Key points
- ▪
Patients with muscular diseases have a postoperative pulmonary complication rate of 25% to 48%. Their inability to take deep inspirations and cough predisposes them to atelectasis and pneumonia.
- ▪
Muscular dystrophies have variable modes of inheritance and clinical presentation, characterized by progressive muscle weakness and often associated with global systemic effects.
- ▪
Both cardiac and respiratory complications are major determinants of quality of life and life span in patients with Duchenne’s muscular dystrophy. The leading cause of death is cardiomyopathy; its severity is unrelated to that of skeletal muscle involvement. Therefore the potential for significant ventricular dysfunction and cardiac conduction defects must be considered in planning anesthesia.
- ▪
Malignant hyperthermia is definitively associated only with triggering agents in patients with central core disease and King-Denborough syndrome. However, volatile anesthetics and succinylcholine may trigger rhabdomyolysis and hypermetabolic responses in myopathic patients.
- ▪
Muscle dystrophies are characterized by increased intramyoplasmic calcium concentration, explaining the increased sensitivity of these patients to inhalational agents and depolarizing relaxants.
- ▪
In patients with myotonias, succinylcholine can induce sustained muscle contractions, preventing adequate ventilation or intubation.
- ▪
Patients with mitochondrial myopathies are at increased risk of developing propofol infusion syndrome.
- ▪
Myoglobinuria is a common metabolic abnormality among the substrate use muscle diseases. Alkalinization of urine with sodium bicarbonate may prevent precipitation of hematin in the renal tubules and reduce the risk of renal failure.
- ▪
Patients with myasthenia gravis are at risk for postoperative controlled ventilation and should be assessed preoperatively using the MG severity score.
Since the writing of this chapter by J. D. Miller and H. Rosenbaum, the genetic defects and molecular mechanisms involved in many of the muscle diseases have been elucidated. In some cases this has facilitated the management of patients with muscular disorders. However, it still requires an astute clinician to make the diagnosis of a muscle disease and to assess the magnitude of the compromise in normal physiologic functions. Common complaints such as fatigue, weakness, and polymyalgias are nonspecific symptoms, with etiology extending from neuromuscular disorders through rheumatologic problems to psychiatric conditions. A detailed neuromuscular physical examination and routine diagnostic testing, including electromyography (EMG), serum electrolytes, thyroid function tests, and serum creatine kinase evaluation, may not provide a definitive diagnosis. In fact, many of these patients may have a final, nonspecific diagnosis of “fibromyalgia” or chronic fatigue syndrome. However, some will have life-threatening muscle disorders that must be recognized if these patients are to be treated effectively.
Perioperative respiratory complications are a major concern for patients with muscular diseases. General anesthesia and surgery, particularly abdominal and thoracic procedures, result in postoperative pulmonary changes, specifically a loss of lung volumes and an increase in the alveolar-arterial gradient. This is the reason for a postoperative pulmonary complication rate of 25% to 48%. Patients with chronic respiratory muscle weakness have a higher incidence of postoperative respiratory complications because of the loss of respiratory reserve. In addition, their inability to take deep inspirations and cough makes them more susceptible to atelectasis and pneumonia. However, a preoperative diagnosis of respiratory compromise may be difficult in patients with skeletal muscle weakness, because their respiratory reserve is rarely challenged. Preoperative pulmonary function testing of these patients provides an assessment of the severity of the pulmonary disease and potential pulmonary risk of undergoing the planned procedure. Patients with deteriorating skeletal muscle strength can also develop kyphoscoliosis with concomitant restrictive lung disease.
If possible, administration of muscle relaxants should be avoided, as should long-acting agents, if required. Hypomotility of the gastrointestinal (GI) tract may lead to delayed gastric emptying and increase the risk of pulmonary aspiration. This would favor securing the airway using rapid-sequence induction with succinylcholine, although in some patients this has been associated with ventricular fibrillation, rhabdomyolysis, and malignant hyperthermia.
Because some patients with muscle disease will also have a cardiomyopathy, the depressant effects of volatile anesthetics may provoke congestive heart failure (CHF). The respiratory depressant effects of a narcotic anesthetic, however, may require prolonged postoperative respiratory support. In all cases, after general anesthesia the clinician must anticipate the possibility of postoperative ventilation and, once extubated, the need for intensive respiratory therapy. Regional anesthesia avoids some of the complications of general anesthesia and may provide a vehicle for postoperative analgesia without employing narcotics. However, even if regional techniques are employed to reduce anesthetic requirements and provide postoperative pain management, it may still be necessary to protect the airway and ensure adequate oxygenation during the procedure through the use of general endotracheal anesthesia.
These points are all common considerations when anesthetizing patients with muscle disease. This chapter delineates the characteristics of specific muscle diseases and the impact on perioperative anesthetic planning.
Muscular dystrophies
Muscular dystrophies are a group of hereditary myopathic diseases characterized by progressive weakness. Clinical presentation is heterogeneous, from severe fatal childhood forms to relatively benign adult forms. The dystrophies are all best characterized by painless degeneration and atrophy of skeletal muscles without evidence of muscle denervation. Originally these diseases were characterized on the basis of their clinical presentation (e.g., limb-girdle myopathy). The discovery of dystrophin and related molecules has given “muscular dystrophy” a molecular biologic basis for diagnosis, genetic mapping, and treatment. Dystrophin is a large (427-kbp) rod-shaped protein representing about 5% of membrane-associated cytoskeletal protein; its gene is located on the short arm of the X chromosome. Along with several other sarcolemmal proteins, dystrophin stabilizes the muscle surface membrane during contraction and relaxation ( Fig. 9-1 ). The dystrophin-associated complex binds intracellular actin to the extracellular basal lamina, which mechanically stabilizes the sarcolemma during muscle contraction.

Pathophysiology
Muscular dystrophies are characterized by degeneration of the skeletal muscle fibers and replacement with fibrous and fatty connective tissue, without accumulation of intermediate metabolic substrates. There is no evidence for direct neurologic involvement. The breakdown of the muscle fiber sarcolemma occurs early in the disease, with an influx of calcium and the activation of proteases, and the eventual destruction of tissue by inflammatory elements. The absence of dystrophin results in a loss of skeletal muscle membrane integrity and subsequent breakdown of the sarcolemma with an influx of extracellular calcium, activation of cellular proteases, inflammation, necrosis, and replacement fibrosis.
Duchenne’s muscular dystrophy (DMD, also called pseudohypertrophic muscular dystrophy) is the most common form, seen in 1 per 3500 births. The myopathy is associated with mutations of the dystrophin gene located in the Xp21 stripe, inherited as a sex-linked recessive trait; most of the reported cases are male. However, well-documented cases in females range in severity from full DMD to mild weakness. Transmission of this disease in female offspring of normal fathers can occur when there is early inactivation of the normal X chromosome (Lyon hypothesis). Only one X chromosome is active in any cell, with inactivation of the other X-chromosome occurring early in embryogenesis. Some heterozygotic females with DMD would then be expected to carry the abnormal X chromosome as the only active dystrophin gene in most cells. Female children with Turner’s syndrome (XO) would also present with DMD. It is unclear whether heterozygotic females pose the same anesthetic risks as males with DMD. Although DNA testing can detect multiple deletions, duplications, and point mutations in the dystrophin gene, proximal limb muscle biopsy remains the standard for diagnosis. Skeletal muscle biopsy early in the disease process may demonstrate necrosis and phagocytosis of muscle fibers, as well as areas of vigorous muscle regeneration. Immunostaining reveals the complete lack of dystrophin at the surface of the muscle fibers.
Diagnosis and Differential
Progressive and symmetric skeletal muscle weakness and wasting with histologic evidence of dystrophic muscle changes are diagnostic of DMD. The initial clinical presentation involves a waddling gait, frequent falling, and difficulty climbing stairs because of proximal muscle weakness in the pelvic girdle. Also, weakness in the shoulder girdle and trunk erectors leads to thoracolumbar scoliosis. Certain muscles, particularly the calves, demonstrate early hypertrophy. The pelvic girdle and proximal leg muscle weakness is responsible for Gowers’ sign, with the child climbing up the legs to stand up. However, the child’s condition usually goes undiagnosed until age 3 to 5 years. This is rapidly followed by atrophy of the other proximal muscles. All muscles are ultimately involved except for the cranial muscles and the external anal sphincter. Because of lack of denervation, there is intact sensation, but the proximal deep tendon reflexes (DTRs) disappear in half of patients by age 10. The earlier the onset, the more rapid is the downhill course. Usually the child is unable to walk by age 9 to 11 years. Joint contractures appear during this period because of the uneven loss of agonist and antagonist muscle groups.
In the heart, loss of dystrophin affects l -type calcium channels with increased intracellular calcium. The excess calcium activates proteases that degrade the contractile proteins, leading to inflammation and myocardial cell death and fibrosis, producing a dilated cardiomyopathy. Cardiomyopathy is the major cause of death in DMD patients. The timing and severity of the cardiomyopathy in DMD is unrelated to the severity of skeletal muscle degeneration. Although all patients with DMD will eventually develop cardiomyopathy, symptoms may not become apparent because of their inability to exercise. Uncharacteristic symptoms of CHF in this population may include sleep disturbances, loss of appetite, nausea, abdominal pain, cough, or increased secretions. These patients will have a resting tachycardia. Physical findings of a cardiomyopathy on examination may include jugular venous distention, displacement of the point of maximal impulse (PMI), and S 3 /S 4 gallop. Scarring of the posterobasal portion of the left ventricle produces tall, right precordial R waves and deep, left precordial Q waves in the electrocardiogram (ECG). Mitral regurgitation may also be present because of papillary muscle dysfunction.
The “gold standard” for assessing cardiac function in DMD patients is the two-dimensional echocardiogram. However, cardiac magnetic resonance imaging (MRI) has been shown to be a more reliable noninvasive measure of cardiac function in DMD patients. In some cases, gadolinium contrast has been used in conjunction with cardiac MRI to visualize fibrotic areas. Several studies have shown the benefits of steroid therapy on skeletal, respiratory, and cardiac muscle function; steroid treated DMD children maintained normal cardiac function longer than untreated DMD children. In addition, angiotensin-converting enzyme (ACE) inhibitors slow the progression of cardiac dysfunction in DMD patients.
Duchenne’s dystrophy produces respiratory muscle weakness, which places these patients at increased risk for perioperative pulmonary complications . Respiratory muscle weakness is detectable by age 10, but the diaphragm is usually spared. There is a progressive decrease in total lung capacity and vital capacity. Inability to cough and clear secretions predisposes these patients to pneumonia, which is often fatal by about the third decade. However, about 15% of patients have a much slower disease course, which stabilizes about the time of puberty. These patients also have lower-than-average intelligence with mild cerebral atrophy, presumably from the lack of normal brain dystrophin; initiating perioperative incentive spirometry may therefore prove difficult. Some clinicians believe that exercise enhances muscle destruction; however, physical therapy that involves passive movement to prevent contractures and resistance exercises for the lungs to increase endurance may be helpful. Because contractures represent a major disability, DMD patients often have elective procedures to relieve these contractures. Thus, perioperative complications that prolong their inactivity may actually exacerbate their condition by preventing the important postoperative physical therapy.
The American College of Chest Physicians 2009 consensus statement on the respiratory management of patients with DMD recommends preoperative assessment of oxygenation, lung volumes, and cough strength ( Table 9-1 ). Oxygen (O 2 ) saturation below 95% on room air by pulse oximetry is considered a “clinically significant abnormality” and warrants further assessment, which may include arterial blood gas (ABG) analysis. Assessment of lung volumes involves measuring forced vital capacity (FVC) in the seated, upright position. DMD patients with FVC less than 50% should receive preoperative training with noninvasive positive-pressure ventilation (NPPV) to facilitate postoperative extubation. In addition, because the coughing and clearing of secretions are important aspects of pulmonary toilet after surgery, preoperative measurement should include maximum expiratory pressure (MEP) and peak cough flow (PCF). Using these values as guidelines, patients should receive preoperative training in the postoperative use of manual and mechanically assisted cough devices.
Assessment | Monitoring Level | Recommendation/Evaluation |
---|---|---|
Oxygenation | Sp o 2 <95% on room air by pulse oximetry | Requires measurement of Pa co 2 |
Lung volumes | FVC in seated, upright position | |
<50% of predicted | Increased risk of perioperative respiratory complications | |
<30% of predicted | High risk of perioperative respiratory complications | |
Cough strength | MEP <60 cm HOH PCF <270 L/min | Preoperative training in assisted cough devices |
In addition to the cardiac and pulmonary manifestations, DMD effects are systemic with the possible need for neurologic, GI, nutritional, and physical therapy support. Many of these patients have elevated plasma muscle enzymes aldolase and creatine kinase (CK) levels early in disease progression. The MB fraction of CK, normally present only in heart muscle, cannot be used as a guide to cardiac injury because it is also elevated as a result of the destruction of regenerating skeletal muscle in DMD. CK levels are highest (50-100 times normal) up to age 3 years and then decrease by about 20% per year as muscle atrophies. Increased plasma levels of liver enzymes have also been noted (aminotransferases/transaminases, lactate dehydrogenase); however, liver damage has not been described in DMD, suggesting a skeletal origin.
Table 9-2 lists the other, less common forms of muscular dystrophy and their clinical course. Becker’s muscular dystrophy (BMD) is an allelic variant of DMD in which the mutated dystrophin gene produces a reduced amount of a truncated dystrophin protein. The pace of muscle destruction in BMD is much slower than in DMD, with symptoms usually appearing in early adolescence. Most BMD patients will develop a cardiomyopathy by age 30, which may not correlate with the degree of skeletal muscle destruction. BMD is usually fatal from respiratory or cardiac complications between ages 30 and 60 .
Dystrophy | Inheritance | Clinical Course | Comorbidities and Anesthetic Concerns |
---|---|---|---|
Becker’s muscular dystrophy (BMD) | X-linked, same locus as DMD Reduced amount and abnormal dystrophin | Later onset (age 12 yr) More benign course Death in early 40s, most often from pneumonia | Cardiac involvement is less frequent and less severe, but heart failure is common cause of death. Pseudohypertrophy common Reports of cardiac arrests intraoperatively and postoperatively (patients at risk for rhabdomyolysis) |
Emery-Dreifuss muscular dystrophy (EDMD) | X-linked | Slow progression Early contractures in elbow, ankles, and neck Significant cardiac risk with sudden death often between ages 30 and 60 years | Early atrial arrhythmias progressing to asystole: prophylactic ventricular pacemaker suggested Possible cardiomyopathy, ventricular fibrosis, and cardiomegaly Possible difficult intubation from limited neck motion (although flexion is more limited than extension) |
Rigid spine syndrome | X-linked? | Slow progression Painless limitation of neck and trunk motions | Severe restrictive lung disease Weak respiratory muscles Cardiomyopathy Scoliosis Difficult intubation |
Facioscapulo-humeral (Landouzy-Dejerine) dystrophy (FSHD) | Autosomal dominant inheritance | Onset in adolescence Weakness of pectoral, orbicularis, shoulder, and pelvic muscles (less than DMD) Life span minimally affected | Rare cardiac involvement Abnormal vital capacity Normal CO 2 response curve Frequent upper respiratory tract infections Postoperative respiratory complications |
Limb-girdle muscular dystrophies (LGMDs) | Five subtypes predominantly autosomal recessive Severe childhood autosomal recessive dystrophy gene located on 17q12-21 | Two most common subtypes: Erb’s type (early onset, shoulder girdle primarily involved) Leyden-Möbius (late onset, pelvic girdle involvement) Severity between DMD and FSHD | Variable cardiac involvement Sinus tachycardia and right bundle branch block most common ECG abnormalities Early severe diaphragmatic weakness (hypoventilation, hypercarbia) Heart transplant in severe childhood autosomal recessive dystrophy |
Distal myopathies | Autosomal dominant | Welander’s myopathy: onset after age 30 Seen mostly in Sweden Mainly affects hands Markesberry’s: onset in fifth decade of life,feet involved Early adult-onset myopathy: involvement of anterior or posterior compartment of legs | Possible cardiomyopathy secondary to interstitial fibrosis of the heart muscle in Markesberry’s dystrophy |
Oculo-pharyngeal muscular dystrophy | Onset after age 30 years, slow progression Weakness of pharyngeal muscles, ptosis, limbs, extraocular muscles (rare diplopia) Similar symptoms to ocular myasthenia gravis No dysarthria, dyspnea | Dysphagia, dyscoordination of posterior pharynx, and esophageal involvement cause aspiration and inanition. Sensitivity to muscle relaxants; anticipate mechanical ventilation postoperatively Anticholinesterase agents do not reverse weakness. Normal sensitivity to vecuronium in case report | |
Congenital muscular dystrophy | Fukuyama’s form, autosomal recessive | Onset at birth Proximal more than distal muscles involved Slow progression Creatine kinase slightly elevated Death by age 10 in Fukuyama’s form (seen frequently in Japan) | Seizures and mental retardation in Fukuyama’s form |
Facioscapulohumeral dystrophy (FSHD) is the third most common muscular dystrophy, with a pattern of progressive muscular weakness involving the face, scapular stabilizers, proximal arm, and fibula. Patients with FSHD usually present with shoulder weakness and scapular winging. FSHD is also associated with retinal abnormalities and hearing loss, but the cardiac muscle is usually spared.
The limb-girdle muscular dystrophies (LGMDs) are a heterogeneous grouping of sarcoglycanopathies, a class of transmembrane proteins that associate with dystrophin in a glycoprotein complex. Common clinical features include early involvement of the proximal muscles of the legs, followed by shoulder muscles with scapular winging. Affected individuals have a characteristic stance of lordosis, abducted hips, and hyperextended knees. The facial and ocular musculature is usually spared. There are usually no associated cognitive or cardiac abnormalities. Onset of LGMD is in late childhood, with slow progression. In contrast to most muscular dystrophies that affect the proximal musculature, the distal myopathies affect the forearms, hands, and lower legs.
Anesthetic Considerations
Patients with muscular dystrophies often require surgery for muscle biopsy, the correction of scoliosis, the release of contractures, and exploratory laparotomy for ileus ( Box 9-1 ). The surgical risk is the lowest early in the disease course, before the patient has significant comorbidities. Thus, it is imperative to determine the severity of the disease and the associated comorbidities. Among patients with muscular dystrophy, 50% to 70% demonstrate some cardiac abnormality, although these are clinically significant in only 10% of patients and often in the terminal phase of the disease. No correlation has been established between the severity of the cardiac disease and the severity of the skeletal disease. Necrosis and fibrosis of the myocardium in DMD is typically limited to the posterobasal and lateral free walls of the left ventricle, whereas in the other muscular dystrophies the fibrosis may be more diffusely dispersed. Dysrhythmias occur frequently, even after minor trauma. Complex ventricular premature beats correlate with both abnormal left ventricular function and an increased incidence of sudden death. These patients probably have impaired cardiac autonomic function with increased sympathetic tone, explaining the resting tachycardia and the propensity to develop arrhythmias.
Potent Inhalational Anesthetics
Use is not recommended because potent inhalational anesthetics may trigger a malignant hyperthermia–like syndrome in patients with Duchenne’s muscular dystrophy and depress myocardial contractility.
Hypnotics
Pentothal should be given in small increments, when used.
Propofol has been recommended as the preferred hypnotic, but higher-than-expected doses may be required for induction.
Consider the myocardial status of the patient. Some patients have significant cardiomyopathy, and reduced heart rate and decreased contractility with an induction dose of propofol may lead to profound hypotension and reduced end-organ perfusion.
Opioids
Use of narcotics eliminates the need for myocardial depressants or inhalational agents; however, consider the use of short-acting opioids and the need for postoperative ventilation.
Muscle Relaxants
Administration of nondepolarizing muscle relaxants is usually followed by an increased response, both in maximal effect and duration of action.
Recovery from neuromuscular blockade in muscular dystrophy patients has been reported to be three to six times longer than in healthy adults. In addition, postoperative pulmonary complications have been associated with the use of long-acting neuromuscular blockers.
The combined effects of primary smooth muscle abnormalities, inactivity, and general anesthesia induces gastric dilation, delayed gastric emptying, and the risk of pulmonary aspiration.
Regional anesthesia may be a good alternative to general anesthesia to avoid the risk of triggering agents and respiratory depression and to allow the use of local anesthetics for postoperative analgesia.
Patients undergoing surgery should have a recent echocardiogram. Echocardiography will demonstrate mitral valve prolapse in 10% to 25% of patients. It may also show posterobasilar hypokinesis in a thin-walled ventricle and a slow relaxation phase with normal contraction, characterizing the cardiomyopathy seen in DMD. However, preoperative echocardiography may not always reflect the ability of the diseased myocardium to respond to perioperative stress. Heart failure can occur during anesthesia for major surgery even with normal preoperative echocardiography and electrocardiography, and sudden death can occur even in patients with fully compensated cardiac status. Angermann et al. advocate the use of stress echocardiography using angiotensin to detect latent heart failure and identify inducible contraction abnormalities.
Atrial and atrioventricular conduction defects with bradycardia are common in Emery-Dreifuss muscular dystrophy (EDMD), and again, the severity of heart disease does not correlate with the degree of skeletal muscle involvement. Several anesthesiologists have recommended preoperative prophylactic cardiac pacing in EDMD patients undergoing general anesthesia and having emergency pacing available when any form of anesthesia is used. Regional anesthesia has been used successfully in EDMD patients for lengthening of both Achilles tendons; in one case a temporary transvenous pacemaker was inserted before administration of the anesthetic. In addition, EDMD patients may prove difficult to intubate and careful assessment with cervical radiography should be undertaken preoperatively. A total intravenous anesthetic (TIVA) or a nitrous/narcotic technique that omits volatile anesthetics and depolarizing agents, to avoid malignant hyperthermia–triggering agents, would seem appropriate if a general anesthesia technique cannot be avoided. To date, however, malignant hyperthermia has not been described in EDMD.
Perioperative respiratory complications are a major concern when anesthetizing patients with muscular dystrophy. As previously discussed, at the end of the first decade of life, reductions in inspiration, expiration, vital capacity, and total lung capacity become prominent and reflect the weakness of respiratory muscles. Decreased ability to cough and the accumulation of oral secretions predispose muscular dystrophy patients to postoperative respiratory tract infections. Respiratory insufficiency, however, may not be apparent because impaired skeletal muscle function prevents these patients from exercising enough to exceed their limited breathing capacity. Preoperative pulmonary function studies are valuable in determining the postoperative course of these patients. Patients with a vital capacity of greater than 30% of the predicted value can usually be extubated immediately after surgery. With progression of the disease (vital capacity less than 30% of predicted) and the added morbidity of kyphoscoliosis, which can contribute to a restrictive respiratory pattern, postoperative ventilatory support will be required. Delayed pulmonary insufficiency may occur up to 36 hours postoperatively, even if the patient’s skeletal muscle strength apparently returned to preoperative level. Sleep apnea may also compound the respiratory problems and may contribute to development of pulmonary hypertension. Preoperative introduction to chest physiotherapy and nasal continuous positive airway pressure (CPAP) and their use early in the postoperative period have been effective in decreasing the incidence of respiratory complications.
Sometimes the first indication that a child has muscular dystrophy is an unexplained cardiac arrest or myoglobinuria with malignant hyperthermia–like findings during general anesthesia. In patients with muscular dystrophies, do inhalational agents and succinylcholine trigger malignant hyperthermia? In 2000, Breucking et al. investigated 200 families with muscular dystrophy of the Duchenne and Becker types who had received a total of 444 anesthetics. Sudden cardiac arrests occurred in six patients with undiagnosed disease at the time they received a general anesthetic of an inhalational agent and/or succinylcholine. There were also nine, less severe incidents of fever, rhabdomyolysis, and masseter spasm. The authors recommended the avoidance of the triggering agents—succinylcholine and volatile anesthetics—to decrease the risk of severe anesthetic complications. In earlier reports, Cobham and Davis (1964) and Richards (1972) found no temperature rise or cardiac arrest after using virtually all anesthetic agents available at that time in DMD patients. Richards reported using halothane 37 times and succinylcholine 12 times, all without subsequent problems.
Nevertheless, since those publications, several case reports have described life-threatening complications (dysrhythmias, cardiac arrest, rhabdomyolysis) after anesthesia with muscle relaxants and inhalational agents. The anesthetic complications often seemed to parallel the severity of the muscle disease. Succinylcholine has been involved in the majority of lethal complications in patients with unsuspected DMD, leading the U.S. Food and Drug Administration in 1992 to issue a warning with regard to the administration of succinylcholine in young children and adolescents. Larach et al. reported that 48% of pediatric patients with cardiac arrest during anesthesia had an unrecognized myopathy, with 67% of these associated with succinylcholine-induced hyperkalemia. An inherent membrane defect in DMD may render the muscle more susceptible to injury induced by inhalational anesthetics and depolarization with succinylcholine. Patients with DMD may have an upregulated isoform of the acetylcholine receptor that is more sensitive to the effects of succinylcholine. These agents probably trigger rhabdomyolysis and nonspecific hypermetabolic responses in DMD patients rather than malignant hyperthermia. Case reports have documented the use of propofol, narcotics, and nondepolarizing muscle relaxants in DMD patients without complications, but as with the earlier series of uneventful anesthetics with triggering agents, large series are required to document their safety. In patients with DMD, onset of nondepolarizing neuromuscular blockade may be significantly delayed, which must be considered when rapid-sequence induction is required. However, the prolonged recovery from neuromuscular blockade may require postoperative ventilation.
Myotonias
Myotonia is derived from the Greek word meaning “muscle stiffness.” In these muscle diseases, alterations in the ion channels allow the muscle membrane to become easily depolarized, resulting in repetitive discharges. The myotonias are divided into dystrophic and nondystrophic groups. The dystrophic patients have progressive muscle wasting and weakness (myotonic dystrophy). The nondystrophi c group has an alteration in the electrical hyperexcitability of muscle fibers, leading to prolonged relaxation after voluntary muscle contraction. In the myotonias the pathognomonic finding is muscle stiffness, which consists of slowed muscle relaxation after vigorous contraction (Thomsen, Becker). In some patients the stiffness may resolve with repeated muscle contractions, whereas in others it may be exacerbated. It is usually worse if a period of rest is followed by a period of exercise, and it can be provoked by cold.
Myotonia results from an abnormality in the electrical properties of the sarcolemma, predisposing the muscle membrane to becoming easily depolarized. This results in a characteristic EMG pattern of repetitive discharges (myotonic runs). A diagnostic clinical sign of myotonia is percussion myotonia; after being struck by a percussion hammer, the muscle continues to contract for a time and becomes transiently indented.
Myotonic Dystrophy
Myotonic dystrophy (dystrophia myotonia, DM; Steinert’s disease) is extremely variable in presentation, from asymptomatic cases to congenital DM with respiratory insufficiency and mental retardation ( Table 9-3 ). It is an autosomal dominant inherited neuromuscular disorder with a worldwide prevalence of 1 in 8000. The genetic defect is a result of the abnormal expansion of the nucleotide CTG on chromosome 19, which codes for a serine-threonine protein kinase. The relationship between the genetic defect and the clinical findings is still unknown. However, knockout mice that completely lack this enzyme develop normally. Expressivity of the genetic defect must also be variable; both minimally affected and severely affected individuals may be seen within a family.
System | Manifestations |
---|---|
Neuromuscular | Myotonia, weakness Reduced deep tendon reflexes |
Ocular | Cataract, ptosis Ophthalmoparesis, retinal pigmentation |
Endocrine | Testicular atrophy, diabetes, pituitary dysfunction, hyperparathyroidism |
Skin | Frontal balding, pilomatrixoma |
Cardiovascular | Hypotension, syncope, palpitations, mitral valve prolapse, sudden death |
Gastrointestinal | Dysphagia, pseudo-obstruction |
Central nervous | Mental retardation |
Immune | Reduced immunoglobulin levels |
Four types of myotonic dystrophy are described: congenital (CDM), juvenile or early onset (JDM), adult onset , and late onset . The most severe form is CDM, which presents as hypotonia rather than myotonia. CDM has a high mortality during the neonatal period from respiratory distress. Cranial muscle weakness occurs at birth, which gives these infants a characteristic tent-shaped mouth. JDM children may have some facial weakness early in life, but major muscle weakness is often delayed and accompanied by some mental retardation. In adult-onset DM, symptoms appear during the second and fourth decades of life, with progressive muscular weakness. Muscle weakness and wasting are the most disabling features of DM. Wasting is usually most prominent in the cranial musculature and distal limb muscles. Temporalis and masseter muscle atrophy leads to the classic appearance of the “hatchet face.” DTRs are usually reduced or absent. Weakness of the muscles of the vocal cord apparatus results in nasal speech and propensity for aspiration pneumonia. The distal limb muscles first affected lead to footdrop and weak handshake.
Myotonic dystrophy is a multisystem disease and can affect the heart (conduction system), smooth muscle (impaired intestinal motility), eye (cataracts), brain (mental retardation), and endocrine system (e.g., testicular atrophy, insulin resistance, hypometabolism). Cardiac conduction abnormalities are common and may cause sudden death. In one report, 57% of DM patients had conduction defects, one third of whom had first-degree atrioventricular block unresponsive to atropine. Many of these patients also have an associated cardiomyopathy, and CHF can be a cause of death. Because anesthetics can increase vagal tone or induce arrhythmias, transthoracic pacing should be readily available.
The pulmonary complications of DM are the result of chronic aspiration and central hypoventilation. Weakness of the respiratory muscles causes alveolar hypoventilation, hypercapnia, and hypoxemia, resulting in increased somnolence. In some patients an increased respiratory effort is required in one group of respiratory muscles (diaphragm) to overcome the myotonia in other respiratory muscles (intercostals). Smooth muscle atrophy leading to poor gastric motility, coupled with a diminished protective cough reflex, promotes aspiration. Recurrent aspiration pneumonia can lead to chronic pulmonary damage such as bronchiectasis and pulmonary hypertension. The hypersomnolence seen with DM is often associated with carbon dioxide (CO 2 ) retention and appears to be primarily a central nervous system (CNS) manifestation of the disease.
A recently recognized variant of DM is proximal myotonic myopathy (PROMM). This disorder has features in common with DM (e.g., facial muscle weakness, frontal balding), but the muscle weakness and stiffness is predominantly confined to proximal rather than distal muscles and usually appears in adolescence.
Anesthetic considerations
Because myotonic dystrophy is a systemic disease, anesthetic management must include consideration of the multiple manifestations of the disease. All these patients must be treated as though they have both cardiomyopathy and cardiac conduction defects. Medications that increase vagal tone or anesthetic plans causing hypoxia may result in high cardiac conduction blocks. Therefore, transthoracic pacing and antiarrhythmic medications should be readily available. If possible, inhalational agents should be avoided because of their myocardial depressant and conduction system effects. These patients are at risk of aspiration and should remain NPO (nothing by mouth), and relatively rapid protection of the airway should be achieved with an endotracheal tube.
Rather than relaxation, however, succinylcholine will produce contractures lasting for several minutes. These contractures can be severe enough to prevent intubation and ventilation and are not inhibited by nondepolarizing agents. Other agents may also induce myotonic contractures, including methohexital and etomidate, as well as propofol. The reversal of neuromuscular blockade by neostigmine could precipitate a myotonic response; thus it is advisable to use shorter-acting nondepolarizing muscle relaxants or avoid relaxation. Myotonic contractions can occur, however, even in the presence of neuromuscular blocking agents and neuraxial anesthesia, because direct stimulation of the muscle (surgical stimulation) may result in contraction.
The combination of central respiratory depression and weak respiratory musculature makes myotonic patients vulnerable to the respiratory depressant effects of most sedatives, hypnotics, and narcotics. Therefore, when possible, regional anesthesia is the preferred anesthetic, and when general anesthesia is required, the patient must be monitored postoperatively. The myotonic responses to DM can be treated with phenytoin (4-6 mg/kg/day) or quinine (0.3-1.5 g/day).
Myotonia Congenita
Myotonia congenita, a distinct entity from the congenital onset of DM, is characterized by two forms, one described by Thomsen in 1876 as autosomal dominant and the other by Becker in the 1950s as autosomal recessive inheritance. Both forms are the result of mutations in the gene that codes for the major chloride channel. The Thomsen variant is a mild disease with generalized myotonia, usually recognized in early childhood because of frequent falling. Cranial and upper-limb musculature is most severely affected, sometimes resulting in difficulty chewing. The myotonic responses occur after a rest interval and may result in the patient falling to the ground in a rigid state. Some patients have an athletic appearance as a result of muscle hypertrophy. Many patients have lid lag and blepharospasm, which involves myotonia of the lid musculature. The Becker recessive variant is similar to the dominant form, except that the myotonia is usually more severe and presents later in life (after age 10) and does not progress in severity beyond the third decade. These patients are usually handicapped in their daily activities because of leg muscle stiffness and generalized weakness. The stiffness of myotonia is treated with medications that reduce the increased excitability of the cell membrane by acting at the sodium channel (local anesthetics, antiarrhythmics).
Myotonia Fluctuans
Becker also described individuals with the dominant form of nondystrophic myotonia in which muscle stiffness fluctuated from day to day. These individuals do not experience muscle weakness, but rather have a prolonged time for relaxation after voluntary muscle contraction and external mechanical stimulation. The stiffness (contractures) that occurs after heavy exercise may last 30 minutes to 2 hours, with days or weeks between incidents. Succinylcholine has triggered myotonic crisis, with difficulty in ventilation and intubation, and thus should be avoided in these patients. The duration of neuromuscular blocker therapy will be increased in patients with myotonia fluctuans, but anticholinesterases should be avoided because they may trigger a myotonic crisis.
A variant of myotonia fluctuans has been described in which the myotonia occurs during exercise and is not relieved by warming a cold limb. In another variant, with persistent and sometimes severe myotonia, increased serum potassium will aggravate the myotonia. Children with this disorder may experience acute hypoventilation and coma after eating a meal rich in potassium, caused by myotonia of the thoracic muscles. Often these children are misdiagnosed as having a seizure disorder. Clearly, mutations of the sodium-chloride channels of muscle can result in several different clinical syndromes, including the systemic form found in myotonic dystrophy.
Metabolic myopathies
Muscle contraction requires energy in the form of adenosine triphosphate (ATP), which is provided from the metabolism of glycogen, glucose, and fatty acids. The metabolic pathways of all three converge into acetyl coenzyme A (acetyl-CoA), which is oxidized in the mitochondria through the Krebs cycle and respiratory chain to ATP ( Fig. 9-2 ). In metabolic myopathies, defects in this process are either defects of substrate use (involving glycogenosis) or disorders of lipid metabolism. Tsujino et al. report that patients with muscle substrate use disease present with two major clinical presentations: (1) acute, recurrent, reversible muscle dysfunction that manifest as exercise intolerance or myalgias, or (2) fixed, often progressive weakness. The disorders presenting as acute reversible muscle weakness can usually be differentiated into defects in glycogen or lipid metabolism based on their presentation. Because glycogen metabolism is important for intense aerobic exercise, patients with defects in glycogen metabolism experience muscle cramping and weakness after strenuous exercise, whereas patients with lipid metabolic defects often complain of muscle cramping or weakness after prolonged moderate exercise. Prolonged fasting can exacerbate these conditions and lead to respiratory muscle fatigue and myoglobinuria.

Metabolic myopathies of infancy or early childhood usually present as multisystem disorders. The “floppy infant syndrome” is the simplified clinical description for children with heterogeneous metabolic myopathies. These children are at risk for respiratory complications, because of diminished respiratory effort and cough reflex, and aspiration pneumonia. These metabolic defects are also likely to result in developmental CNS defects, cardiomyopathies, and cardiac conduction defects. The progressive atrophy of skeletal musculature will lead to contractures and scoliosis, which may require surgical correction.
Glycogen Storage Myopathies
The glycogen storage disorders are the result of muscle enzymatic defects in glycogenolytic or glycolytic pathways leading to the accumulation of glycogen. The myopathy is not caused by the accumulation of glycogen, however, but rather by the block in energy production, and thus substrate use diseases. The glycogen storage diseases were assigned Roman numerals in the order of their discovery and classified as “muscle diseases of glycogenosis” by Cori ( Fig. 9-3 ). This section discusses these myopathic nonlysosomal glycogenoses in their enzymatic sequential order.


Full access? Get Clinical Tree
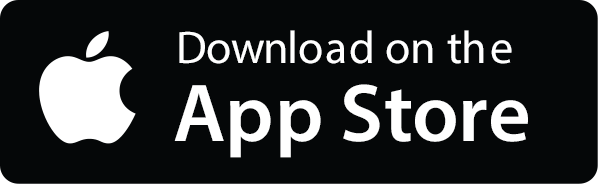
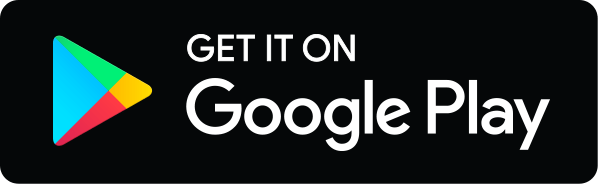
