Fig. 4.1
Comparison of size of objects from 10−10 to 1 m. (Used with permission from Kateb B, Chiu K, Black KL, et al. Nanoplatforms for constructing new approaches to cancer treatment, imaging, and drug delivery: what should be the policy? Neuroimage 2011;54:S106–S124)
Equally important to small size for nanotechnology is the concept of constructing or manipulating materials atom-by-atom or molecule-by-molecule. This concept was expressed—and the term “nanotechnology” coined—by Norio Taniguchi in 1974. Two quite different techniques for nanofabrication include (1) “top-down” methods such as nanolithography (Greek “small writing on rocks”) and (2) “bottom-up” methods such as self-assembly (of atoms, molecules, proteins, etc) and chemical vapor deposition (e.g., to produce carbon nanotubes—Fig. 4.2a, b) [7].
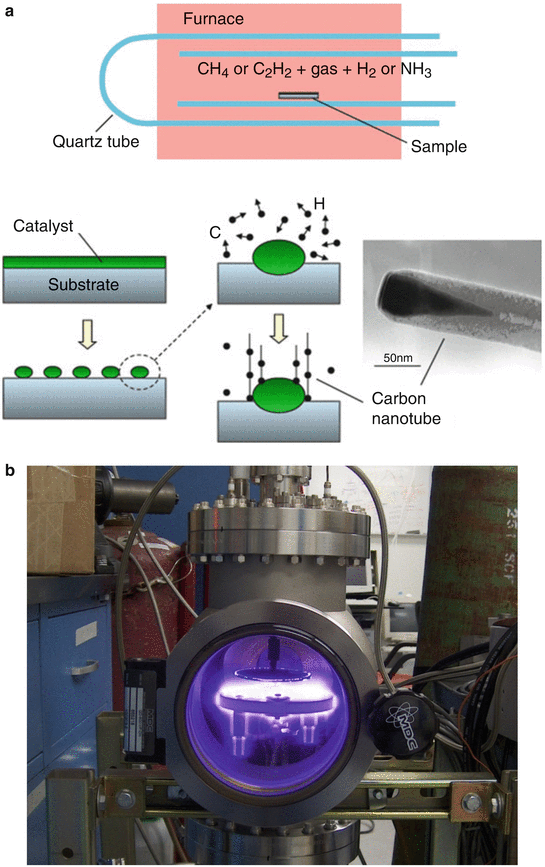
Fig. 4.2
(a, b) (a) Schematics of a chemical vapor deposition reactor (top) and the basic sequence of carbon nanotube growth (bottom). (a: Used with permission from Daraio C, Jin S. Synthesis and patterning methods for nanostructures useful for biological applications. In: Silva GA, Parpura V, editors. Nanotechnology for Biology and Medicine. New York: Springer Science + Business Media; 2012). (b) Photograph of a custom built plasma enhanced chemical vapor deposition reactor. (b: Courtesy of NASA Ames Center for Nanotechnology)
Three types of nanomaterials important for nanomedicine and nanosurgery include nanoparticles (NPs), nanoscaffolds (NSs), and nanotubes/nanofibers. Several types of nanoparticles and nanotechniques for tumor targeting are depicted in Fig. 4.3a–j [8]. Another important nanoparticle is the quantum dot (QD) [9, 10]. QDs (Fig. 4.4a–c) have the unusual property that they fluoresce with a different color depending on their size. QDs are therefore extremely useful for multiple tagging—drugs, tumor cells, receptors, etc.—to follow the various tagged moieties over time and space [11].
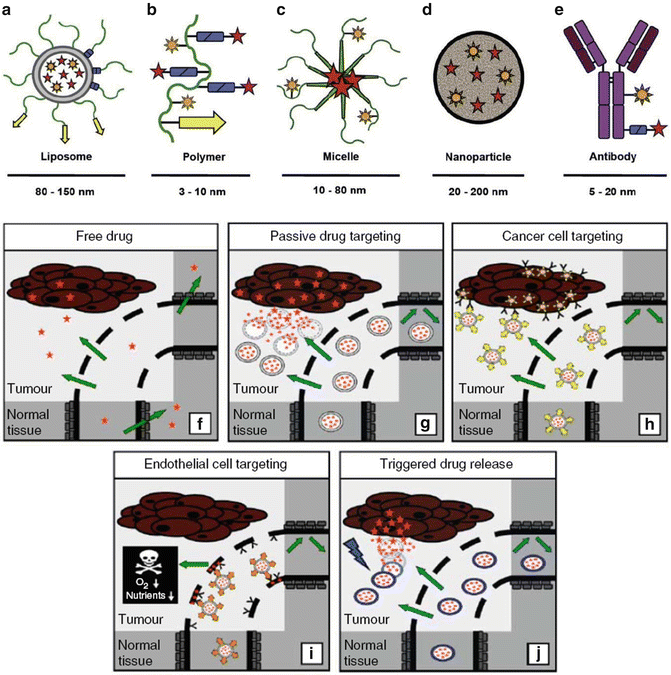
Fig. 4.3
(a–j) (a–e) Examples of nanomaterials, nanotechniques, and nanoparticles: liposomes and liposomal bilayers— gray; polymers and polymer coatings— green; linkers for drug release and “stealth” coatings— blue; targeting ligands— yellow; imaging agents— orange; conjugated or entrapped (chemo-) therapeutic agents— red. (f–j) Drug targeting strategies for tumors. Passive drug targeting with NPs (g) offers some benefit over free drug administration (f) (intravenous) but even more advantageous is active drug targeting of tumor cell surface receptors (h), local endothelial cell receptors (i), and triggered drug release (j) (by stimuli such as hyperthermia, pH change, or ultrasound). (a–j: Used with permission from Rizzo LY, Theek B, Storm G, Kiessling F, Lammers T. Recent progress in nanomedicine: therapeutic, diagnostic and theranostic applications. Curr Opin Biotechnol 2013;24(6):1–13; Lammers T, Kiessling F, Hennink WE, Storm G. Drug targeting to tumors: principles, pitfalls and (pre-) clinical progress. J Control Release. 2012 Jul 20;161(2):175–87; Lammers T, Aime S, Hennink WE, Storm G, Kiessling F. Theranostic nanomedicine. Acc Chem Res. 2011 Oct 18;44(10):1029–38)
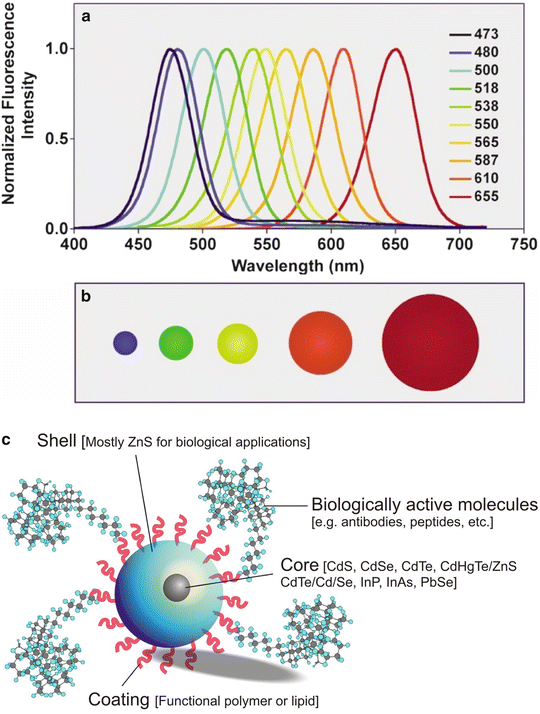
Fig. 4.4
(a–c) (a, b) Graph (a—top) showing that the wavelength of the light emitted by QDs varies with the diameter of the QD (b—bottom). (a, b: Used with permission from Smith AM, Nie S. Chemical analysis and cellular imaging with quantum dots. Analyst 2004;129:672–677.) (c) Structure of a semiconductor QD. The heavy metal core is responsible for the fluorescence properties of the QD. The shell stabilizes the core, while the coating provides anchor sites for organic and biological ligands such as antibodies, and peptides. (c: Used with permission from Pathak S, Cao E, Davidson MC, Jin S, Silva GA. Quantum dot applications to neuroscience: new tools for probing neurons and glia. J Neurosci 2006;26(7)1893–1895)
NSs are typically self-assembling arrays such as the peptide NS illustrated in Fig. 4.5a–c [12]. Peptides composed of charged amino acids self-assemble into scaffolds in the presence of physiological salt solutions and body fluids (e.g., blood, cerebrospinal fluid). The impressive properties of NSs for both hemostasis and tissue repair are discussed below.

Fig. 4.5
(a–c) Self-assembling peptide nanofiber scaffold (SAPNS). (a) Model of the arginine-alanine-aspartate-alanine (RADA) 16-I molecular building block. (b) Model of numerous RADA 16-I molecules which undergo self-assembly to form well-ordered nanofibers with the hydrophobic alanine sandwich inside and the hydrophilic residues outside. (c) SEM of SAPNS (scale bar: 500 nm). (a–c: Used with permission from Ellis-Behnke RG, Liang YX, You SW, et al. Nano neuro knitting: peptide nanofiber scaffold for brain repair and axon regeneration with functional return of vision. PNAS 2006;103(13):5054–5059)
Carbon nanotubes (CNTs) and the closely related carbon nanofibers (CNFs)—see Figs. 4.2a, b and 4.6—have properties that allow novel interactions with tissues, from acting as carriers for antitumor drugs (Smalley’s “nanoscale missiles”) to tissue monitors and modulators with sensitivity orders of magnitude higher than non-nano techniques. Figure 4.7 details some of the advantages of nanoelectrode arrays as biosensors based on size alone; other advantages—particularly important for recording and stimulating nervous system tissues (e.g., neuromodulation and deep brain stimulation)—include greatly reduced impedance and greatly increased capacitance with nanoarrays in comparison with traditional electrodes.
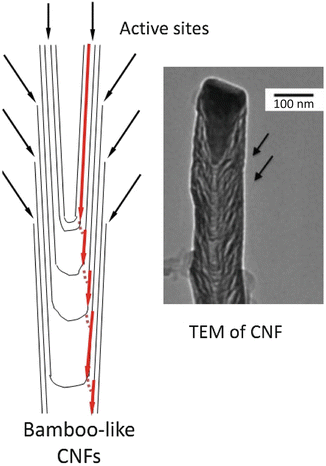
Fig. 4.6
Schematic of a carbon nanofiber showing the bamboo-like (or stacked dixie cup-like) structure of the multiple active sites (left) and TEM of a CNF (right). The multiple active sites result in very high electron transfer and very high capacitance. (Courtesy of NASA Ames Center for Nanotechnology)
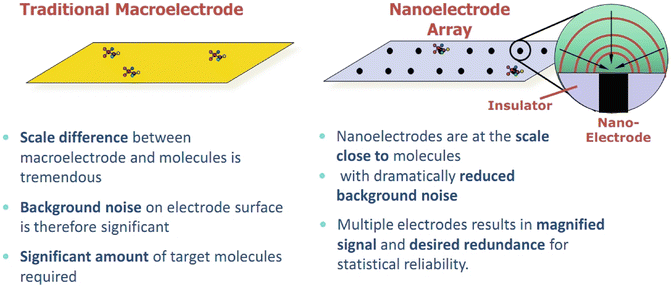
Fig. 4.7
Nanoscale electrodes offer dramatic improvement over traditional macro- or microelectrodes for detecting and quantifying small analytes in low concentrations. (Courtesy of NASA Ames Center for Nanotechnology)
Nanotechniques for Imaging, Targeting, and Healing
Superparamagnetic iron oxide NPs are being developed as improved MRI contrast agents for assessing the cerebral vasculature and malignant brain tumors [13]. Figure 4.8a presents MRI scans (7 T) of the human brain: (1) without contrast, (2) with gadoteridol (0.1 mmol/kg), and (3) with ferumoxytol (510 mg), a superparamagnetic iron oxide NP. Because ferumoxytol particles are much larger than gadoteridol particles (30 nm vs. 1 nm), they tend to leak out of the blood vessels into tumors more slowly (Fig. 4.8b). Gadoteridol enhancement peaks less than 30 min following injection; ferumoxytol enhancement peaks at about 24 h. This offers significant advantages: one can obtain an MRI of the brain immediately after ferumoxytol contrast injection and visualize the cerebral vasculature feeding the tumor, and repeated scans can be obtained over hours following contrast injection without repeating the contrast, especially important for intraoperative MRI scanning where several scans may be required during surgery.
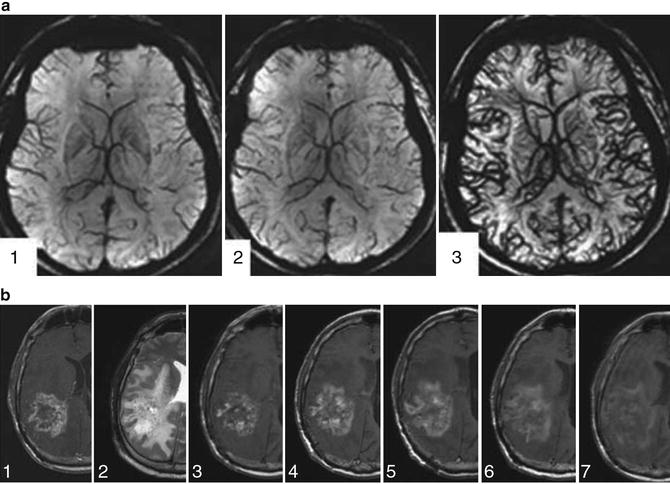
Fig. 4.8
(a, b) (a) Cerebral vasculature with 7T MRI. (1) Without contrast agent. (2) With gadoteridol 0.1 mmol/kg i.v. (3) With ferumoxytol 510 mg i.v. (b) Time course of ferumoxytol enhancement (1.5T MRI) in a patient with recurrent glioblastoma multiforme. (1) T1—gadoteridol. (2) T2. 3–7: T1—at the following five time points (hours post-injection of ferumoxytol): (3) 4–6 h; (4) 6–20 h; (5) 24–28 h; (6) 48–52 h; (7) ~72 h. Peak enhancement with ferumoxytol is at 24–28 h, much later than the ~30 min peak enhancement with gadoteridol. (a, b: Used with permission from Weinstein JS, Varallyay CG, Dosa E, et al. Superparamagnetic iron oxide nanoparticles: diagnostic magnetic resonance imaging and potential therapeutic applications in neurooncology and central nervous system inflammatory pathologies, a review. J Cereb Blood Flow Metab 2010;30:15–35)
Imaging of tumors with either gadoteridol or ferumoxytol relies on passive targeting, i.e., the tumor imaging or targeting depends on the tumor blood vessels being “leakier” than normal blood vessels. The leaky vessels adjacent to the tumor result in slow clearance of the contrast agent or QD-PEG (polyethylene glycol) NPs, causing an accumulation of NPs in the extracellular environment of the tumor. More effective imaging/targeting of tumors occurs with active techniques [8, 9, 14], Active targeting utilizes an antibody against the tumor cells conjugated to the QDs, by which the NPs accumulate on the tumor cell membrane and are incorporated within the tumor cells.
More sophisticated, multimodality NP techniques for tumor imaging and treatment are being developed, as illustrated in Figs. 4.3a–j and 4.9. In Fig. 4.9, a nanocrystal coated with polyethylene glycol (PEG) uses an antibody to target, pH sensitivity to release the chemotherapeutic agent, and magnetic properties for MRI detection [14]. Another technique by which NPs can improve cancer treatment is by thermotherapy (e.g., heating tumors using an MRI scanner once the tumor has been targeted with magnetic NPs) [15]. NPs can also enhance the efficacy of standard external beam radiation therapy for tumors. The radiation interacts with hafnium oxide NPs to generate a much larger number of electrons than radiation therapy alone, resulting in greatly increased treatment effect [16].
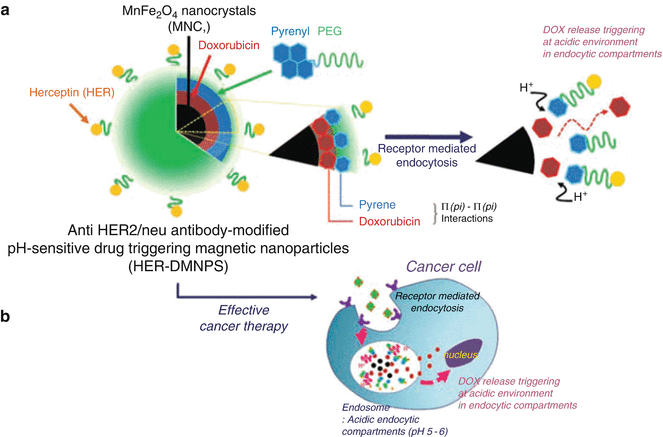
Fig. 4.9
Schematic illustration of anti-HER2/neu antibody-modified pH-sensitive drug-releasing magnetic NPs (HER-DMNPs) for cancer therapy followed by MRI. DOX doxorubicin, HER herceptin, PEG polyethylene glycol. (Used with permission from Wang LS, Chuang MC, Ho JA. Nanotheranostics—a review of recent publications. Int J Nanomed 2012;7:4679–4695)
NPs are also being developed to reduce inflammation and improve healing in conditions ranging from arthritis to infection to endovascular stenting. The use of NPs incorporated into drug-eluting stents (to promote endothelial healing and minimize restenosis) for interventional cardiology has been reviewed [17]. NPs containing aspirin-triggered Resolvin D1 (a hexaenoic acid) have recently been shown to decrease inflammation in several conditions [18]. Examples of this nano-pro-resolving medicine (AT-RvD1 NPRM) are given in Fig. 4.10a, b, where a mouse model of (1) peritonitis (produced by intraperitoneal injection of zymosan) and (2) temporomandibular joint (TMJ) inflammation (produced by local injection of T Freund’s adjuvant) were treated pre-insult by intravenous injection of AT-RvD1 NPRM. Figure 4.10a graphs the decrease in acute inflammation with the peritonitis model when AT-RvD1 NPRM was administered; Fig. 4.10b graphs the decrease in myeloperoxidase activity (MPO) by ELISA 12 h following injection of the TMJ.