For adults, total energy expenditure averages 39 kcal/kg in males and 34 kcal/kg in females. Approximately 20 kcal/kg is expended as basal metabolism necessary to maintain integrity of the cell membrane and other energy-requiring tasks essential for life. In the resting state, the basal expenditure of calories is equivalent to approximately 1.1 kcal per minute, which requires approximately 200 to 250 mL per minute of oxygen in a 70-kg man for oxidation of nutrients. As the level of activities increase above the basal state, the caloric (and oxygen) requirements increase in proportion to the energy expenditure required (Table 33-1). The caloric values of carbohydrates, fats, and proteins are approximately 4.1 kcal/g, 9.3 kcal/g, and 4.1 kcal/g, respectively. Fat forms the major energy storage depot because of its greater mass and high caloric value (Fig. 33-2).1 As a consequence, the primary form in which potential chemical energy is stored in the body is fat (triglyceride). The high caloric density and hydrophobic nature of triglycerides permit efficient energy storage without adverse osmotic consequences.


Carbohydrate Metabolism
Carbohydrates comprise a group of organic compounds that include sugars and starches and, in addition to carbon, contain hydrogen and oxygen in the same ratio as water (2:1). Three disaccharides are important in human biology—sucrose: glucose and fructose; lactose: glucose and galactose; and maltose: glucose and glucose. Starch, found in grains such as wheat, rice, and barley and other plants, including potatoes and corn, consists of many units of glucose joined by glycosidic bonds. Sugars are an important energy source for the body and the sole source of energy for the brain.
The liver is the site of carbohydrate metabolism where regulation, storage, and production of glucose takes place. The liver is the only organ that contains glucose kinase, an enzyme that has a high reaction rate (Km), capable of phosphorylating glucose, but only when its concentration is high. Adequate concentrations appear immediately after a meal when glucose concentration in the portal vein is increased. At least 99% of all the energy derived from carbohydrates is used by mitochondria to form ATP in the cells (Fig. 33-3). The final products of carbohydrate digestion in the gastrointestinal tract are glucose, fructose, and galactose. After absorption into the circulation, fructose and galactose are rapidly converted to glucose. As a result, glucose is the predominant molecule used to produce ATP. This glucose must be transported through cell membranes into cellular cytoplasm before it can be used by cells. This transport uses a protein carrier in carrier-mediated diffusion, which is enhanced by insulin. Resistance to insulin, and thus transport of glucose into the cell in diabetes mellitus or sepsis,2 results in hyperglycemia with associated adverse sequelae. Immediately upon entering cells, glucose is converted to glucose-6-phosphate under the influence of the enzyme hexose kinase. Phosphorylated glucose is ionized at pH 7 and, because plasma membranes are not permeable to the ions, the phosphorylated glucose cannot pass back through the membrane and is effectively trapped within the cell.

The fetus derives almost all its energy from glucose obtained from the maternal circulation. Immediately after birth, the infant stores of glycogen are sufficient to supply glucose for only a few hours. Furthermore, gluconeogenesis is limited in the neonate. As a result, the neonate is vulnerable to hypoglycemia if feeding is not initiated.
Glycogen
After entering cells, glucose can be used immediately for release of energy to cells or it can serve as a substrate for glycogen synthase. Dephosphorylation of the enzyme, glycogen synthase by protein phosphatase-1, which in turn is regulated by insulin and glucagon, activates the enzyme. Activated glycogen synthase combines molecules of glucose into a long polymer, similar to the way plants store carbohydrate as starch. Glycogen synthase is deactivated when it is phosphorylated—by glycogen synthase kinase-3, 5′-adenosine monophosphate–activated protein kinase, and protein kinase-A. The liver and skeletal muscles are particularly capable of storing large amounts of glycogen, but all cells can store at least some glucose as glycogen, and the glycogen in these cells is increasingly recognized as having important roles in both health and disease.3 The liver stores glycogen for release of glucose during fasting, and muscle, which can store as much as 90% of the glucose contained in a meal, catabolizes glycogen during strenuous exercise.4 The ability to form glycogen makes it possible to store substantial quantities of glucose without significantly altering the osmotic pressure of intracellular fluids. Glucose is cleaved from glycogen between meals, during fasting, and during exercise by glycogen phosphorylase and by a debranching enzyme.
Gluconeogenesis
Gluconeogenesis is the formation of glucose from amino acids and the glycerol portion of fat. Amino acids are first deaminated before entering the citric acid (Krebs) cycle (see Fig. 33-3). This process occurs when body stores of glycogen decrease below normal levels. An estimated 60% of the amino acids in the body’s proteins can be converted easily to pyruvate and glucose, whereas the remaining 40% have chemical configurations that make this conversion difficult.
Gluconeogenesis is stimulated by hypoglycemia. Particularly in the liver, simultaneous release of cortisol mobilizes proteins, making them available for breakdown to amino acids used in gluconeogenesis. Thyroxine is also capable of increasing the rate of gluconeogenesis.
Energy Release from Glucose
Glucose is progressively broken down into two molecules of pyruvate, both of which can enter the citric acid cycle (Fig. 33-4), and the resulting energy is used to form ATP. For each mole of glucose that is completely degraded to carbon dioxide and water, a total of 38 moles of ATP is ultimately formed. The most important means by which energy is released from the glucose molecule is by glycolysis and the subsequent oxidation of the end products of glycolysis. Glycolysis is the splitting of the glucose molecule into two molecules of pyruvate, which enter the mitochondria where the pyruvate is converted to acetyl-coenzyme A (CoA), which enters the citric acid cycle and is converted to carbon dioxide and hydrogen ions with the formation of ATP (oxidative phosphorylation). Oxidative phosphorylation occurs only in the mitochondria and in the presence of adequate amounts of oxygen.

Anaerobic Glycolysis
In the absence of adequate amounts of oxygen, a small amount of energy can be released by anaerobic glycolysis, also known as fermentation in plants, fungi, and bacteria because conversion of glucose to pyruvate does not require oxygen. Indeed, glucose is the only nutrient that can serve as a substrate for the formation of ATP without oxygen. This release of glycolytic energy to cells can be lifesaving for a few minutes should oxygen become unavailable.
During anaerobic glycolysis, most pyruvic acid is converted to lactic acid, which diffuses rapidly out of cells into extracellular fluid. When oxygen is again available, this lactic acid can be reconverted to glucose. This reconversion occurs predominantly in the liver. Indeed, severe liver disease may interfere with the ability of the liver to convert lactic acid to glucose, leading to metabolic acidosis.
Lipid Metabolism
Lipids are hydrophobic organic molecules that include waxes, sterols, fat-soluble vitamins, triglycerides (fats), phospholipids, and other substances. Lipids contain a high amount of potential energy, but are also important as structural components of cell membranes, in signaling pathways, and as precursors to a number of cytokines. Fatty acids and their derivatives as well as molecules that contain sterols such as cholesterol are also considered lipids. Although there are biosynthetic pathways to synthesize and degrade lipids, some fatty acids are essential and must be ingested in the diet. Fatty acids are carboxylic acids consisting of a long hydrocarbon chain ending in a carboxyl group; the hydrocarbon chain can be saturated or unsaturated (Fig. 33-5). Humans can desaturate carbon atoms no closer than the 9th carbon from the tail of the aliphatic chain. However, humans require fatty acids (that are therefore essential) that are desaturated as close as the 6th and as close as the 3rd carbon to the terminus of the aliphatic chain—ω6 and ω3 fatty acids, respectively. Twenty carbon chain fatty acids are stored in the second position of phospholipids (see the following text), and when released, serve as substrates for a group of very important cytokines, the eicosanoids—prostaglandins, thromboxanes, and leukotrienes. Arachidonic acid (see Fig. 33-5), a 20 carbon chain v6 fatty acid (C20:4ω6) is a precursor for prostaglandins and thromboxanes of the two series and leukotrienes of the four series, whereas eicosapentaenoic acid, C20:5ω3, is a precursor for prostaglandins and thromboxanes of the three series and leukotrienes of the five series.

A glycerol stem to which three fatty acid molecules are bound is known as a triglyceride (Fig. 33-6). A triglyceride molecule to which one of the terminal fatty acids is replaced with a phospate ion is known as a phospholipid (Fig. 33-7). Phospholipids are the building blocks of cell membranes (Fig. 33-8), form myelin, and, because of their unique structure and functions, are being used in other scientific applications.




Full access? Get Clinical Tree
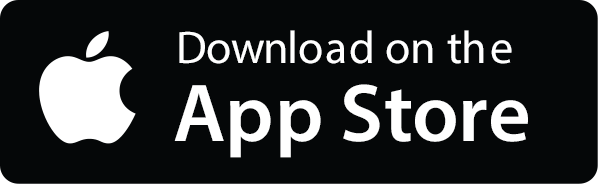
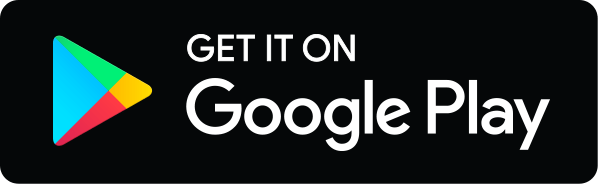