Monitoring and Maintaining a Patent Airway
Elizabeth H. Sinz
Kane High
The major general objectives of respiratory support in emergency cardiovascular care (ECC) are to provide a patent airway and adequate oxygenation and ventilation. These objectives require prioritization according to patient condition and potential precipitating cause.
Assessing the need for oxygenation and ventilation
Determinants of adequate respiration at the cellular level
Pearls and pitfalls of oximetry and capnometry
Principles of Oxygenation and Ventilation
The major general objectives of respiratory support in ECC are to provide a patent airway and adequate oxygenation and ventilation. These objectives require prioritization according to patient condition and potential precipitating cause. The major objectives of respiratory support can be organized into initial primary, or basic life support (BLS), priorities and secondary, or advanced cardiac life support (ACLS), priorities. Methods of maintaining the airway and administering oxygen are predicated upon clinical circumstances and may change with time; therefore, serial repeated evaluation of the airway and patient is critical.
The objectives of airway intervention and assessment are as follows:
BLS Primary A—Airway
Ensure a patent and protected airway; use manual techniques as needed.
Ensure a patent and protected airway; use simple, noninvasive airway adjuncts as needed.
BLS Primary B—Breathing
Provide supplementary oxygen.
Monitor the quality of oxygenation and ventilation with noninvasive devices.
Provide positive-pressure oxygenation and ventilation with manual techniques or noninvasive airway devices when spontaneous breathing is inadequate or absent.
ACLS Secondary A—Airway
Establish a patent and protected airway with invasive advanced airway devices.
Confirm proper placement of these devices with both clinical and device confirmation techniques.
ACLS Secondary B—Breathing
Provide effective positive-pressure oxygenation and ventilation through properly inserted advanced airway devices.
Secure the advanced airway devices to prevent displacement.
Monitor oxygenation and ventilation and tailor support as needed.
Cardiac or Respiratory Arrest?
Professional as well as lay-rescuers may be unable to accurately determine the presence or absence of adequate or normal breathing in unresponsive patients32,33,34 because the airway is not open35 or the patient has occasional agonal gasps, which can occur in the first minutes after SCA and may be confused with adequate breathing. Agonal gasps are not effective breaths.29 The patient who has agonal gasps should be treated as if he or she were not breathing by giving rescue breaths. CPR training should emphasize how to recognize agonal gasps and should instruct rescuers to give rescue breaths and proceed with the steps of CPR when the unresponsive patient demonstrates agonal gasps.
Initial Airway Sequence to Determine Adequate Breathing
Health care providers should quickly determine if the patient demonstrates spontaneous breathing efforts.
Is the patient making spontaneous breathing efforts?
Do these efforts appear adequate?
Can you feel the patient exhale into your hand?
If the efforts are inadequate, are the inadequate efforts caused by fatigue or one of the many causes of respiratory depression?
If the patient is making spontaneous breathing efforts, is there evidence of partial or complete upper airway obstruction caused by foreign material such as food, vomitus, or blood clots, or posterior displacement of the tongue or epiglottis?
If spontaneous breathing is absent or inadequate, provide positive-pressure ventilation with one of the following ventilation techniques described in this chapter:
–Mouth-to-mouth
–Mouth-to-mask
–Ventilation with a bag through a face mask, endotracheal tube, esophageal–tracheal Combitube, or laryngeal mask airway (LMA).
Clues to the Presence of Airway Obstruction
Significant partial upper airway obstruction typically causes noisy airflow during inspiration (stridor or “crowing”) and cyanosis (late sign). Another sign of airway obstruction is use of accessory muscles, indicated by retractions of the suprasternal, supraclavicular, and intercostal spaces. Complete airway obstruction results in patient efforts at breathing against a closed airway this typically causes a rocking or “see-saw” motion of the chest and abdomen as the diaphragm moves into the abdomen, pushing the abdomen upward and the chest collapses in the absence of airflow to fill the lungs. If the patient is not making spontaneous breathing efforts, airway obstruction becomes more difficult to recognize and must be determined during attempts to provide positive-pressure ventilation. Occasionally, isolated bradycardia, secondary to occult hypoxemia, provides an early sign of airway obstruction. It is important to recognize the presence of airway obstruction caused by the tongue and epiglottis, as this is common in the unconscious patient due to loss of muscle tone.
Assessing the Need for Supplementary Oxygen and Ventilation
Health-care providers must be able to accurately assess oxygenation and ventilation to detect and treat respiratory distress and failure. Clinical parameters form the cornerstone of initial assessment and intervention. Frequently, pulse oximetry and capnography provide useful supplemental and continuous information. The two major functions of respiration are to achieve and maintain:
Oxygenation (oxygenate arterial blood)
–Evaluate oxygenation with pulse oximetry
Ventilation (remove carbon dioxide from venous blood)
–Evaluate ventilation with capnography
Oximetry: Basic Principles
To understand the clinical utility of oximetry—as well as its limitations and pitfalls—one must understand the physics involved in measurement and the clinical principles of adequate tissue oxygenation. Here too, “treat the patient and not the number” is most important.
Basic Physics of Pulse Oximetry
The concentration of a substance in a fluid can be determined by its ability to transmit light. Oxygenated hemoglobin absorbs and reflects red and infrared light differently than nonoxygenated hemoglobin. Oxygenated hemoglobin primarily absorbs infrared light; reduced (nonoxygenated) hemoglobin primarily absorbs red light. In pulse oximetry, red and infrared light are passed through a pulsatile tissue bed, and a photodetector captures any nonabsorbed light on the other side of the tissue bed. A microprocessor calculates the relative absorption of red and infrared light that occurred as it passed through the tissue bed and can determine the percentage of oxygenated and nonoxygenated hemoglobin present in that tissue bed. In this way the pulse oximeters calculate the percent of hemoglobin that is saturated with oxygen (percent saturation, percent SO2; percent SaO2). In the absence of abnormal hemoglobins, such as carboxyhemoglobin or methemoglobin, if the arterial oxygen saturation is greater than 70%, there should be no more than 3% variance between pulse oximetry and the arterial oxyhemoglobin saturation measured by co-oximeter used in arterial blood gas determinations. Studies have shown pulse oximetry to be an accurate and useful guide for patient care in the in-hospital setting as well as in the prehospital EMS setting,36,37,38,39 including transport by rotary-wing aircraft.40
Hemoglobin and Tissue Oxygen Delivery
Analysis of arterial blood is an invasive procedure, and arterial blood gases are infrequently ordered, available, or clinically useful during cardiac arrest. Pulse oximetry was developed to provide a noninvasive, painless approximation of the percent of hemoglobin saturated with oxygen, usually designated as SpO2 to distinguish it from the value measured by co-oximetry of an arterial blood sample (SaO2). In cardiac arrest, the pulse oximeter will not function well because there must be pulsatile blood flow through the tissue where the light measurements are being made to obtain an accurate measurement although a reading may appear with adequate chest compressions. But pulse oximetry is commonly used for monitoring patients who are not in arrest, because it provides a simple, continuous method of tracking oxyhemoglobin saturation. There are three major determinants of adequate tissue oxygenation:
There must be an adequate amount of oxygen to saturate the hemoglobin molecules.
There must be enough hemoglobin to carry adequate oxygen.
Cardiac output must be sufficient to deliver the saturated hemoglobin to peripheral tissues.
Arterial oxygen content is determined by the hemoglobin concentration and its saturation with oxygen. Normal pulse oximetry saturation, however, does not ensure adequate systemic oxygen delivery because it does not calculate the total
oxygen content (O2 bound to hemoglobin + dissolved O2) and adequacy of blood flow (cardiac output):
oxygen content (O2 bound to hemoglobin + dissolved O2) and adequacy of blood flow (cardiac output):
Arterial oxygen content (mL of oxygen per dL of blood)
= Hemoglobin concentration (g/dL)
× 1.34 (mL oxygen/g hemoglobin)
× Oxyhemoglobin saturation
+ (PaO2 × 0.003)
(normal oxygen content = 18-20 ml/dL)
Measurement of the oxygen content is only part of the determination of effective oxygenation. The oxygen present must be delivered to the tissues for use. Peripheral cells must be able to uptake and use oxygen for cellular metabolism. Oxygen delivery is defined by how much oxygen is present and how much of it is delivered to the body. Oxygen delivery is defined by the following equation:
Oxygen delivery (mL of oxygen/min)
= arterial oxygen content (mL oxygen/dL blood)
× cardiac output (L/min)
(× 10 dL/L)
Pulse Oximetry Precautions and Limitations
Pulse oximetry readings, even those that are accurate, do not necessarily correlate with cardiac output and oxygen delivery. In clinically evaluating a patient’s cardiac output and oxygen delivery, always assess systemic perfusion and be aware of the hemoglobin concentration. If cardiac output or hemoglobin concentration is low, oxygen delivery can be inadequate even if oxyhemoglobin saturation is normal.
Abnormal Hemoglobins (e.g., Carbon Dioxide Poisoning)
The light absorption of carboxyhemoglobin (carbon monoxide) and of methemoglobin (nitrates or aromatic amines) differs from that of normal hemoglobin, so carboxyhemoglobin and methemoglobin are not recognized by pulse oximeters. If these altered forms of hemoglobin are present, the SaO2 calculated by the pulse oximeters will be falsely high because most pulse oximeters calculate the percent of normal hemoglobin that is saturated with oxygen rather than the percent of total hemoglobin that is saturated with oxygen. When you suspect the presence of carbon monoxide poisoning or methemoglobin toxicity, you should measure the oxyhemoglobin saturation with a co-oximeter (this requires arterial blood sampling).
Abnormal Conditions Affecting Pulse Oximetry
In several clinical situations, abnormal or inaccurate oximetry readings may occur. In a large review of pulse oximeter devices, the major sources of error were finger thickness, hemoglobin level, skin color, and peripheral temperature.
Motion artifact and low perfusion are the most common sources of SpO2 inaccuracies. Motion artifacts can occur with patient transport, movement, twitching, and agitation.
When the systolic blood pressure is low, oximetry becomes inaccurate because of decreased pulsatile flow, response time, or calibration characteristics of the instrument. Oximetry may also read falsely high when the SaO2 is <70% or may become inaccurate when the hemoglobin level is very low (2–3 g/dL).
Peripheral hypoperfusion from hypothermia, low cardiac output, or vasoconstrictive drugs may cause or increase inaccuracies.
Very dark skin, fingernail polish, and fungal infections of the nails (onychomycosis) may cause spurious readings when digital monitors are used.
Accuracy of Pulse Oximeters in the Presence of Carboxyhemoglobinemia and Methemoglobinemia
Most commercially available pulse oximeters use two light-emitting diodes and a photodetector in a sensor. The two diodes emit a red and an infrared light. Oxygenated hemoglobin in the pulsatile tissue bed primarily absorbs infrared light, but reduced (nonoxygenated) hemoglobin in the pulsatile tissue bed primarily absorbs red light. A microprocessor in the unit determines the relative absorption of red and infrared light to calculate the percentage of oxygenated versus reduced (nonoxygenated) hemoglobin present in the tissue bed.
The light absorption of methemoglobin and carboxyhemoglobin is different from that of normal hemoglobin, so pulse oximeters will not accurately reflect total hemoglobin saturation in the presence of these two products.41 With significant methemoglobinemia, pulse oximeters display an oxygen saturation of approximately 85%. With significant carboxyhemoglobinemia (as occurs in carbon monoxide poisoning), the pulse oximeter will typically reflect the oxygen saturation of normal hemoglobin, not the percentage of hemoglobin bound to carbon monoxide. If these or other conditions affecting oxyhemoglobin saturation are present, arterial hemoglobin oxygen saturation must be determined by using co-oximetry.
Safety Considerations
There are some safety precautions regarding use of the pulse oximetry equipment:
Do not use oximetry probes with broken or cracked casings. Burns have been reported when the lights from the probes come into direct contact with the skin.
Do not connect the probes from one manufacturer to base units made by another manufacturer.
Capnography and Capnometry
Detection of exhaled CO2 is one of several independent methods of confirming endotracheal tube position. Given the simplicity of the exhaled CO2 detector (waveform, colorimetry, or digital), it can be used as the initial method for
detecting correct tube placement even in the patient in cardiac arrest. Detection of exhaled CO2, however, is not infallible as a means of confirming tube placement, particularly during cardiac arrest. Evidence from a meta-analysis in adults42 indicated a range of results:
detecting correct tube placement even in the patient in cardiac arrest. Detection of exhaled CO2, however, is not infallible as a means of confirming tube placement, particularly during cardiac arrest. Evidence from a meta-analysis in adults42 indicated a range of results:
Positive predictive value (probability of endotracheal placement if CO2 is detected): 100%
Negative predictive value (probability of esophageal placement if no CO2 is detected): 20% to 100%
Therefore, exhaled carbon dioxide almost certainly indicates that ventilation of the lungs is occuring; however absence of exhaled carbon dioxide may be due to esophageal intubation or to cardic arrest with no pefusion of the lungs. The use of CO2 detecting devices to determine the correct placement of other advanced airways (e.g., Combitube, LMA) has not been adequately studied.
Basic Principles
The body eliminates carbon dioxide through ventilation. When blood passes through the lungs, carbon dioxide moves from the blood, across the alveolar capillary membrane into the alveoli and then into the airways and is exhaled. Alveolar PCO2 should be approximately equal to pulmonary venous, left atrial and arterial PCO2. If there is a good match of ventilation and perfusion in the lungs and there is no airway obstruction, exhaled CO2 should correlate well with arterial PCO2, and exhaled carbon dioxide can be used to estimate arterial carbon dioxide tension. Carbon dioxide can be detected by either of two techniques:
Capnometry is a qualitative method that detects the presence of CO2 in exhaled air. Colorimetric capnometers are semiquantitative devices based on a chemical reaction between exhaled CO2 and a chemical detector impregnated in a strip of paper. These devices are used to identify the presence or absence of a sufficient quantity of CO2 to produce a color change at a point in time.
Capnography devices are quantitative devices that measure the concentration of CO2 using infrared absorption detectors. Carbon dioxide concentration is typically displayed by these devices as a continuous exhaled CO2 concentration waveform with a digital display of end-tidal CO2. This plot of CO2 concentration against time is called a capnograph.
Capnometry
A CO2 concentration of >2% will react with the chemical reagent in a colorimetric CO2 detector and change the color (Fig. 18-1). If the endotracheal tube is actually in the trachea, the CO2 detector will turn a color determined by the manufacturer. In the absence of expired CO2, the color of the colorimetric indicator will remain unchanged. Health care providers commonly use this color change with a disposable device as a quick check to provide one method of device confirmation of the success or failure of endotracheal intubation.43
Color Change with Colorimetric Device: Know Your Device
The 2000 Guidelines for Advanced Cardiovascular Life Support (ACLS) and Pediatric Advanced Life Support (PALS) as well as associated training materials offer examples of how colorimetric CO2 detection devices work and suggest mnemonic schemes for interpretation. Those details were meant to help providers remember the significance of specific colors indicating the relative concentration of CO2 present in the expired air. Some of the materials, for example, link the color purple with a sign of low CO2 and yellow with high CO2. New devices have been introduced on the market with new indicators and color schemes. The indicators in some commercially available devices respond to detection of increasing CO2 concentration by transitioning in color using one of the following schemes:
Purple-to-yellow
Blue-to-yellow
White-to-purple
Even more possibilities will likely appear on the market in the coming years. Clearly, any one mnemonic that relies on color will not fit all available devices.
The product inserts for some commercially available semiqualitative colorimetric capnometers recommend that after intubation, six positive-pressure breaths be provided by hand or mechanical ventilation before attempting to identify exhaled CO2. Six breaths will wash out any CO2 that is present in the stomach or esophagus from bag-mask ventilation. Any CO2 detected after six breaths can be presumed to be from the lungs.44,45 There is no need to continue ventilation attempts if the endotracheal tube is clearly misplaced.
In patients who weigh >2 kg with a perfusing rhythm (not in cardiac arrest), the sensitivity and specificity of colorimetric capnometry methods approaches 100% if six ventilations have been provided following intubation. This means that if the endotracheal tube is in the trachea of a patient with a perfusing rhythm, the colorimetric device will change color with few exceptions (see “False-Positive Results,” below). If the tube is in the esophagus, there should be no CO2 detected after six breaths, so a colorimetric CO2 detector should remain unchanged.
False-Positive Results
When exhaled CO2 is detected (positive reading for CO2) in cardiac arrest, it is usually a reliable indicator of tube position in the trachea. False-positive readings (CO2 is detected but the tube is located in the esophagus) have been observed in animals that ingested large amounts of carbonated liquids before the arrest.44
A color change is generally a reliable indicator of the presence of CO2 and endotracheal intubation. False-positive color changes are uncommon but can occur when the tip of the tube is in the supraglottic area rather than in the trachea. A false-positive may also be possible following prolonged
bag-mask ventilation,8,9,10 which is why some manufacturers recommend that six ventilations be provided after intubation and before the check for exhaled CO2. Finally, if the colorimetric detector is contaminated with acidic gastric contents or acidic drugs, such as endotracheal administration of epinephrine, a colorimetric detector may remain unchanged during the entire respiratory cycle.
bag-mask ventilation,8,9,10 which is why some manufacturers recommend that six ventilations be provided after intubation and before the check for exhaled CO2. Finally, if the colorimetric detector is contaminated with acidic gastric contents or acidic drugs, such as endotracheal administration of epinephrine, a colorimetric detector may remain unchanged during the entire respiratory cycle.
False-Negative Results
False-negative results occur if the tube is in the trachea but the colorimetric indicator remains unchanged. A false-negative result is most often associated with cardiac arrest (see below). False-negative results may also occur with severe airway obstruction or pulmonary edema, which can impair CO2 elimination so that inadequate CO2 is detected in exhaled gas. Administration of an intravenous (IV) bolus of epinephrine in patients with cardiac arrest or very low cardiac output may transiently reduce pulmonary blood flow and reduce exhaled CO2.46
False-negative readings (in this context defined as failure to detect CO2 despite tube placement in the trachea) may be present during cardiac arrest for several reasons. The most common explanation for false-negative readings during CPR is that blood flow and delivery of CO2 to the lungs are low. False-negative results have also been reported in association with pulmonary embolus because pulmonary blood flow is reduced. In addition, elimination and detection of CO2 can be drastically reduced with severe airway obstruction (e.g., status asthmaticus) and pulmonary edema.47 For these reasons, if CO2 is not detected, the AHA 2005 guidelines recommend that a second method be used to confirm endotracheal tube placement, such as auscultation of the lungs, direct visualization of the tube passing through the vocal cords with a laryngoscope, or the esophageal detector device.
Capnography
Some capnography devices are infrared devices in which a light-emitting diode is used to measure the intensity of light transmitted across a short distance, usually the diameter of an endotracheal tube. The measured light absorption varies inversely with the concentration of CO2 passing through the endotracheal tube. When attached to the end of an endotracheal tube, these infrared devices are called mainstream capnometers. These devices readily reveal low exhaled CO2 indicative of esophageal intubation, and they can provide estimates of the adequacy of ventilation and the effectiveness of circulation during CPR. Conversely, sidestream capnometers draw a small sample of gas from the airway through a small tube to measure the CO2 concentration in a device detached from the airway and the patient.
Capnography devices provide a continuous readout of the concentration of CO2. They are used to monitor the quality of ventilation in nonarrest patients. Because of their high degree of sensitivity to expired CO2, however, capnographs can often detect a sufficient quantity of CO2 to indicate the presence of an endotracheal tube in the trachea even when cardiac arrest is present. Continuous capnography monitoring devices can identify and signal a fall in exhaled CO2 consistent with endotracheal tube dislodgment. This may be very helpful when patients are being moved or during emergencies.
Capnometry and Capnography in Cardiac Arrest
End-tidal CO2 monitoring is a safe and effective noninvasive indicator of cardiac output during CPR capnography may be an early indicator of return of spontaneous circulation (ROSC) in intubated patients because an increase in exhaled CO2 typically occurs when cardiac output increases and perfusion of the lungs improves. During cardiac arrest, CO2 continues to be generated throughout the body; however, there is little pulmonary blood flow, and the level of CO2 in exhaled gas may be too low to produce a color change or a graphic exhaled CO2 waveform. In such situations, when a colorimetric device is attached to the distal end of a properly placed endotracheal tube, the color remains unchanged or the CO2 level remains very low. Because the unchanged color and lack of exhaled CO2 may also indicate that the tube has been placed in the esophagus, the health care provider must decide whether the lack of carbon dioxide indicates esophageal intubation or reflects the absence of blood flow to the lungs. The major determinant of CO2 excretion is its rate of delivery from the peripheral production sites to the lungs. In the low-flow state during CPR, ventilation is relatively high compared with blood flow, so the end-tidal CO2 concentration is low. If ventilation is reasonably constant, then changes in end-tidal CO2 concentration reflect changes in cardiac output. Patients who are successfully resuscitated from cardiac arrest have significantly higher end-tidal CO2 levels than those who cannot be resuscitated.
References
1. Kern KB, Hilwig RW, Berg RA, et al. Importance of continuous chest compressions during cardiopulmonary resuscitation: improved outcome during a simulated single lay-rescuer scenario. Circulation 2002;105(5):645–649.
2. von Planta M, Weil MH, Gazmuri RJ, et al. Myocardial acidosis associated with CO2 production during cardiac arrest and resuscitation. Circulation 1989;80(3):684–692.
3. Yannopoulos D, McKnite S, Aufderheide TP, et al. Effects of incomplete chest wall decompression during cardiopulmonary resuscitation on coronary and cerebral perfusion pressures in a porcine model of cardiac arrest. Resuscitation 2005;64(3):363–372.
4. Aufderheide TP, Pirrallo RG, Yannopoulos D, et al. Incomplete chest wall decompression: A clinical evaluation of CPR performance by trained laypersons and an assessment of alternative manual chest compression–decompression techniques. Resuscitation 2006;71(3):341–351.
5. Yannopoulos D, Aufderheide TP, McKnite S, et al. Hemodynamic and respiratory effects of negative tracheal pressure during CPR in pigs. Resuscitation 2006;69(3):487–494.
6. Baskett P, Nolan J, Parr M. Tidal volumes which are perceived to be adequate for resuscitation. Resuscitation 1996;31(3):231–234.
7. Aufderheide TP, Sigurdsson G, Pirrallo RG, et al. Hyperventilation-induced hypotension during cardiopulmonary resuscitation. Circulation 2004;109(16):1960–1965.
8. Paradis NA, Martin GB, Goetting MG, et al. Simultaneous aortic, jugular bulb, and right atrial pressures during cardiopulmonary resuscitation in humans. Insights into mechanisms. Circulation 1989;80(2):361–368.
9. Idris AH, Staples ED, O’Brien DJ, et al. Effect of ventilation on acid-base balance and oxygenation in low blood-flow states. Crit Care Med 1994;22(11):1827–1834.

Full access? Get Clinical Tree
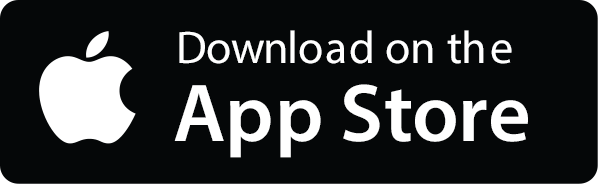
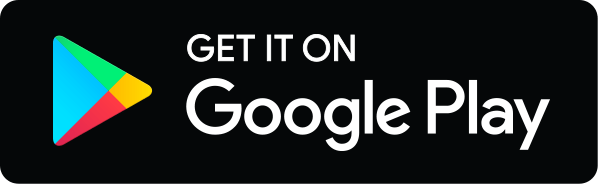