(1)
Botulinum Toxin Treatment Program, Yale School of Medicine, New Haven, CT, USA
Abstract
Botulinum neurotoxins (BoNTs) are now widely used in clinical medicine for treatment of a variety of hyperactive movement disorders, autonomic dysfunctions (sialorrhea and hyperhidrosis), spasticity, and chronic migraine. Further indications in other pain disorders are being actively explored based on animal studies and laboratory data indicating an analgesic effect for BoNTs through diverse mechanisms.
This chapter provides an overview of the molecular structure, pharmacological characteristics, mode of action, immunology, and side effects of botulinum neurotoxins.
Keywords
Botulinum toxinBotulinum neurotoxinOnabotulinumtoxinA (onaA)AbobotulinumtoxinA (aboA)IncobotulinumtoxinA (incoA)RimabotulinumtoxinB (rimaB)Introduction
The first detailed description of clinical symptoms related to botulinum neurotoxins was published by Justinus Kerner M.D. after an outbreak of food poisoning in the years 1817–1822 resulting from the consumption of rotten sausage in southern Germany (Erbguth 2004). Kerner also predicted the future therapeutic potential of the “poison” based on its muscle weakening effect. In 1895, a Belgian bacteriologist Emile van Ermengem from the University of Ghent isolated the gram-positive rods of botulinum toxin bacilli from smoked ham. He called the responsible agent bacillus botulinum. American investigator Lamanna et al. (1946) at the US Army’s Fort Detrick facility developed techniques for the concentration/crystallization of toxins which helped Edward Schantz from the University of Wisconsin to produce the first batch of the BoNT. Allan Scott, an ophthalmologist who had worked during the 1960s and 1970s on the paralyzing effects of BoNTs, published the weakening effect of BoNT-A on extraocular muscles of primates (Scott et al. 1973) and subsequently received permission from the FDA to conduct the first study on the potential of BoNT-A for strabismus in human subjects (1978). The FDA’s approval of BoNT-A for the treatment of strabismus in 1989 opened the door for further human research and discoveries.
Over the past 30 years, a variety of indications for the use of BoNTs emerged based on the muscle weakening effect of the toxin following intramuscular injection. Currently, major clinical indications include treatment of focal and segmental dystonias (blepharospasm, cervical dystonia, focal limb, and task-specific dystonias) as well as spasticity and cosmetic indications (Jankovic et al. 2008), mainly based on the inhibitory effect of the toxin upon acetylcholine release from the presynaptic vesicles. The inhibition of acetylcholine release from cholinergic postganglionic nerve endings, which innervate sweat and salivary glands, provided an effective treatment for sialorrhea and hyperhidrosis (Lakraj et al. 2013a, b).
In the field of pain, early observations in cervical dystonia suggested an analgesic effect for onabotulinumtoxinA (onaA) since the associated neck pain often improved earlier and independent of the improvement of neck posture (Jankovic and Schwartz 1990). Over the past 15 years, animal observations and human studies have illustrated a variety of mechanisms through which BoNTs can influence pain mediators. By the turn of the century, the pain indications of BoNTs had begun to be explored actively in human subjects. These efforts led to the recent approval of onabotulinumtoxinA for the treatment of chronic migraine in Europe and the USA (2010).
Structure and Mode of Action
Clostridium botulinum is a gram-positive anaerobic bacillus that produces the botulinum neurotoxin (BoNT). There are seven serotypes of BoNTs designated as A, B, C, D, E, F, and G. Each serotype has a distinct molecular structure, but there is significant homology between different toxins and between the structure of BoNT and tetanus toxin (Atassi 2004). Only serotypes A and B are currently used in clinical practice. Serotype F has a shorter duration of action and faster recovery, hence presenting a potential for future use in certain clinical conditions.
The neurotoxin complex is composed of BoNT itself (core toxin) with a molecular weight of 150 KD and a nontoxic protein complex that protects the toxin when exposed to deactivating factors (stomach acid, high temperature, and proteases) (Fig. 1.1). The nontoxic protein complex (NAPS) consists of hemagglutinin proteins (HA proteins) and non-HA proteins. The non-hemagglutinin protein fraction includes specific antigenic proteins which are the source of antibody formation against the toxin after BoNT therapy. The core toxin is composed of a heavy chain (HC) of 100 KD and a light chain (LC) of 50 KD linked by a single disulfide bond. The HC provides the toxin’s binding and translocation domain and LC its catalytic domain. Within minutes after intramuscular injection, the core toxin dissociates from the protein complex (Pickett 2009) and the molecule is activated (nicked). The type A toxin activation (nicking) takes place through its own protease (Simpson 2013), while for other toxin serotypes activation occurs through tissue proteases. The heavy chain has two distinct terminals Hc and Hn. Through Hc, the heavy chain of the toxin binds the core toxin complex to the specific cell membrane receptor made of ganglioside and possibly other materials (Swaminathan 2011). The membrane receptor sites are different for type A and type B toxins. The receptor is “vesicle membrane protein 2” for type A and “synaptotagmins I and II” for type B toxin. At this stage, the core toxin (HC–LC) enters an endosome (membrane bound compartment) inside which the disulfide bond breaks and HC and LC separate from each other. The HC domain of the toxin opens a channel in the cell membrane and translocates the light chain (LC) into the cytosol (intracellular fluid matrix) (Fig. 1.2). The LC, a protease with a zinc motif, then lands and catalyzes one of the soluble NSF proteins (SNARE) present in the presynaptic space (Rothman 1996). Deactivation of the SNARE complex prevents vesicle fusion and release of neurotransmitters (acetylcholine and others) from the presynaptic vesicles. Different serotypes of the BoNT act upon different types of SNARE proteins. BoNT-A acts upon SNAP25 (synaptosomal-associated protein), which is attached to the cell membrane (Blasi et al. 1993), whereas BoNT-B inhibits the function of synaptobrevin, a SNARE protein that is within the vesicle membrane itself (Fig. 1.2).
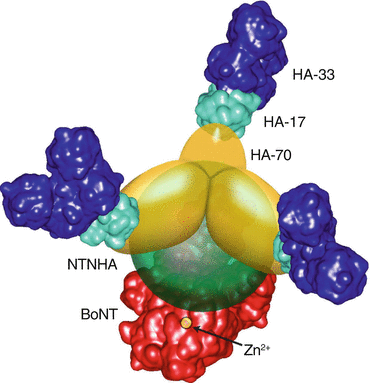
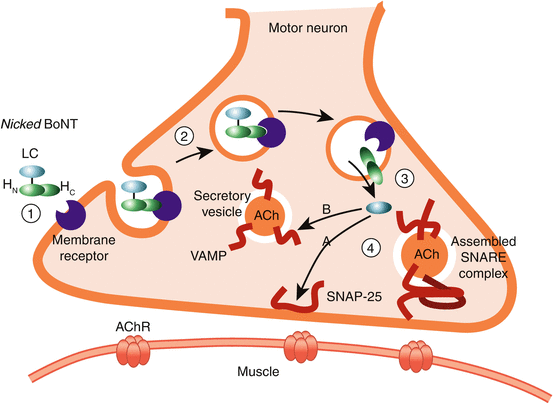
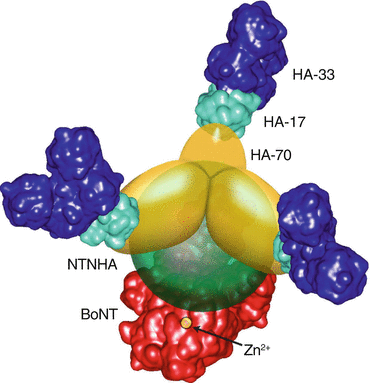
Fig. 1.1
Botulinum neurotoxin complex—core toxin and toxin-associated proteins (Reprinted with permission from Journal of Biology and Chemistry, Hasegawa et al. © 2007 The American Society for Biochemistry and Molecular Biology)
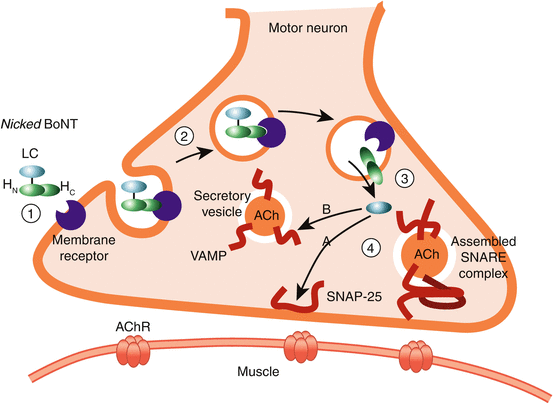
Fig. 1.2
Endocytosis of heavy and light chain (HC and LC) of botulinum neurotoxin types A and B with selective cleaving of LC to SNAP25 and synaptobrevin (From Chen and Dashtipour 2013, with permission from John Wiley and Sons © 2012 Pharmacotherapy Publications, Inc)
Currently, four types of BoNTs are used widely in clinical practice with the following FDA designations and trade names:
OnabotulinumtoxinA (onaA—trade name, Botox—Allergan, Inc., Irvine, CA), abobotulinumtoxinA (aboA—trade name, Dysport—Ipsen Biopharm Ltd., Wrexham, UK), incobotulinumtoxinA (incoA—trade name, Xeomin—Merz Pharmaceuticals LLC, Greensboro, NC), and rimabotulinumtoxinB (rimaB—trade name, Myobloc in the USA and NeuroBloc in Europe—Solstice Neurosciences, Inc., San Francisco, CA). OnaA is provided in 100 and 200 unit vials, incoA in 50 and 100 unit vials, and aboA in 300 and 500 unit vials. RimaB comes in 2,500 and 5,000 and 10,000 units/vial. Although, as emphasized by the FDA, units of various BoNTs are not interchangeable, in randomized comparator clinical trials (RCTs), approximate equivalence of the toxins is sometimes used (1 onaA unit = 1 incoA = 2.5 aboA = 40–50 units of rimaB).
RimabotulinumtoxinA comes prepared in an already diluted form in the vial. All three type A toxins (ona, abo, and inco) need to be prepared and diluted with preservative-free saline. The commonly used dilutions are in 1–2 cc of 0.9 % saline. AboA is currently approved for 1 cc dilution, although a 2 cc dilution makes it easier to compare with the onaA units (an ongoing multicenter RCT is now assessing the efficacy of 2 cc dilution for aboA). IncoA does not need refrigeration, but all other toxins do. After mixing, gentle shaking is recommended for onaA. With incoA, the manufacturer recommends gentle shaking and multiple inversions of the vial. All manufactures recommend using the prepared solution of the toxin within 4–24 h before injection. In case of onabotulinumtoxinA, however, some studies have shown retained efficacy of prepared solution up to 6 weeks if kept refrigerated at 39.2 F (4 C) and even up to 6 months if frozen (Liu et al. 2012).
Diffusion and Spread of Neurotoxins
In a recent communication, Ramirez-Castaneda et al. (2013) reviewed the diffusion, spread, and migration of the neurotoxins. The information provided in this section is largely from this review article. Overall, the authors emphasize that in most clinical conditions, diffusion of the toxin is limited, a factor that accounts for its relative safety in clinical practice.
A variety of factors could potentially influence the diffusion of the BoNTs into the injected and adjacent muscles. The total dose, number of sites injected, volume of injected solution, type of toxin, state of muscle pathology, and amount of muscle activity after injection are potential contributors to more extensive toxin diffusion. Information implicating any of these factors is still scarce and evolving, however. Although various electrophysiological studies indicate that local injection of the BoNTs causes abnormal electrophysiological changes in distant and even contralateral muscles (Lange et al 1991; Garner et al. 1993), these changes do not seem to have meaningful clinical implications since significant weakness of distant muscles rarely occurs after local injection and contralateral weakness has not been convincingly documented in clinical settings (in therapeutic doses).
Data from animal studies suggest that the extent of diffusion is dose dependent. In one study (Brodic et al. 1994), injection of one unit of onaA into the longissimus dorsi muscle of the rabbit demonstrated marked reduction of diffusion gradient beyond 15–30 mm from the site of injection, whereas injection of five to ten units caused an effect within the entire muscle. The authors used acetylcholine esterase staining to define the extent of BoNT’s spread.
The four readily available neurotoxins in the USA and Europe (ona, abo, inco, rima) have different molecular compositions (Table 1.1), and one would think that toxins with smaller molecular weight (for instance, incoA with 150 KD and practically no additional protein) would diffuse more readily and more extensively. A study in mice, however, has suggested that this may not be the case (Carli et al. 2009). Injection of ona-, abo-, and incoA toxins into the tibialis anterior muscle of mice showed very similar diffusion patterns for all three toxins regardless of their molecular weight. Most of the toxins remained close to the site of the injection and did not spread to the adjacent muscles. The investigators used neural cell adhesion molecule (N-CAM) as a measure of BoNT diffusion. N-CAM, which is present in embryonic muscle tissue and disappears in adult muscle, gets activated and reappears after the paralysis induced by intramuscular BoNT injection.
Table 1.1
Properties of different therapeutic botulinum toxins
Nonproprietary name: BoNT type | OnabotulinumtoxinA | AbobotulinumtoxinA | IncobotulinumtoxinA | RimabotulinumtoxinB |
Company | Allergan, Inc., Irvine, CA, USA | Ipsen Biopharm Ltd., Wrexham, UK | Merz Pharmaceuticals GmbH, Frankfurt, Germany | Europe/US WorldMeds, Louisville, KY, USA |
Trade name | Botox | Dysport | Xeomin | NeuroBloc/Myobloc |
Mechanism of action | Cleaves SNAP25 | Cleaves SNAP25 | Cleaves SNAP25 | Cleaves VAMP |
Molecular weight, kD | 900 | 500–900 | 150 | 700 |
Dosage form | Spray-dried powder | Freeze-dried powder | Freeze-dried powder | Sterile solution |
Shelf life, month | 36 | 24 | 36 | 24 |
Storage temperature, °C | <8 | <8 | <25 | <8 |
pH value after reconstitution | 7.4 | 7.4 | 7.4 | 5.6 |
Excipients | 500 mg HSA and 0.9 mg NaCl in 100 U vial | 125 mg HSA and 2.5 mg lactose in 500 U vial | 1,000 mg HSA and 4.7 mg sucrose in 100 U vial | 0.5 mg/cm3 HSA; 0.01 M sodium succinate; 0.1 M NaCl; and SWI in 2,500, 5,000, and 10,000 U vials |
Units per vial | 50, 100, 200 | 300, 500 | 50, 100 | 2,500; 5,000; 10,000 |
Recommended volume of reconstitution | Maximum, 10 mL | Maximum, 1 mM | Maximum, 8 mL | 0.5 mL; 1 mL; 2 mL |
Total protein, ng/vial | rv5 | rv5 | rv0.6 | rv50 |
Antigenic protein load, ng/vial | rv0.8 | Unknown | rv0.6 | rv10.7 |
Biologic activity | 100 MU-A/vial | 500 MU-I/vial
![]() Full access? Get Clinical Tree![]() ![]() ![]() |