients with CAD, thoracic epidural anesthesia improves cardiac function through an effect on diastolic function and near normalization in the coronary blood flow (3). As shown by echocardiographic testing, a reduction in mean arterial pressure leads to myocardial wall motion dysfunction only during lumbar epidural anesthesia, not during thoracic epidural anesthesia (4) (see Chapter 11).
The visceral sympathetic innervation reaching the suprarenal gland will also be blocked. As a consequence, the systemic circulation of catecholamines released by the adrenal gland will decrease, thus compounding the drop in peripheral resistance, venous return, cardiac output, and blood pressure.
If the epidural blockade reaches the cardiac sympathetic fibers (T1–T5), in addition to the vasodilatation and systemic catecholamine decrease just mentioned, a drop in chronotropism and inotropism is observed in healthy people. Hypotension can be profound in patients with poor cardiovascular reserve and requires prompt action to correct. Thoracic epidural anesthesia is associated with a significant reduction in the arrhythmias associated with cardiac surgery and thoracic surgery, but not in esophageal cancer resection (5,6,7,8).
Respiratory Effects
Epidural blockade in the thoracic spine from T1 to T5 is related to minor physiologic respiratory changes (5% decreases in vital capacity [VC] and forced expiratory volume [FEV] 1.0; 0.16 kPa increases in PaCO2) in healthy patients. Shunt fraction and alveolar-arterial differences in PaO2 do not change with thoracic epidural anesthesia. To affect the mechanical function of the intercostal muscles, a thoracic epidural blockade would require a highly concentrated local anesthetic covering segments from T2 to T8. Motor blockade is about four to five dermatomes below the sensory level. Epidural blockade with mepivacaine 2% from dermatomes C4 to T7 and from T5 to L4 leads to a decrease of 25% in VC and of 13% in FEV1 (9). In chronic obstructive pulmonary disease (COPD) patients, epidural block with bupivacaine 0.5% (sensory block from C4 to T8) leads to a decrease of only 8% from baseline in VC and FEV1. With extensive epidural block, the sitting position is poorly tolerated. Supine position is often associated with decreases in VC and FEV1, up to 23%. On the other hand, hypotension and hypoperfusion of the respiratory centers in the brainstem from thoracic blockade can produce severe changes in the respiratory physiology (see Chapter 11).
During the postoperative period Whaba (10) and Mankikian (11) have shown improvements in functional residual capacity (FRC) and VC with the use of thoracic epidural local anesthetics. Mankikian also demonstrated that postoperative thoracic epidural block corrects postoperative diaphragmatic dysfunction. In patients with COPD or increased bronchial reactivity, high thoracic epidural block does not disrupt mechanical and functional respiratory patterns more than in healthy patients. The unopposed bronchial reactivity due to the sympathetic blockade might be attenuated by the systemic effect of the local anesthetics (see Chapter 11).
Regional Anesthesia Techniques for Thoracotomy
Thoracotomy is associated with atelectasis, intrapulmonary shunt, and ventilation–perfusion mismatch. General anesthesia, postoperative immobilization, and systemic administration of opioids and sedative drugs adversely affect ventilation and gas exchange and cause respiratory complications. Open lung surgery is also a cause for intra- and postoperative arrhythmias. An ideal anesthesia and analgesia technique for thoracotomy should be rapid in onset, facilitate recovery, and should provide optimum analgesia for a minimum of 3 postoperative days (as long as chest drains are in situ). Patients should be able to move, take deep breaths, produce effective coughs, and cooperate with physiotherapy. Dietary intake and bowel function should be restored soon after surgery. The most common regional anesthesia techniques for thoracic surgical procedures involving the opening of the chest include thoracic epidural, paravertebral, intercostal, and interpleural blockade.
Thoracic Epidural Blockade
Thoracic epidural anesthesia is achieved by approaching the epidural space with a paramedian or mid-line technique. Thoracic epidural anesthesia has been used for lung, chest wall, esophageal, and plastic/reconstructive surgeries (see Chapter 11).
Epidural local anesthetics increase PaO2 and decrease the incidence of pulmonary infections and pulmonary complications overall. Gruber and co-workers evaluated the effects of thoracic epidural anesthesia in patients with end-stage COPD (12) and showed better ventilatory mechanics with no changes in either gas exchange or inspiratory muscle force. Effectiveness of the sensory block should be established before induction of general anesthesia with either cold or pin-prick testing and by autonomic response such as a decrease in blood pressure or pupillary reflex dilation in response to noxious stimulation (13). Intraoperative thoracic epidural blockade has been shown to decrease chronic pain after thoracotomy (14,15). One-lung ventilation causes significant increases in shunt fraction associated with a decrease in PaO2. However, PaO2 remains significantly increased in patients with thoracic epidural anesthesia and general anesthesia compared with total intravenous anesthesia (TIVA) alone (16). Thoracic epidural anesthesia in combination with general anesthesia does not impair arterial oxygenation to the same extent as TIVA, which
might be a result of the changes in cardiac output. It is suggested that patients with cardiopulmonary disease and impaired oxygenation before one-lung ventilation might benefit from thoracic epidural anesthesia combined with general anesthesia. In a meta-analysis of 65 studies, Ballantyne and associates concluded that postoperative epidural pain control may significantly decrease pulmonary morbidity (17). Commonly used opioid–local anesthetic mixtures include fentanyl-bupivacaine, morphine-bupivacaine, and fentanyl-ropivacaine. Very-low-dose ketamine (approximately 3 mg/hr for 72 hours) potentiates morphine–ropivacaine analgesia and reduces postthoracotomy pain (18) (see Chapter 11).
might be a result of the changes in cardiac output. It is suggested that patients with cardiopulmonary disease and impaired oxygenation before one-lung ventilation might benefit from thoracic epidural anesthesia combined with general anesthesia. In a meta-analysis of 65 studies, Ballantyne and associates concluded that postoperative epidural pain control may significantly decrease pulmonary morbidity (17). Commonly used opioid–local anesthetic mixtures include fentanyl-bupivacaine, morphine-bupivacaine, and fentanyl-ropivacaine. Very-low-dose ketamine (approximately 3 mg/hr for 72 hours) potentiates morphine–ropivacaine analgesia and reduces postthoracotomy pain (18) (see Chapter 11).
The Direct Search Procedure technique has been utilized to find proper doses, infusion rates, and combinations of drugs to achieve optimal analgesia with thoracic epidural anesthesia (19,20). A sophisticated mathematical model tested multiple solutions and infusion rates based on bupivacaine, fentanyl, and clonidine. The three best combinations of bupivacaine dose (mg/h), fentanyl dose (μg/h), clonidine dose (μg/h), and infusion rate (mL/h) were: 9-21-5-7, 8-30-0-9, and 13-25-0-9. Mixing an opioid (fentanyl 2–3 μg/mL or sufentanil 0.5–1 μg/mL) with a local anesthetic improves pain control with thoracic epidural anesthesia; side effects may increase, decrease, or remain unchanged. Adding epinephrine to mixtures of opioids with low-concentration solutions of local anesthetics has proven beneficial for thoracic epidural analgesia (20), but the role of clonidine remains undetermined.
A recent well-conducted study of analgesia after thoracotomy showed a minor difference between intercostal block and IV patient-controlled analgesia (PCA) versus epidural analgesia with local anesthetics and fentanyl on pain and lung function (21) (see Chapter 11).
Level of Catheter Insertion
Most robust evidence for the analgesic and functional benefits provided by thoracic epidural anesthesia comes from studies using local anesthetics with or without opioids (2,22,23,24). Epidural catheters placed in the lumbar area for thoracic/upper abdominal surgery require hydrophilic opioids to produce analgesic effects in the thoracic region. However, no clear functional benefits have been documented with this approach. To achieve maximal analgesia, the epidural catheter should be placed in the dermatome corresponding to the mid-point of the surgical incision. Therefore, it is recommended that the catheter be inserted at the T4–T6 level for thoracic surgery. Epidural catheters placed in the lower thoracic spine do not provide adequate analgesia to incisions in the T4– 6 region during thoracic surgery. Increasing the infusion rate to improve analgesia produces unwanted motor blockade in the lower extremities and impairs mobilization. Likewise, catheters inserted above T4 tend not to cover the insertion site of the chest drains, producing only partial analgesia. Blocking the upper thoracic dermatomes is usually accompanied by hypotension secondary to cardiac sympathetic blockade, decrease in cardiac output, and blockade of the sympathetic innervation of the suprarenal glands. This is more noticeable when patients sit and ambulate after surgery. Compensatory sympathetic activity and vasoconstriction occur in the nonblocked distal territories (25,26). Moderate intravascular volume load and cardiostimulant drugs are recommended to reestablish cardiac output and blood pressure in this scenario. Up to 50% of the patients faint when placed in the sitting position for epidural catheterization (27). Sedation and glycopyrrolate or atropine before the procedure may decrease this side effect.
Assessment of Inadequate Analgesia
Postoperative analgesia may be associated with primary failure (inadequate analgesia immediately postoperatively) or secondary failure (initial adequate analgesia followed by an increase in pain/inadequate analgesia). Techniques to determine the etiology of epidural failure are discussed in Table 23-1.
Duration of Epidural Catheterization
Recommended practice is to remove thoracic epidural catheters 48 to 72 hours after chest operations or once the chest drains have been removed. An exception is the esophagectomy, in which some teams remove the epidural catheter by the fourth or fifth day after the barium test has been performed (28).
Treatment of Thoracic Epidural Anesthesia–induced Hypotension
Holte and co-workers assessed plasma volume before and 90 minutes after thoracic epidural anesthesia in 12 volunteers (29). They then administered 7 mL/kg hydroxyethyl starch or 0.2 mg/kg ephedrine. The authors concluded that epidural anesthesia does not lead to any changes in intravascular volume and, as both ephedrine and fluids have comparable hemodynamic effects, vasopressors might be preferred in the treatment of hypotension associated with epidural anesthesia (in patients who are well hydrated), especially for patients with cardiopulmonary diseases in which perioperative fluid overload is undesirable (see also Chapter 11).
Paravertebral Blockade
Paravertebral analgesia for thoracotomy can be provided by the surgeon once the chest is open or percutaneously by the anesthesiologist; both approach have similar success rate. The technique has been described under several different terms: paravertebral, continuous intercostal nerve block, extrapleural intercostal nerve block, extrapleural paravertebral, and retropleural analgesia (see Chapter 16, Figs. 16-15,16-16,16-17,16-18).
Typically, paravertebral catheters are located posterior to the parietal pleura in close proximity to the paravertebral pleural recess, where they provide analgesia by putting the local anesthetic in contact with the intercostal nerves close to their exit from the spine. This anatomic position explains why, in most cases, unilateral analgesia occurs in the affected side of the chest, but in a variable percentage of the patients, it migrates in part to the epidural space via the intervertebral foramen to produce a partial epidural block as well. The analgesic action may also be attributed to the local anesthetic’s action on the nerve roots or intercostal nerves and in part to a blockade of the sympathetic chain (31). Different local anesthetics have been successfully used (bupivacaine 0.25%, 0.5%; ropivacaine 0.5%; lidocaine 1%, 2%). A bolus of lidocaine 0.25 mL/kg provides excellent hemodynamic stability (32), as well as bupivacaine-fentanyl and ropivacaine-fentanyl mixtures (33) at 0.1 mL/kg/hr. Clonidine, when used as an adjunct to bupivacaine for continuous paravertebral block, improves analgesic efficacy. Hypotension occurs more frequently among patients receiving clonidine, but this responds well to treatment with volume (34). Sedation is the major adverse effect of clonidine that interferes with its clinical application.
A number of publications support a superior effect of paravertebral versus systemic opiates associated with the lower use of rescue analgesics and respiratory complication rates (35). FEV1 is also better preserved with paravertebral anesthesia when compared to systemic opiate administration. Randomized trials have compared paravertebral versus epidural anesthesia for thoracotomy in a recent meta-analysis (36) that included a total of 520 patients from 10 trials. Patients who received paravertebral anesthesia had a quality of analgesia at 4, 8, 24, and 48 postoperative hours similar to that of thoracic epidural anesthesia; however, patients with paravertebral anesthesia had better pulmonary function, lower rates of
pulmonary complications, less morphine consumption, and lower incidences of postoperative nausea and vomiting (PONV), urinary retention, and hypotension (36). Interestingly, the failure rate with paravertebral seems to be lower than with epidural anesthesia (37). The concept that epidural anesthesia is the gold standard for postoperative management of pain after thoracic surgery has been challenged by these publications supporting the paravertebral technique. Plasma levels of bupivacaine are between 3 and 4 μg/mL following a few days of infusion. For ropivacaine 0.2% at rates of infusion of 6 to 10 mL/hr, plasma ropivacaine concentrations remain below the toxic threshold. Paravertebral and multimodal analgesia have been also compared with PCA plus multimodal analgesia. Dynamic and static pain scores were lower in the paravertebral group. Side effects (nausea, vomiting, urinary retention) were less frequent in the paravertebral group (30% versus 75%; p <0.005) (35) (see also Chapter 16).
pulmonary complications, less morphine consumption, and lower incidences of postoperative nausea and vomiting (PONV), urinary retention, and hypotension (36). Interestingly, the failure rate with paravertebral seems to be lower than with epidural anesthesia (37). The concept that epidural anesthesia is the gold standard for postoperative management of pain after thoracic surgery has been challenged by these publications supporting the paravertebral technique. Plasma levels of bupivacaine are between 3 and 4 μg/mL following a few days of infusion. For ropivacaine 0.2% at rates of infusion of 6 to 10 mL/hr, plasma ropivacaine concentrations remain below the toxic threshold. Paravertebral and multimodal analgesia have been also compared with PCA plus multimodal analgesia. Dynamic and static pain scores were lower in the paravertebral group. Side effects (nausea, vomiting, urinary retention) were less frequent in the paravertebral group (30% versus 75%; p <0.005) (35) (see also Chapter 16).
Table 23-1 Most common causes of epidural failure/problems and correction | ||||||||||||||||||||||||||||||||||||||||
---|---|---|---|---|---|---|---|---|---|---|---|---|---|---|---|---|---|---|---|---|---|---|---|---|---|---|---|---|---|---|---|---|---|---|---|---|---|---|---|---|
|
Intercostal Blockade
Intercostal blockade (single-injection or continuous) was more popular for thoracotomy before thoracic epidural anesthesia became widespread. The injections can be done either under direct view by the surgeon from inside the chest or percutaneously. Single-injection intercostal nerve blocks for thoracotomy provide better pain relief than opioids alone, with
less total opioid requirement, and they improve briefly the FEV1 (37a). In a large study, an incidence of pneumothorax of 0.07% was reported, and peak plasma levels were reached at 10 minutes (bupivacaine 0.19–1.46 μg/mL) (38,39,40) with no signs of toxicity. Intercostal nerve blockade can be considered a reasonable alternative to paravertebral and thoracic epidural anesthesia (see Chapter 16 and Figs. 16-4,16-5,16-6,16-7,16-8,16-9,16-10,16-11,16-12).
less total opioid requirement, and they improve briefly the FEV1 (37a). In a large study, an incidence of pneumothorax of 0.07% was reported, and peak plasma levels were reached at 10 minutes (bupivacaine 0.19–1.46 μg/mL) (38,39,40) with no signs of toxicity. Intercostal nerve blockade can be considered a reasonable alternative to paravertebral and thoracic epidural anesthesia (see Chapter 16 and Figs. 16-4,16-5,16-6,16-7,16-8,16-9,16-10,16-11,16-12).

Full access? Get Clinical Tree
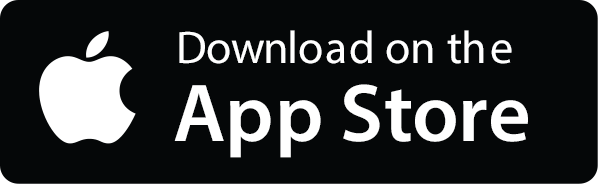
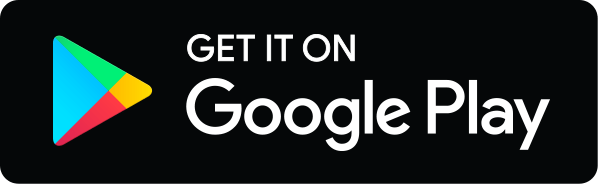
