Fig. 20.1
A schematic representation of the brain . The cortical area or cortex (shown in deep blue) is where conscious processes are integrated, and the subcortical areas (shown in red) are where a number of nonconscious processes such as implicit memory or nociception are integrated. The subcortical structures include the limbic system involved in emotional modulation, the thalamus which receives sensory information and relays them to the cortex, and also the medulla where the controls of blood pressure, heart rate, and respiration are located
Nociceptive information is transmitted through the spinal cord to the thalamus and then to the cortex. In awake subject, a number of responses are triggered: firstly the motor response, then the cardiovascular autonomic response with a possible emotional component, and finally a cognitive response. Anesthetics induce inhibition of these different processes, and this inhibition depends on both the agent and the dose
If the stimulus is greater than expected or the analgesia insufficient, a partial activation of the brain networks, may occur with firstly the reappearance of autonomic responses (pupils or cardiovascular reactivity), then a motor response is possible and finally a cortical awakening may be observed. The assessment of nociception is based on monitoring of these possible responses, especially the autonomic responses which are, up to now, the most investigated
Schematically, anesthetic agents induce in a dose-dependent manner a loss of consciousness (cortical inhibition), followed by a loss of motor response to nociceptive stimulation (spinal inhibition), and lastly a loss of autonomic response to nociception (subcortical inhibition) [2] (Fig. 20.2).
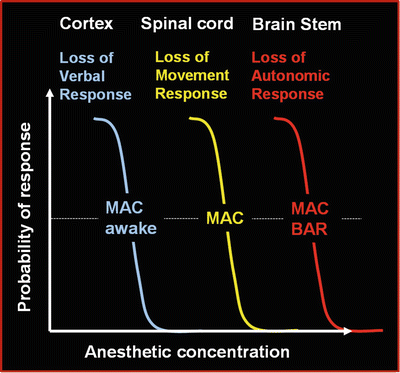
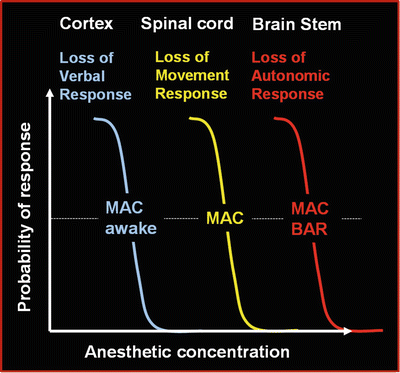
Fig. 20.2
Anesthetic agents induce in a dose-dependent manner a loss of consciousness (cortical inhibition ), followed by a loss of motor response to nociceptive stimulation (spinal inhibition), and lastly a loss of autonomic response to nociception (subcortical inhibition)
Nociceptive information is transmitted through the spinal cord to the thalamus and then to the cortex. In an awake subject, a number of responses are triggered: firstly, the motor response; then, the cardiovascular autonomic response with a possible emotional component; and, finally, a cognitive response. Anesthetics induce inhibition of these different processes, and this inhibition depends on both the agent and the dose. If the stimulus is greater than expected or the analgesia insufficient, a partial activation of the brain networks may occur with firstly the reappearance of autonomic responses (pupillary or cardiovascular reactivity), then a motor response is possible, and finally a cortical activation may be observed. The assessment of nociception is based on monitoring of these possible responses , especially the autonomic responses which are, up to now, the most investigated.
The EEG Gives Mainly Information About Cortical Inhibition and Loss of Consciousness
Up to now the concept of monitoring the depth of anesthesia is mainly based on EEG analysis, using devices providing automatically calculated indices which are supposed to vary with the hypnotic concentrations [3].
High doses of analgesic agents may cause a slowing of EEG with large amplitude waveforms and without burst suppression. These effects are only seen with high concentrations, well above those used for routine balanced anesthesia. In routinely used doses, these agents do not influence the EEG to a noticeable degree. The weakness of this effect is also dependent on the intensity of the painful stimulus (and on the balance between pain-related activation and analgesic related inhibition).
The value of the EEG or an EEG-derived parameter in predicting movement attributed to a painful stimulus under hypnotic anesthesia depends on the profile of the relationship between the anesthetic agent and the EEG parameter; thus, if the concentration being examined lies in the horizontal portion of the dose–response curve, the relationship will be weak, but if it lies in the steep part, the predictive value will be better. This explains why the BIS is more predictive during propofol infusion than under sevoflurane anesthesia . The motor response to each hypnotic agent, which is mediated by cortical and spinal mechanisms, is in proportion to the agent’s specific cortical, subcortical, and spinal actions (spinal effects of sevoflurane are more marked than for propofol) [4].
In a schematic sense, pain causes EEG activation in the deeply asleep patient, similar to a startle reaction, with a decrease in slow delta and augmentation of fast alpha and beta activity. This depends on the type of stimulus and on the degree of pain (articular > muscular > cutaneous) [5]. In the anesthetized patient, the same response is observed, similar to a startle reaction, and is diminished by increasing the depth of anesthesia or by increasing the dose of analgesic agent. This activation of rapid frequencies in response to pain (cortical awakening) is the basis of the decision algorithms that generate a higher BIS score and indicate a lack of analgesia .
In the same way, increase of EEG and EMG variability seems to be associated with the occurrence of intraoperative somatic events in anesthetized adults [6]. Some authors have proposed a composite variability index (CVI) derived from the standard deviations of the bispectral index and the electromyogram, to assess the level of analgesia /nociception during general anesthesia [7]. However, if the CVI appears to correlate with somatic responses to noxious stimuli, the unstimulated CVI depends more on the hypnotic drug effect than on opioid concentration [8].
The noxious stimulation response index (NSRI) is a newer index under development. It is a novel index, ranging between 100 and 0, computed from estimated hypnotic and opioid effect-site concentrations using a hierarchical interaction model [9]. The NSRI provides a prediction of the probability of a response to a standardized noxious stimulus (laryngoscopy ). However given the unreliability of currently available PKPD modeling data in children, this index will require specific validation in pediatric populations.
Based on cortical and subcortical recordings of EEG in patients undergoing neurosurgery, the study by Velly went further and deeper, providing important findings regarding the monitoring of depth of anesthesia. The authors have demonstrated that quantitative parameters derived from the cortical EEG but not from the subcortical EEG were able to predict consciousness versus unconsciousness. Conversely, quantitative parameters derived from the subcortical EEG but not from the cortical EEG were able to predict movement in response to laryngoscopy [10]. They conclude that in humans, unconsciousness mainly involves the cortical brain, but that suppression of movement in response to noxious stimuli is mediated through the effect of anesthetic agents on subcortical structures. These data support Kissin’s view that “the search for a reliable index of anesthetic depth” should be transformed into a search for separate indices of different components of anesthesia [11]. These conclusions suggest that besides monitoring of the hypnotic component of general anesthesia, the analgesic component should also be assessed, for instance, by using investigating and analyzing the subcortical effects of anesthesia .
Monitoring Nociception Requires Assessment of Subcortical Activity
The EEG-derived parameters can improve our ability to detect consciousness or unconsciousness. However the probability of movement in response to a noxious stimulus seems to be much more difficult to assess because it is under the control of brain structures not monitored by the EEG. Thus we need an index allowing the assessment of subcortical activity, and this activity may be approached at multiple levels, for instance, diencephalic, mesencephalic, and brain stem (Fig. 20.1). The best way might be to use subcortical electrodes, as in Velly’s study; however this invasive method obviously cannot be considered in routine clinical practice. Conversely, a simple (and old) way to assess the nociceptive response is to look at the motor response to a painful stimulus; however we know that the motor response may have disappeared while the autonomic response, including cardiovascular response, persists (Fig. 20.2). This persistence of autonomous responsiveness suggests that subcortical brain regions may not be completely inhibited, and this lack of inhibition might be responsible for a deleterious stress response or a nonconscious mnesic trace. On the other hand, the possibility of opioid-induced postoperative hyperalgesia should lead us to refine opioid administration by targeting a relevant clinical end point [12, 13].
Consequently monitoring of the nociception–antinociception balance in routine practice appears to be an important challenge for anesthesiologists [14].
During the last decade, a variety of monitoring systems were developed in order to assess the nociceptive balance. Up to now, all these devices are based on the assessment of the autonomic response to nociceptive stimulation. The emerging clinical devices include those which assess peripheral sympathetic response (skin conductance), cardiac and vascular sympathetic response (surgical pleth index ), parasympathetic cardiac response (analgesia /nociception index), and finally pupillometry which is based on the assessment of the pupillary reflex dilatation (PRD) induced by nociceptive stimuli.
Assessment of Peripheral Sympathetic Responses to Nociceptive Stimuli
Skin Conductance Measurement
The sudoral glands in the palms of the hands and the soles of the feet are exclusively innervated by sympathetic nervous fibers. Sympathetic stimulation generates an efflux of sweat at the surface of the skin. Sweat is mainly composed of salt and water; thus after a sympathetic stimulation, an electric current can be transmitted faster from one point to another at the surface of the skin, that reflects an increase of skin conductance. The interval between a sympathetic stimulation and an elevation of skin conductance is very short (approximately 2 s).
A noninvasive device based on these changes of skin conductance has been developed by the team of Hanne Storm, in Norway [15, 16]. Skin conductance fluctuations are recorded by three cutaneous electrodes attached to the palm or sole of the patient, and connected to a monitor.
This device intends to evaluate the intensity of nociception by measuring the intensity of the peripheral sympathetic activation through the changes of skin conductance.
This noninvasive device has been initially designed and tested in infants including neonates. The evolution of the values of skin conductance parameters during the first year of life was assessed by Hernes [17]: in full-term healthy children, skin conductance parameters increase during the first 10 weeks, then reach values close to those observed in adults. In neonatology units, the skin conductance monitor showed very interesting results: the value of skin conductance parameters increased during heel stick procedures in awake premature infants [15], but on the contrary, it did not increase during non-painful stimuli (such as changing and feeding) [18]. In infants, skin conductance is probably not influenced by hemodynamic variations, muscle relaxants, ventilation, or temperature (except in extreme conditions) [19]. Inter- and intraindividual variability was described as low in infants [20].
Used in 1–16-year-old children to assess postoperative pain, skin conductance fluctuations were correlated to standard clinical pain assessment tools with a good sensitivity and a moderate specificity [21]. Another study, performed in school-aged children after surgery, found that the number of fluctuations of skin conductance per second (NFSC) correlated weakly with numeric rating scale pain scores; the authors concluded that NFSC measurement was feasible in a perioperative setting but was not specific for postoperative pain intensity and was unable to identify analgesia requirements when compared with self-report measures [22]. In a prospective observational study, skin conductance was not found to be more sensitive or faster than a clinical scale for the assessment of pain or stress in critically ill children undergoing painful procedures [23].
Fewer data are available about skin conductance variations in anesthetized children. In sedated children under mechanical ventilation in intensive care units, skin conductance was the parameter that correlated best to COMFORT scale scores, compared to heart rate or blood pressure during endotracheal suctioning [24]. During the maintenance of inhaled desflurane anesthesia in children, at BIS 50, tetanic stimulations of 60 milliamps performed at different levels of remifentanil analgesia induced low-amplitude variations of skin conductance [25]. These mild variations were only observed at the lower infusion rates of remifentanil. Indeed, at this deep and constant level of hypnosis, with halogenated agents, the sympathetic nervous system activity is likely to be virtually abolished. And as sympathetic activity is the source of skin conductance variations, deep halogenated anesthesia might prevent the occurrence of significant skin conductance fluctuations for moderate nociceptive stimulations.
In adult patients, changes in skin conductance partially reflected changes in plasma noradrenaline levels and were not affected by a bolus of opioids [26]. Several studies from the team of Ledowski investigated the usefulness of the NFSC to assess post-operative pain. The authors found that NFSC were weakly correlated with postoperative VAS ratings [27–29]. Taken together, these results led to some questioning about the sensitivity and above all the specificity of this device used in the particular context of awake cooperating adults for whom self-evaluation of pain by means of a VAS remains the gold standard.
Regarding assessment of skin conductance in anesthetized adults, skin conductance parameters were correlated to the variations of catecholamine serum concentrations during intubation [19] and to a clinical score of stress [30]. At emergence [31] or during peroperative stimulations inducing a rise in the bispectral index above 50 [32], skin conductance parameters increased. The ability of skin conductance to predict clinical reactions or response to extubation, however, is still debated [31, 33, 34]. In critically ill adults , NFSC might be more useful at evaluating emotional distress than pain assessment alone [35].
Taken together, these findings suggest that skin conductance may provide a noninvasive and quick assessment of sympathetic activation induced by emotional stress or nociception, in children, and even in neonates, as well as in adults. Skin conductance measured in healthy subjects may provide a useful parametric prediction of pain for many experimental settings [36]. One of the major interests of this monitor is to propose an evaluation of nociception which does not imply an involvement of the patient: it might improve pain assessment in patients whose communication abilities are poor. However the relevance of this monitor might be weaker when the sympathetic activity is strongly inhibited, for instance, under general anesthesia .
Pulse Wave Amplitude Measurement Using Photoplethysmography
The activation of the peripheral sympathetic nervous system induces a distal vasoconstriction. The degree of vasoconstriction is determined by the intensity of the sympathetic stimulation. By measuring the absorption of light at the tip of the finger, the photoplethysmographic monitor intends to quantify this distal vasoconstriction and thus to evaluate the peripheral sympathetic nervous activity. Basically, the photoplethysmographic pulse wave amplitude (PPGA) is inversely correlated to the intensity of sympathetic activation [37].
This noninvasive monitor is routinely used in anesthesia, because the digital absorption of light also provides vital information about the patient’s arterial blood oxygenation (SpO2). In the operating room, the variability of the beat-to-beat amplitude of the plethysmographic signal is often masked by the auto-scaling processes of the monitor.
In the context of monitoring of analgesia , to increase the specificity of the index, photoplethysmography was associated to the measure of RR interval. Indeed, the changes in pulse wave amplitude provide information about the peripheral sympathetic vasomotor tone, while heart rate changes result from autonomic influences on the sinus node. These two parameters are normalized and processed in a unique algorithm that creates an index, scaled between 0 and 100: the surgical stress index (SSI) , later renamed surgical pleth index (SPI) , presented in 2007 by Huiku [38].
Although the performance of the SPI to evaluate postoperative pain in awake adults seems to be poor [39], many studies are in favor of its usefulness in the assessment of the peroperative balance between nociception and analgesia . In anesthetized adults, it has been demonstrated that the SSI increases after nociceptive stimulation [40, 41] and that it decreases in a dose-dependent manner after the administration of opioids [38, 42, 43]. During balanced anesthesia, the SSI correlated with the target concentration of remifentanil, but not with the concentration of hypnotics [42, 44]. Regarding regional anesthesia, the SSI does not increase if a nociceptive stimulation is applied in a site covered by a peripheral nerve block [45].
The SSI is the first monitor to have been tested as a pharmacodynamic assessment of the analgesia /nociception balance and used to guide the administration of remifentanil infusions: in adults under BIS-guided propofol TCI, compared to a “standard practice” group, SSI guidance for remifentanil (with SSI target 20–50) was associated with significantly decreased total remifentanil consumption of 30 % [46]. SSI also decreased the incidence of movement, and of peroperative major hemodynamic variations. This unexpected association between “less analgesic” and “less hypertension, less tachycardia, less movement” could be explained by a greater overall stability of the analgesia /nociception balance during the surgical procedure, thus providing a more stable level of hypnosis. Recovery and postoperative pain were similar in both groups. These important findings were later confirmed by a second study comparing SPI-guided remifentanil infusion to standard practice in adults anesthetized with entropy-guided propofol infusion for day-case surgery [47]. Again, SPI monitoring resulted in a reduced total consumption of remifentanil, but also of propofol. Postoperative pain and complications were similar in both groups. Finally, this last study showed a faster recovery and hence a better cost-effectiveness associated to the SPI guidance of remifentanil administration.
Interestingly, SSI has been shown to increase after noxious stimulations even in patients receiving β-blocking agents, when compared with those receiving an appropriate dose of fentanyl [48]. However, other factors known to influence autonomic reactions independently of a noxious stimulus might interfere with the accuracy of the SSI in evaluating the nociception–antinociception balance. Factors that may be relevant include intravascular volume status, diabetes, or chronic high arterial pressure and antihypertensive medications. Local vasoconstriction (hypothermia , severe hypovolemia ) or vasodilation (regional anesthesia) might also influence the value of the SSI . Lastly, in fully awake patients under spinal anesthesia, the SSI does not reflect the nociception–antinociception balance [49].
In children, however, very few studies have focused on the interest of the SSI in the assessment of peroperative nociception. As in adults, the SSI increased significantly during nociceptive stimulation in 22 patients aged 4–17 undergoing strabismus surgery under sevoflurane [50]. Recently the potential interest of the SPI was investigated in children from 3 to 10 years, scheduled for adenotonsillectomy. The authors found that SPI-guided analgesia reduced opioid consumption during surgery compared with conventional analgesia practices, whereas it also led to more frequent postoperative hypertension and higher emergence agitation and pain scores in the recovery room; they concluded that SPI was inadequate to guide preoperative analgesia in children [51].
Up to now, no study on the SSI included a patient younger than 3 years. In neonates or infants, because of the very high physiological levels of heart rate at rest, the reliability of the SSI still requires investigations.
Assessment of Cardiac Parasympathetic Responses to Nociceptive Stimuli
The autonomic response to noxious stimulation can be investigated at the cardiac level, by studying heart rate variability. Basically heart rate variability results from sympathetic and parasympathetic modulation of the sinus node. When the sympathetic influence increases, the parasympathetic influence decreases and vice versa. This autonomic equilibrium is classically called the sympathovagal balance . Noxious stimulations induce changes in this balance with a shift toward the sympathetic activity associated with a decrease of the parasympathetic influence.
When HR is continuously recorded beat-to-beat, regular oscillations are found. Among these constitutive rhythms, it can be interesting to focus on the respiratory oscillations of HR. Indeed the latter result from changes in the parasympathetic drive exerted on the sinus node in response to respiratory movements (Fig. 20.3). Consequently quantification of these respiratory oscillations of HR allows an indirect assessment of cardiac parasympathetic modulation [52].
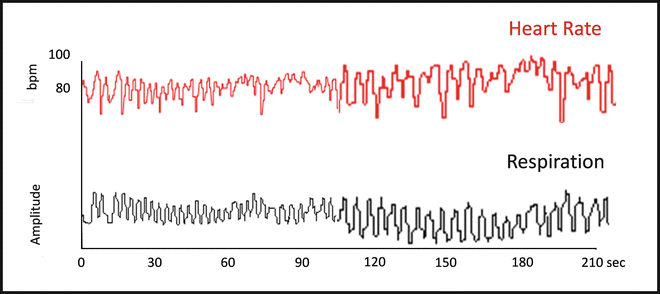
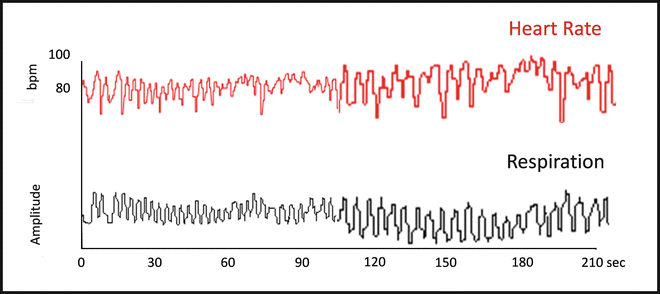
Fig. 20.3
Beat-to-beat continuous recording of heart rate (in red) and respiratory movements (in black). Respiratory oscillations of HR are generated by the reflex modulation of cardiac parasympathetic activity, due to the distension of pulmonary stretch receptors. Indeed, at the end of inspiration, there is an increase of HR due to PS inhibition, followed by a slowing down during expiration due to the comeback of the vagal control on the sinus node. This phenomenon is called respiratory sinus arrhythmia (RSA) . The quantification of RSA allows parasympathetic assessment
Using continuous recordings of HR in anesthetized adults, it has been observed that the respiratory sinus arrhythmia pattern changed when a surgical stimulation was associated with clinical signs of insufficient analgesia , even though the patient was not conscious [53, 54]. A nociception/analgesia algorithm based on the magnitude of the respiratory fluctuations in the RR series was developed and tested in adults anesthetized with propofol [55]. The parameters computed from this algorithm were recorded, and the calculated index was found to be related to pain/analgesia and relatively independent from other anesthesia-related events like hypnosis and hemodynamic conditions.
Therefore the analgesia /nociception index (ANI) was designed to give an online index calculated from the ECG signal, providing a quantification of the respiratory variability of heart rate. This index is supposed to reflect the cardiac parasympathetic activity and thus to decrease in response to a nociceptive stimulation under general anesthesia [56]. Its accuracy for predicting analgesia/nociception balance is still under investigation. Previous studies, carried out to validate the device, have suggested that in anesthetized adults, the ANI seems more sensitive than HR and SBP to moderate nociceptive stimuli in propofol anesthetized patients [57, 58]. The ANI could be also used to assess the parasympathetic tone in various clinical conditions, for instance, it might be a useful indicator of parasympathetic tone changes in emotional situations [59, 60].
In a recent study, the ability of the ANI was compared with that of the SPI to detect standardized noxious stimulation during propofol–remifentanil anesthesia. The authors demonstrated that ANI and SPI may improve detection but not prediction of a possible inadequate nociception–antinociception balance [61]. The same conclusions were found in adults anesthetized with sevoflurane [41, 62]. Regarding prediction of movement during laryngoscopy, the performance of the ANI might be interesting [63]. Interestingly, low doses of ketamine administered during standardized sevoflurane anesthesia did not influence the ANI reactivity to nociceptive stimulation [64]. Regarding postoperative pain assessment, the value of the ANI is still being debated: Boselli et al. demonstrated in adults that a measurement of ANI during the immediate postoperative period after general anesthesia was significantly correlated with pain intensity on arrival in the postanesthesia care unit [65, 66]. However on the other hand, Ledowski concluded that ANI did not reflect different states of acute postoperative pain measured on a NRS scale after adult sevoflurane-based general anesthesia [67]. Therefore further studies are required to conclude on the value of the ANI in awake subjects.
Recently, a prospective randomized study performed in adults failed to show any advantages of ANI-guided morphine administration in elective laparoscopic cholecystectomy , compared to the current standard of care [68]. This nevertheless highlights the difficulties in designing such studies; indeed the results depend on both the targeted threshold of the ANI number and the administrated opioid.
This noninvasive monitor might be useful in anesthetized children . For example, it could be used under general anesthesia to assess the sensory blockade induced by regional anesthesia [69]. In anesthetized children receiving different remifentanil infusion rates, the ANI provided a more sensitive assessment of nociception than hemodynamic parameters or skin conductance [25]. The current ANI was not designed for analyzing high respiratory frequencies and high heart rate level and so was not adapted to pain assessment in infants. However a further approach was developed to assess high-frequency variability of heart rate. The algorithm was adapted to the typical physiological parameters of the neonate. Indeed in full-term newborn infants, postoperative pain seems to be associated with a decrease in high-frequency heart rate variability. These findings suggest that assessment of HRV could provide a useful indicator to assess prolonged pain in newborn infants [70, 71]. Another new version of the ANI monitor dedicated specifically to assess well-being in neonates is in progress [70, 72].
The main advantage of this monitor is its usability. If the concept is physiologically attractive, its reliability under different anesthetic agents and conditions interacting with the autonomic nervous system activity should be investigated. Indeed parasympathetic activity could be decreased by stress (including nociceptive stress), anxiety, and all the factors or drugs which are known to increase the sympathetic activity. Consequently ANI may not be considered as a specific and robust measure for assessment of pain intensity [73].

Full access? Get Clinical Tree
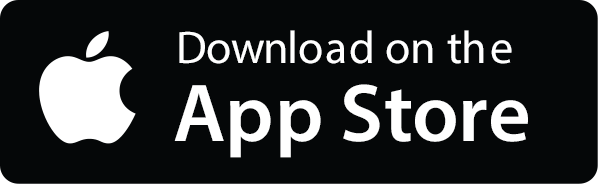
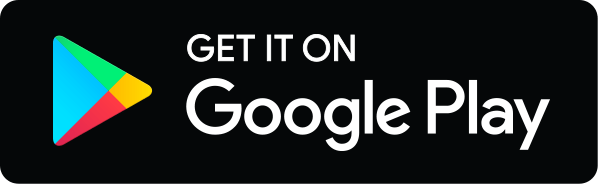