Methylxanthine Poisoning
Michael W. Shannon†
†Deceased.
The methylxanthines most commonly used in the clinical setting are theophylline and its ethylenediamine salt, aminophylline. Until recently, theophylline was used exclusively as a bronchodilator for the management of reversible obstructive pulmonary diseases and as a respiratory stimulant for the treatment of apnea of prematurity in neonates. During the 1980s, its use fell dramatically as more effective therapies for recurrent bronchospasm became available [1]. However, there has been renewed interest in theophylline as the scope of its pharmacologic benefits broadens. Potential uses for theophylline now include preconditioning of cardiac ischemia [2], treatment of bradycardia [3], amelioration of perinatal asphyxia [4], acute mountain sickness [5], bradycardia after spinal cord injury [6], protection from contrast-induced nephropathy [7], and treatment of attention-deficit hyperactivity disorder [8]. Recent clinical trials of theophylline for asthma have demonstrated substantial benefit, restoring interest in the drug for this indication [9,10,11,12,13,14,15,16]. Despite its renewed popularity, theophylline, with its potent pharmacologic actions, variable metabolic disposition in humans, and narrow therapeutic-to-toxic ratio, is a common cause of intoxication [1,17].
Caffeine and theobromine are other widely used methylxanthines. Caffeine is found in many pharmaceutical preparations (e.g., antisleep drugs), as well as in dietary supplements, including guarana and kola nut. Although severe toxicity from caffeine ingestion is uncommon, case reports of serious poisoning in children and adults are well documented [18,19,20]. Because caffeine and other xanthine derivatives are structurally similar to theophylline, signs and symptoms of toxicity resemble those seen in theophylline intoxication, and the approach to management is identical.
Three clinical circumstances account for most cases of theophylline poisoning: unintentional ingestions by children, intentional ingestions (suicide attempts) by adolescents or adults, and medication errors (miscalculation of dose, change in frequency of administration, lack of serum drug level monitoring, or an unrecognized drug–drug or drug–disease interaction) [1,21,22]. Most cases of theophylline intoxication result from chronic, unintentional overmedication.
Pharmacology
Theophylline is available commercially as a liquid, tablet, sustained-release capsule, or solution for intravenous administration. Overdose of sustained-release theophylline can lead to a marked delay in complete absorption, with peak serum theophylline concentrations occurring as long as 15 to 24 hours after ingestion [23]. Therapeutic serum theophylline concentrations range from 10 to 20 μg per mL.
A loading dose of 5 to 6 mg per kg of intravenous aminophylline should produce a serum theophylline level of 10 μg per mL in patients not currently taking theophylline. Maintenance dosages vary with age and underlying conditions (Table 139.1). For patients taking theophylline regularly, a loading dose increases the steady-state serum theophylline level. Typically, administration of 1 mg per kg of theophylline raises the serum drug concentration by 2 μg per mL. This relationship can also be used to predict the theophylline concentration after an overdose; the maximum possible drug concentration (in micrograms per milliliter) should be no more than twice the ingested or administered dose (in milligrams per kilogram).
Theophylline has a volume of distribution of 0.4 L per kg and is 40% to 65% bound to plasma proteins [24]. Its metabolism is almost exclusively by hepatic cytochrome P450 system; it is oxidized or demethylated in the liver by at least two isoenzymes (CYP1A2 and CYP3A4) [24]. Less than 15% of the drug is excreted unchanged in urine. At therapeutic doses, hepatic metabolism generally occurs by first-order elimination kinetics [25]. The drug exhibits saturable (Michaelis–Menten) kinetics in overdose leading to prolonged, unpredictable elimination rates. The elimination half-life of theophylline also varies widely with age: typical half-lives are 20 to 30 hours in
premature infants, 4 to 7 hours in newborns, 3 to 4 hours in children 6 months to 18 years of age, and 8 to 9 hours in adults [24,25,26,27]. Many drugs, chemicals, and medical conditions affect the steady-state serum concentration and elimination half-life of theophylline (Table 139.2). The drugs that inhibit theophylline clearance are those that inhibit CYP1A2 and CYP3A4, including erythromycin, clarithromycin, ciprofloxacin, and cimetidine [24,28]. Drugs that increase theophylline clearance include barbiturates, carbamazepine, and the polyaromatic hydrocarbons of cigarette smoke (including passive smoke inhalation) [29,30]. Enzyme induction by these drugs can be temporary; if patients who smoke quit abruptly, theophylline clearance can fall to normal within days, leading to inadvertent theophylline intoxication unless dose is adjusted accordingly. Several disease states are also associated with a reduction in theophylline clearance, including congestive heart failure and liver disease [24,31]. Both hyperthyroidism and cystic fibrosis are associated with increased elimination of theophylline [32].
premature infants, 4 to 7 hours in newborns, 3 to 4 hours in children 6 months to 18 years of age, and 8 to 9 hours in adults [24,25,26,27]. Many drugs, chemicals, and medical conditions affect the steady-state serum concentration and elimination half-life of theophylline (Table 139.2). The drugs that inhibit theophylline clearance are those that inhibit CYP1A2 and CYP3A4, including erythromycin, clarithromycin, ciprofloxacin, and cimetidine [24,28]. Drugs that increase theophylline clearance include barbiturates, carbamazepine, and the polyaromatic hydrocarbons of cigarette smoke (including passive smoke inhalation) [29,30]. Enzyme induction by these drugs can be temporary; if patients who smoke quit abruptly, theophylline clearance can fall to normal within days, leading to inadvertent theophylline intoxication unless dose is adjusted accordingly. Several disease states are also associated with a reduction in theophylline clearance, including congestive heart failure and liver disease [24,31]. Both hyperthyroidism and cystic fibrosis are associated with increased elimination of theophylline [32].
Table 139.1 Intravenous Aminophylline Maintenance Doses | ||||||||||||||||||||||
---|---|---|---|---|---|---|---|---|---|---|---|---|---|---|---|---|---|---|---|---|---|---|
|
Table 139.2 Factors Affecting Serum Theophylline Concentrations | |
---|---|
|
Theophylline has a variety of physiologic effects in therapeutic doses (Table 139.3). These effects include smooth muscle relaxation, mild central nervous system (CNS) excitation, and diuresis. Intoxication is associated with an array of other metabolic and clinical consequences. Although the effects of theophylline have been well characterized, their pharmacologic and pathophysiologic mechanisms remain poorly understood. Three primary cellular mechanisms of theophylline action have been theorized: inhibition of cyclic guanosine monophosphate or cyclic adenosine monophosphate (cAMP) activity, adenosine receptor antagonism, and adrenergic hyperstimulation (particularly at the beta-receptor) secondary to elevated levels of circulating plasma catecholamines [33,34,35,36]. Inhibition of calcium translocation and leukotriene production has also been postulated to be a fourth mechanism.
The physiologic changes seen with therapeutic doses of theophylline, including tachycardia, diuresis, bronchodilation, and CNS excitation, were thought to result from theophylline’s inhibition of phosphodiesterase, the intracellular enzyme that inactivates cAMP, an important “second messenger” [37]. Such enzyme inhibition would lead to elevated intracellular cAMP concentrations, affecting a broad range of physiologic responses. However, this theory has been brought into question; in vitro data indicate that phosphodiesterase inhibition does not occur at therapeutic serum concentrations of theophylline, suggesting that increased cAMP activity is not a major mechanism of its therapeutic effects [38]. Whether the increased theophylline concentrations seen in the intoxicated patient are sufficient to inhibit phosphodiesterase activity is unknown.
Table 139.3 Physiologic Effects of Theophylline | |
---|---|
|
Investigation has also been directed at the role of adenosine receptor antagonism as a mechanism of theophylline action. Adenosine is a nucleoside that promotes smooth muscle constriction, slows cardiac conduction, and acts as an endogenous anticonvulsant. With the structure of theophylline being similar to that of adenosine and with the drug having opposite physiologic actions, theophylline may be a simple competitive antagonist at bronchial and vascular smooth muscle, cardiac, and CNS sites. However, adenosine antagonism alone does not provide a complete explanation for theophylline’s pharmacologic effects [39,40].
Additional data suggest that many of theophylline’s actions can be accounted for by its stimulation of plasma catecholamines release [32,41]. Plasma concentrations of epinephrine, norepinephrine, and dopamine all rise significantly after theophylline administration [40]. With therapeutic doses, plasma catecholamine activity typically increases four- to sixfold. After theophylline intoxication, plasma catecholamine activity may rise to 30-fold [33,35]. Increased plasma catecholamines provide a ready explanation for many of the effects of theophylline seen after therapeutic doses and
potentially mediate many of the effects of theophylline intoxication. In all probability, the combined effects of adenosine receptor antagonism and catecholamine release are responsible for the predominant effects of theophylline intoxication.
potentially mediate many of the effects of theophylline intoxication. In all probability, the combined effects of adenosine receptor antagonism and catecholamine release are responsible for the predominant effects of theophylline intoxication.
Plasma catecholamines, particularly epinephrine, are capable of inducing hypokalemia, hyperglycemia, and metabolic acidosis. Epinephrine-induced hypokalemia appears to result from β2-adrenergic receptor–linked stimulation of Na+/K+ adenosine triphosphatase. This leads to increased intracellular transport of potassium with preservation of total body potassium content [42]. Consistent with the theories of plasma catecholamine activity is the observation that theophylline-induced hypokalemia can be inhibited by pretreatment with propranolol or reversed by propranolol administration [43].
The CNS effects of theophylline intoxication include respiratory stimulation, vomiting, and seizures. These may result from disturbances in CNS cyclic guanosine monophosphate activity, adenosine antagonism, or adrenergic excess. Changes in neuronal transmembrane potentials by any of these mechanisms would lower excitation thresholds. Additionally, there are theories that theophylline inhibits CNS γ-aminobutyric acid receptor activity and stimulates N-methyl-D-aspartate and other excitatory neurotransmitters production. Theophylline administration has been associated with an abnormal electroencephalogram pattern in 34% of children and 12% of adults [44,45]. Cerebral vascular effects are also significant with theophylline and other methylxanthines because they are potent cerebral vasoconstrictors. This is the presumed mechanism of the efficacy of caffeine in the treatment of migraine headache. However, decreases in cerebral blood flow can be extreme, particularly during inhalational anesthetics administration [46]. In animal models, theophylline amplifies brain damage induced by seizures [47].

Full access? Get Clinical Tree
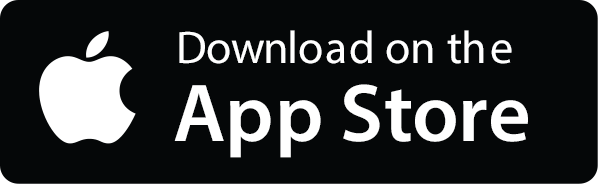
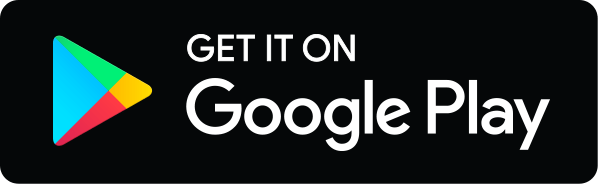