Paul A. StrickerAlan Jay Schwartz
I. Physiology
A. Cardiovascular System
Normal Fetal to Pediatric Cardiovascular Transition
Development of normal cardiovascular physiology in the pediatric patient depends on the transition from fetal circulation to an adult flow pattern (1,2). The fetus uses the low vascular resistance placenta as the organ of respiration and therefore does not require pulmonary blood flow. Placental venous blood streams past the liver through the ductus venosus to provide venous inflow to the right atrium and is shunted across the foramen ovale and ductus arteriosus into the left heart and aorta bypassing the right heart flow and the pulmonary circuit (Fig. 33-1).
FIGURE 33-1 Fetal circulation showing direction of blood from the placenta (umbilical artery) that allows blood to bypass the fetal lungs via the foramen ovale, ductus arteriosus, and ductus venosus.
During the birthing process, elimination of the low resistance placental circulatory bed results in a rise in the neonate’s systemic vascular resistance. This is coupled with the decrease in neonatal pulmonary vascular resistance. This reduces and eventually eliminates the blood flow that had been directed away from the lungs through the foramen ovale and ductus arteriosus. The rise in arterial oxygen level when the neonate initiates breathing is essential to maintain blood flow through the alveolar vascular bed.
Although the pulmonary vascular resistance declines at birth, it is not at the normal adult level until the end of the neonatal period. Any factor that can cause a rise in the pulmonary vascular resistance (e.g., hypoxia, hypothermia, respiratory or metabolic acidosis) can precipitate a reversion to a fetal circulatory pattern, with reopening of the foramen ovale and ductus arteriosus shunting blood away from the neonate’s lungs. There are other differences in cardiac function that distinguish the pediatric heart from the adult heart. Most notable is the fact that the young child has a relatively noncompliant heart that depends on rate rather than contractility to boost cardiac output.
Common Congenital Cardiac Malformations
Malformations of the cardiac anatomy include many variations in which the ventricular and atrial chambers and the cardiac valves are deformed, causing abnormal blood flow patterns. It is quite difficult to remember all of the possible anatomic variations that comprise congenital heart disease. Viewing congenital heart disease as a physiologic assessment enables the clinician to group the various lesions into three general categories: lesions that cause obstruction to blood flow without shunting, lesions that result in an increase in pulmonary blood flow through a shunt pathway, and lesions that result in a decrease in pulmonary blood flow through a shunt pathway.
Congenital aortic stenosis and coarctation of the aorta represent examples of nonshunt-obstructing congenital cardiac defects (Fig. 33-2A,B). The major physiologic impairment is an increase in myocardial workload. Congenital aortic stenosis can be associated with rapid cardiac arrest when the stenotic valve is so narrow that the left ventricle fails to generate sufficient forward cardiac output to supply oxygen to the coronary circulation. A critical difference from adult aortic stenosis is that the pediatric heart does not have sufficient time to adapt and hypertrophy to compensate and overcome the valvular obstruction.
FIGURE 33-2 A: Aortic stenosis. B: Coarctation of the aorta also can lead to intracardiac shunting. Depending on the location of coarctation in relation to a patent ductus arteriosus, intracardiac shunting can be either right to left (preductal) or left to right (postductal). C: Ventricular septal defect leads to intracardiac shunting. Direction (right to left or left to right) depends on associated cardiac anatomy. D: Atrial septal defect leads to intracardiac shunting. Direction (right to left or left to right) depends on associated cardiac anatomy. E: Tetralogy of Fallot (TOF) consists of four anatomical abnormalities: (1) ventricular septal defect, (2) right ventricular outflow tract obstruction, (3) overriding aorta, and (4) left ventricular hypertrophy. (If an atrioventricular defect is present, the malformation is termed a pentalogy of Fallot.) TOF lesion leads to a right to left intracardiac shunt. ASD, atrial septal defect; VSD, ventriculoseptal defect; RA, right atrium; RV, right ventricle; PA, pulmonary artery; LA, left atrium; LV, left ventricle; Ao, aorta.
Ventricular septal defect (VSD) is the most common congenital cardiac lesion (Fig. 33-2C). It results in shunting of blood from the higher pressure left ventricle to the lower pressure right ventricle. As long as the shunt communication is sufficiently large to allow flow through it and the pulmonary vascular resistance is sufficiently low to allow flow from the right ventricle to the pulmonary vascular bed, lesions like VSD will increase pulmonary blood flow. The overall shunt is from left to right. However, at any time during the cardiac cycle, the flow may cease or become right to left, highlighting the distinct possibility for paradoxical embolization from the venous to arterial circulation. When an atrial septal defect (Fig. 33-2D) (another example of a lesion that increases pulmonary blood flow) is present, paradoxical embolization to the cerebral circulation that causes a stroke in adult life may be the first diagnostic clue of the presence of the intracardiac communication.
Tetralogy of Fallot (TOF) (Fig. 33-2E) is an example of those congenital cardiac abnormalities that result in a decrease in pulmonary blood flow. The obstruction to normal blood flow out of the right ventricle into the pulmonary outflow tract causes shunting from the right to left circulations through the VSD that is part of TOF (right to left shunt [cyanotic]). All congenital cardiac lesions that shunt blood flow away from the lungs have some obstruction to right heart outflow into the pulmonary circuit. Understanding this principle makes it easier to understand the physiology and anatomy of the congenital lesions.
Anesthetic management of neonates displaying transitional circulation and pediatric patients with congenital cardiac lesions mandate use of medications and techniques that promote control of pulmonary vascular resistance and a balance between the pulmonary and systemic vascular resistances. The goal is to optimize the ratio of pulmonary to systemic circulation as best as anatomically possible.
B. Pulmonary System
Normal Fetal to Pediatric Transition
The pulmonary system is involved in dramatic developmental changes in the transition from fetal to postnatal physiology (1,2). The lungs undergo active development throughout the gestational period and childhood. Alveolar development occurs primarily in the third trimester beginning in the saccular stage (24 to 38 weeks) and peaking in the alveolar stage (36 weeks to 8 years) (1). Infants born prematurely benefit from maternal antenatal administration of glucocorticoids, which promote maturation of the fetal lung and surfactant production.
Surfactant is one of the most important factors contributing to adequate gas exchange during the transition to postnatal life. Surfactant is produced by type II endothelial cells, which proliferate during the alveolar stage. It is a mixture of neutral lipids, phospholipids, and specific proteins with an amphipathic nature, which leads to a decrease in surface tension that stabilizes alveoli and provides alveolar inflation while reducing hydrostatic forces that cause pulmonary edema.
During the transition to extrauterine life, the first breaths lead to an increase in pulmonary arterial oxygen (PO2) and a decrease in partial pressure of carbon dioxide (PCO2), which stimulates pulmonary vascular dilation, decreased pulmonary vascular resistance, and constriction of the ductus arteriosus (Table 33-1).
Table 33-1 Normal Arterial Blood Gas Values in Neonate
Respiratory Function
Respiratory function differs significantly in infants and children. Oxygen consumption is dramatically higher than adult levels, at approximately 7 to 9 mL/kg/min (Table 33-2). The demand for oxygen is met with increased minute ventilation and with an increased ratio of minute ventilation to functional residual capacity (FRC) ratio. However, the FRC is relatively low compared with the minute ventilation. The oxygen consumption is higher, thus infants and children have a lower oxygen reserve and can rapidly develop hypoxemia.
Inhalation induction as well as emergence of anesthesia are faster in infants and children as a result of increased minute ventilation relative to adults.
Table 33-2 Normal Respiratory Function Values in Infants and Adults
Chest wall compliance is higher than that in adults, because the ribs and intercostal muscles are not fully developed, which can lead to significant retractions that do not provide efficient effort for gas exchange. The primary mechanism driving respiratory effort in neonates is the diaphragm, which is easily fatigued when the work of breathing is increased due to increased resistance to ventilation or hyperventilation.
Meconium Aspiration
Fetal hypoxemia may result in intrauterine passage of meconium that mixes with amniotic fluid. The fetal breath movements will then result in pulmonary exposure to meconium prenatally. During birth, infants may also aspirate meconium produced during labor. The latter scenario is consistent with thick meconium that can cause a mechanical airway obstruction. Current Pediatric Advanced Life Support recommendations do not support routine suctioning for infants born with meconium-stained amniotic fluid. Meconium aspiration may result in alveolar damage, which results in impaired oxygenation as well as increased pulmonary vascular resistance.
During resuscitation of a newborn with a very low Apgar score, suctioning may delay other very important therapeutic interventions such as stimulation, assisted ventilation, and chest compressions.
Persistent Pulmonary Hypertension of the Newborn
The pulmonary circulation is highly sensitive to pH and oxygen levels, as well as other mediators such as nitric oxide, adenosine, prostaglandins, and lung inflation (3). During the newborn period, certain factors can impair vasodilation of the pulmonary vascular tree (Table 33-3) and result in elevated pulmonary vascular resistance. Systemic hypotension and cardiac arrhythmias may result, particularly if the right ventricle is not able to compensate and right atrial dilation ensues.
Table 33-3 Factors that Impair Vasodilation of the Pulmonary Vascular Tree
C. Renal System
The kidneys begin to receive increased blood flow after the transition to postnatal circulation. However, the glomerular filtration rate (GFR) remains lower than adult levels for the first two years of life. As a result, infants have impaired ability to retain free water. Therefore, infants are less likely to tolerate prolonged fasting periods, particularly during the first few weeks of life. Their inability to regulate GFR to excrete large amounts of water also leads to an inability to tolerate fluid overload without resulting electrolyte abnormalities. Urine output is initially low, but increases to 1 to 2 mL/kg/hour after the first day of life.
D. Hepatic System
The synthetic and metabolic functions of the liver remain immature in term newborns. The enzymes required for metabolism and drug elimination are present but have not been induced yet (4). The results are variable, depending on the medication and elimination pathways. Morphine relies on hepatic biotransformation for elimination; therefore, it has a prolonged half-life in neonates. Alternatively, lidocaine does not demonstrate prolonged elimination. Synthetic function is also limited, as exhibited by decreased albumin and vitamin K production, which is one of the medications routinely administered at birth to prevent postpartum hemorrhagic complications such as intraventricular hemorrhage.
Neonatal glycogen stores are decreased, especially in preterm infants, thus increasing the risk of hypoglycemia.
E. Central Nervous System
The central nervous system has recently come under close scrutiny in pediatric anesthesia. Specifically, there has been evidence of anesthetic-induced neuroapoptosis in animal models that is being closely studied for implications in humans (5). Data in humans are limited to retrospective studies that suggest an association between administration of general anesthesia under the age of 3 years and an increased incidence of learning deficits (5). The U.S. Food and Drug Administration provided comments on the issue and recommends that medications to provide general anesthesia should be used except for the rare scenario of an infant or child undergoing a purely elective procedure before the age of 3 years (5). In this case, a thorough conversation between the parents, the surgeon, and the anesthesiologist detailing the risks and benefits of the proposed surgical procedure and anesthetic should be undertaken. Most surgical procedures in children are not truly elective, as delaying surgical care can have effects on growth and development at later stages. Furthermore, untreated pain can have detrimental effects on behavioral and neurologic development and should be treated (5).
II. Pharmacology
Administration of appropriate doses of anesthetic agents, analgesics, and all medications in the pediatric setting requires consideration of pharmacologic differences between children and adults (4). Several variables in pediatric patients affect pharmacokinetics. For nearly all parameters, developmental differences are greatest in neonates and premature infants. For example, total body water comprises 70% to 83% of weight in premature babies and term neonates, whereas it is approximately 60% of weight in infants 6 months through adulthood. Increased total body water translates to larger volumes of distribution of hydrophilic medications, which (assuming similar pharmacodynamics) translates to increased dosing requirements per kilogram of body weight. A selection of pharmacokinetic variables and their influence on drug metabolism are presented in Table 33-4. Volatile anesthetic MAC requirements are greatest at approximately 1 month of age.
Table 33-4 Pharmacokinetic Variables and Their Clinical Influence in Infants and Children
III. Equipment
The three factors that affect design and choice of pediatric breathing circuits are excessive resistance to flow, excessive dead space, and decreased heat and humidification. It is for these reasons that a variety of valveless breathing systems have been devised (Fig. 33-3) (1,2).
FIGURE 33-3 Anesthesia breathing circuits. A: Jackson-Rees modification of Ayre’s T-piece. B: Mapleson D circuit. FGF, fresh gas flow. (From Ruitort KT, Eisenkraft JB. The anesthesia work station and delivery systems for inhaled anesthetics. In: Barash PG, Cullen B, Stoelting RK, et al., eds. Clinical Anesthesia. 7th ed. Philadelphia:Wolters Kluwer/Lippincott Williams & Wilkins; 2013:1178–1215, with permission.)
Ayre’s T-piece has no unidirectional gas flow valve and is effective for the spontaneously breathing patient. It is compact, can provide supplemental oxygen, and is not associated with rebreathing of carbon dioxide. Once neuromuscular relaxants were introduced into anesthesia practice, however, Ayre’s T-piece became ineffective, as it was difficult to provide the required positive pressure breathing with this device. The Jackson Rees modification of the Ayre’s T-piece solved this problem while maintaining the valveless system by adding a reservoir bag with a variable occlusion pop-off (a variably occluded pigtail on the bag). Although the Jackson Rees modification of the Ayre’s T-piece solved the need to be able to provide positive pressure breathing, it became apparent that another technical issue had to be solved—the potential for rebreathing carbon dioxide.
Mapleson introduced variations on the Jackson Rees system to address the potential for rebreathing. Mapleson recognized that while rebreathing could occur because this system did not contain unidirectional gas flow valves, the sequential placement of the fresh gas inlet, the pop-off, the reservoir bag, and the connection to the patient can be varied. Depending on whether the patient was breathing spontaneously or was controlled with positive pressure, if the fresh gas flow was sufficient, carbon dioxide rebreathing could be minimized. There are six variations of the Mapleson system, the Mapleson D being commonly used in pediatric patient care. The Mapleson D system places the fresh gas inlet close to the connection to the patient’s airway. The pop-off is farther away from the patient and fresh gas inflow, and the bag is distal to the pop-off. The popularity of the Mapleson D system results from its ability to minimize carbon dioxide rebreathing when controlled ventilation is the ventilatory mode. Rebreathing is also eliminated during spontaneous ventilation when the fresh gas flow is two to three times the patient’s minute ventilation.
Modern-day pediatric anesthesia patient care effectively employs the circle breathing system, without undue resistance to the patient when opening the valves. It has the added benefit of conservation of the patient’s heat and airway humidity. If concern exists that airway heat and humidity will be lost during ventilation with the anesthesia machine, a heater and humidifier can be incorporated into the circle system.
Recent reports demonstrate that by using the proper-sized cuffed endotracheal tube, the ability to provide positive pressure ventilation was enhanced. There was also less operating room anesthetic gas contamination, better isolation of the airway from gastric contents, and less need for additional laryngoscopies in attempts to select the proper size tube.
IV. Perioperative Management
A. Preoperative Assessment
Children should be evaluated for common coexisting conditions (1,2). One of the most common questions relates to children with ongoing upper respiratory infections. A large prospective trial evaluated healthy children scheduled for elective surgery. It assessed their respiratory symptoms for nasal drainage (clear or discolored yellow/green) as well as cough (dry or productive and color of sputum), lethargy, and fever for correlation with respiratory complications, including bronchospasm and laryngospasm (6). The relative risk for respiratory complications was >1.5 if the child had active symptoms, including clear runny nose, green runny nose, moist cough, and fever. A general discussion between the parents, child, surgeon, and anesthesiologist should take place to determine the risk–benefit ratio for every scenario. A thorough preanesthetic discussion with the parents or responsible guardians should include the possibility of admission to the hospital for postoperative management.
The first observation of a child’s behavior offers great insight into the developmental stage, as the developmental stages may not always correlate with a child’s age. Children will naturally express anxiety around strangers and may not tolerate a thorough physical examination. Neurologic examination should focus on the child’s activity level and note any anomalies such as contractures, weakness of extremities, or abnormal appearance. The cardiovascular examination focuses on auscultation of the heart sounds, noting that heart murmurs are common in newborns (patent ductus arteriosus continuous murmur, patent foramen ovale, atrial septal defect, and ventricular septal defect). The presence of a murmur should warrant further exploration of signs or symptoms of cardiac disease, particularly fainting, discoloration such as blue lips, or failure to thrive. Furthermore, the abdominal examination may reveal a large or very small liver, which correlates with volume status as well as the ability for the heart to handle preload.
Pulmonary examination focuses on determining the presence of abnormal air movement, including absent breath sounds, wheezing, or coarse breath sounds. It can often be challenging to differentiate coarse lung sounds from transmitted upper airway sounds in the presence of nasal congestion. The abdominal examination evaluates for signs of trauma, distention, or discomfort. Furthermore, umbilical hernias may be appreciated. The extremities should be assessed for range of motion, contractures, or deformities as well as possible sites for intravenous access. There should be a discussion with the patient and guardians regarding the risks and benefits of delivering general versus regional anesthesia to the child. The risks of anesthesia that are specific to pediatrics include, in particular, respiratory depression, particularly in ex-premature infants, respiratory complications, such as bronchospasm and laryngospasm, hypoxia, and aspiration pneumonia.
Adolescents may be in a position where they can provide assent to a procedure, but ultimately the legal guardian must provide consent.
B. Fasting Guidelines
Children are particularly sensitive to dehydration during preoperative fasting. It is important to emphasize the most recent recommended guidelines by the American Society of Anesthesiologists’ taskforce (7). In general, there is consensus that:
1. Clear liquids should be allowed up to 2 hours before the procedure;
2. Breast milk 4 hours before the procedure;
3. Formula 6 hours before the procedure;
4. Solids about 8 hours or longer (i.e., midnight the night before) before the procedure.
C. Premedication
Oral premedication with a benzodiazepine (e.g., midazolam 0.5 mg/kg up to 10 mg) is an effective method (8). Oral midazolam has a rapid onset (5 to 15 minutes to peak effect) and short duration of action, which makes it well suited for ambulatory surgical procedures.
D. Parental Presence
Another approach to mitigating the stress of separation from parents is to allow a parent or caregiver to be present during induction. This approach is most effective when parents are calm and is not effective for all parents or children (8). In addition to selecting the appropriate parents or children for which to use this approach, to be successful the parent or guardian should be adequately prepared in terms of what to expect during the course of induction. Parents should be coached regarding how they can be most helpful to their child.
E. Induction of Anesthesia
Induction of anesthesia represents a stressful event for children (and parents!). Beyond the mandatory goals of maintaining a patent airway and stable hemodynamics, the goals of a pediatric induction also include a smooth separation from the parent (if a parent is not present for induction) and a cooperative child during the process of induction, while establishing and meeting parental expectations during the process. Two of the most commonly used approaches for minimizing the stress of induction (which may be used alone or in combination) are discussed in the sections that follow (1,2).
Inhalation Induction of Anesthesia
In the absence of contraindications, an inhaled induction

Full access? Get Clinical Tree
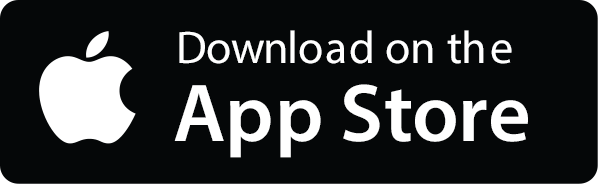
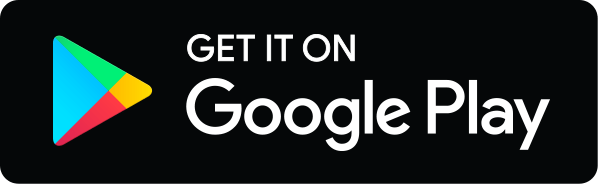