Metabolic Acidosis and Metabolic Alkalosis
Robert M. Black
Normal Acid–Base Physiology
Acidemia and alkalemia denote, respectively, blood pHs below or above the normal value of 7.40. A simple (single) acid–base disturbance always causes the blood pH to change. In comparison, the coexistence of two opposing primary acid–base disturbances, such as a metabolic acidosis due to diarrhea with metabolic alkalosis due to vomiting, may result in little or no deviation of the blood pH from normal. Maintenance of blood pH at approximately 7.40 is necessary to stabilize intracellular pH at 7.20, a crucial chemical condition for optimal cell physiology.
Renal Regulation of H+ Secretion
Maintenance of a normal plasma bicarbonate (HCO3–) concentration depends on reclamation of the 4,500 mEq of HCO3– filtered by the kidneys each day. Reabsorption of filtered HCO3– takes place almost entirely in the proximal tubule (Fig. 71.1). In this process, luminal HCO3– combines with H+ secreted into the tubular lumen by an Na-H antiporter. The formation and subsequent dissociation of carbonic acid (H2CO3) to carbon dioxide (CO2) and water (H2O), catalyzed by carbonic anhydrase, permit CO2 to enter the luminal membrane of the proximal tubular cell. Once inside the cell, CO2 combines with OH– to form HCO3–. An Na-3HCO3 cotransporter then carries HCO3– across the peritubular membrane into the blood. As a result, filtered HCO3– is returned to the circulation without any net loss of H+.
A fall in proximal tubular bicarbonate reabsorption causes urinary HCO3– loss and may lead to a fall in plasma HCO3– concentration and to metabolic acidosis. The carbonic anhydrase inhibitor acetazolamide, for example, reduces the activity of luminal carbonic anhydrase, thereby decreasing the entry of H2O and CO2 across the luminal membrane, which decreases HCO3– reabsorption by the tubular cell (Fig. 71.1).
The process of reclamation of all filtered HCO3– by itself is not sufficient to maintain a normal blood pH. The kidney must also excrete the 50 to 100 mEq per kg of H+ generated each day from the metabolism of dietary proteins, particularly sulfur-containing amino acids (i.e., methionine, cystine), which are converted to sulfuric acid. This acid load is initially buffered in the body to minimize changes in blood pH, causing a clinically undetectable decrease in the plasma HCO3– concentration. The kidney must eventually excrete this daily acid increment to replete the HCO3– used in this process, however, or more severe acidemia will develop over time.
Energy requirements limit the ability of the kidney to excrete acid (H+ ions) when the urine pH falls below 4.5. To offset this limitation, urinary buffers are present in the urine that maintain the urine pH above this critical value, permitting ongoing excretion of the daily acid load.
Two distinct urinary buffering systems enable continued H+ secretion: titratable acids and ammonia. Titratable acids (primarily HPO4)1 are freely filtered through the glomerulus and can combine with H+:

Approximately one-half of the daily acid load is excreted in this way.
By comparison, the most important urinary buffer is ammonia, as the abundance of this buffer can be varied according to physiologic needs. Ammonia synthesis occurs in the proximal tubule, derived principally from the breakdown of glutamine to α-ketoglutarate (Fig. 71.2) [1]. This process is stimulated by intracellular acidosis and by hypokalemia, both of which act by decreasing the intracellular pH (see following). Ammonia thus generated can combine with intracellular H+, forming ammonium (NH4+). NH4+ is then secreted into the proximal tubule lumen by substituting for H+ on the Na-H antiporter. Ammonia (NH3) that forms by the dissociation of H+ from NH4+ is largely reabsorbed, recycled, and then secreted into the collecting tubule. There, it is trapped in the tubular lumen as NH4+ by combining with secreted H+ and excreted as ammonium chloride (NH4Cl). For each molecule of buffered H+ excreted in the urine, an HCO3– is regenerated (Fig. 71.2), thus replenishing the HCO3– used initially by the body to buffer daily metabolic acid load.
Metabolic Acidosis
Metabolic acidosis can be categorized by the presence or absence of an increased anion gap. The anion gap (AG) refers to the difference between measured cations (Na+) and measured anions (chloride [Cl–] and HCO3–):2

The normal AG varies between 3 and 11 mEq per L and averages approximately 7 to 8 mEq per L [2]. These unmeasured anions consist of proteins (primarily albumin), sulfates, phosphates, and circulating organic acids. Uric acid is a large molecule and therefore does not contribute significantly to the AG even when hyperuricemia is present.
A reduction in the plasma albumin concentration can lower the baseline AG (approximately 2.5 mEq for every 1 g per dL fall in the albumin concentration) [3]. Thus, the hypoalbuminemic patient may not have a high AG even in the presence of a disorder that typically causes an elevation (e.g., lactic acidosis; see later).
Metabolic Acidosis with an Increased Anion Gap
The causes of metabolic acidosis associated with an increased AG are listed in Table 71.1. Lactic acidosis is the most frequent form in hospitalized patients, whereas chronic renal failure is the principal cause of an increased AG in ambulatory persons.
Chronic Kidney Disease (CKD)
Renal disease represents an interesting example of the potential overlap between normal and elevated AG acidosis. The high AG in patients with advanced chronic kidney disease is usually a late finding and reflects a severe reduction in glomerular filtration rate (GFR). As the GFR falls below 20 to 30 mL per minute (plasma creatinine > 3 to 4 mg per dL), anions, such as sulfate and phosphate, that would normally be excreted by filtration are retained. With lesser degrees of renal dysfunction, however, metabolic acidosis appears primarily because H+ (HCl) secretion is reduced, with little or no effect on the AG.
The metabolically generated daily acid load on a typical American diet approximates 50 to 100 mEq. This acid, mainly sulfuric, is immediately buffered by NaHCO3:

The excess sulfate is excreted in the urine. If glomerular and tubular function decline in parallel, then the H+ and the SO42- are retained, producing metabolic acidosis with a high AG. If, however, there is more significant tubular dysfunction, the excretion of acid is diminished, but excretion of sulfate may be maintained due to reduced reabsorption. In the latter setting, the AG may not rise as the serum HCO3– concentration decreases.
Therefore, the decrease in plasma HCO3– (severity of acidemia) need not correlate with extent of the rise in AG in renal dysfunction. Typically, the plasma bicarbonate concentration is greater than 12 mEq per L in patients with uncomplicated CKD. A search for a second acid–base disorder is indicated when a lower HCO3– concentration is identified.
Lactic Acidosis
Lactic acidosis is probably the most common cause of severe metabolic acidosis encountered in the intensive care unit. The AG is always increased above baseline (normal lactate level
is < 1.0 mmol per L)3 because lactate does not appear in the urine until a higher plasma concentration (at least 6 to 8 mmol per L) is achieved. Lactate levels greater than 5 mmol per L are considered diagnostic of lactic acidosis, although levels between 2 and 5 mmol per L may be significant in the appropriate clinical circumstances [4]. Metformin, a biguanide commonly used in the treatment of type II diabetes mellitus, can cause lactic acidosis, particularly in patients who present with acute or chronic renal insufficiency. Hemodialysis has been used in the treatment of metformin-induced acidosis [5].
is < 1.0 mmol per L)3 because lactate does not appear in the urine until a higher plasma concentration (at least 6 to 8 mmol per L) is achieved. Lactate levels greater than 5 mmol per L are considered diagnostic of lactic acidosis, although levels between 2 and 5 mmol per L may be significant in the appropriate clinical circumstances [4]. Metformin, a biguanide commonly used in the treatment of type II diabetes mellitus, can cause lactic acidosis, particularly in patients who present with acute or chronic renal insufficiency. Hemodialysis has been used in the treatment of metformin-induced acidosis [5].
Table 71.1 Causes of Metabolic Acidosis with an Increased Anion Gap | ||
---|---|---|
|
Most cases of lactic acidosis involve the L-isomer. By comparison, D-lactic acidosis, a disorder observed most commonly in patients with abnormal bowel anatomy, results in a rise in the AG, but the lactate level is normal [6]. D-Lactate is not detected by the usual lactate assay, which measures only L-lactate and a specific assay must be requested to diagnose this disorder.
Ketoacidosis
Ketoacidosis occurs when acetoacetic acid and β-hydroxybutyric acid are overproduced by the liver (see Chapter 101 for a complete discussion). Acetone, a breakdown product of acetoacetic acid, is not an acid; as such, it does not contribute to the acidemia or to the increased AG observed in this disorder.4
Although ketoacidosis is generally associated with an elevated AG, loss of ketoanions in the urine, particularly during intravenous fluid therapy, may attenuate the expansion of the
AG. Once formed, ketones may be excreted in the urine before, under the influence of insulin, they can be metabolized back to HCO3–. Because the initially produced ketoacids titrate the plasma HCO3– concentration downward, the loss of urinary ketoanions (as sodium or potassium salts) is tantamount to the renal loss of HCO3–. The net effect is that a high AG metabolic acidosis is present before therapy in most individuals with ketoacidosis but may convert to a normal AG metabolic acidosis once saline repletion occurs and ketogenesis ceases [7].
AG. Once formed, ketones may be excreted in the urine before, under the influence of insulin, they can be metabolized back to HCO3–. Because the initially produced ketoacids titrate the plasma HCO3– concentration downward, the loss of urinary ketoanions (as sodium or potassium salts) is tantamount to the renal loss of HCO3–. The net effect is that a high AG metabolic acidosis is present before therapy in most individuals with ketoacidosis but may convert to a normal AG metabolic acidosis once saline repletion occurs and ketogenesis ceases [7].
Rhabdomyolysis
Massive muscle breakdown is an important cause of metabolic acidosis with an increased AG. Acute kidney injury due to myoglobinuria can cause retention of anions (e.g., phosphate) that have been released from damaged myocytes.
Ingestions
The most common acid–base abnormality observed with salicylate intoxication is a respiratory alkalosis caused by direct stimulation of the medullary respiratory center. A pure metabolic acidosis owing to aspirin toxicity is uncommon. With moderate-to-severe salicylate intoxication, the AG increases as salicylic acid, not simply due to accumulation of salicylate in the blood, promotes formation of lactic acid. The consequence is a mixed respiratory alkalosis with a high AG metabolic acidosis.
Methanol and ethylene glycol ingestions require early diagnosis because prompt treatment may be lifesaving. Inhibitors of alcohol dehydrogenase such as ethanol and fomepizole are used for this latter purpose, with fomepizole being the preferred agent, if available. Either agent can limit the conversion of the alcohols to their more toxic metabolic products. Methanol or ethylene glycol ingestion as a cause for high AG metabolic acidosis is suggested by the history and physical findings (see Chapter 119). The turnaround time for measurement of these toxins may delay treatment. The detection of an osmolal gap is a relatively quick way of supporting the suspected diagnosis.
The osmolal gap refers to the difference between the plasma osmolality (POsm) measured by the laboratory and that calculated using the following formula:

Normally, the measured POsm is higher than the calculated value by 10 mOsm per kg. A larger osmolal gap indicates the presence of osmotically active substances not normally present. The most frequent causes of an increased osmolal gap are ethyl alcohol, isopropyl alcohol, ketones, lactate, mannitol, ethylene glycol, and methanol. If ethanol, lactate, or ketones cannot be identified in a patient with an AG metabolic acidosis with an osmolal gap, the diagnosis of ethylene glycol or methanol intoxication should be strongly suspected [8]. In the intensive care unit setting, a high osmolal gap acidosis has also been associated with the use of continuous high-dose infusions of lorazepam for more than 48 hours. Propylene glycol, which is used as a solvent for intravenous medications including lorazepam, has been implicated as the cause of the hyperosmolar metabolic acidosis in this scenario [9]. It is important to understand that the presence of an osmolal gap that results from an ingested alcohol may only be detected when POsm is measured in the laboratory by freezing-point depression [10]. Also, after the alcohol is metabolized, the osmolal gap may disappear.
Toluene (present in glue and metabolized to hippuric acid) is a rare cause of metabolic acidosis. The AG rises early and then returns toward normal, as hippurate is excreted by the kidneys, a process that is similar to the renal handling of ketones (see previous discussion) [11].
Rarely, acetaminophen administration in therapeutic doses can lead to an elevated AG metabolic acidosis in metabolically stressed individuals, including pregnant women. In this setting, reduced glutathione stores permit the generation of pyroglutamic acid (5-oxoproline) [12].
Metabolic Acidosis with a Normal Anion Gap
Metabolic acidosis with a normal AG, which may also be called a hyperchloremic acidosis, is associated with the conditions listed in Table 71.2. The decrement in the plasma HCO3– concentration is matched by a rise in the plasma Cl– level, maintaining electroneutrality.
Acid and Chloride Administration
The infusion of amino acid solutions during hyperalimentation is an abundant source of hydrochloric acid (HCl). The development of a metabolic acidosis is more common in patients with renal insufficiency.
Oral administration of cholestyramine chloride reportedly occasionally also causes acidemia. This resin, which is sometimes used in the management of hypercholesterolemia, is nonresorbable and can act as an anion-exchange resin, exchanging its Cl– for endogenous HCO3– and producing a metabolic acidosis. Sevelamer chloride, a compound used as a phosphorous binder in chronic kidney disease, has been associated with lower bicarbonate levels than in those patients treated with calcium-based binders. The mechanism of the metabolic acidosis is believed to be similar to cholestyramine [13].
Bicarbonate Losses
Loss of HCO3– from the gastrointestinal tract or kidneys can lead to a reduction in the plasma HCO3– level. Bowel contents are alkaline compared to blood because HCO3– is added by pancreatic and biliary secretions. HCO3– is later exchanged for Cl– in the ileum and colon. The result is that most alkali secreted into the gut lumen is reclaimed by the colon. Gastrointestinal losses of HCO3– (or HCO3– precursors such as lactate and acetate) are most commonly observed in patients with diarrhea so severe that colonic transit time is too rapid for
alkali reabsorption. At times, the resulting HCO3– losses can approach 40 mEq per L of stool. Less frequently, metabolic acidosis from HCO3– depletion is a result of pancreatic fistulae, biliary drainage, or a ureterosigmoidostomy. In the last circumstance, the excretion of acid (as NH4Cl) urine directly into the colon permits the exchange of HCl for HCO3– because the colon is permeable to H+ and Cl–, unlike the urinary bladder [14]. This problem does not usually occur with an ileal bladder.
alkali reabsorption. At times, the resulting HCO3– losses can approach 40 mEq per L of stool. Less frequently, metabolic acidosis from HCO3– depletion is a result of pancreatic fistulae, biliary drainage, or a ureterosigmoidostomy. In the last circumstance, the excretion of acid (as NH4Cl) urine directly into the colon permits the exchange of HCl for HCO3– because the colon is permeable to H+ and Cl–, unlike the urinary bladder [14]. This problem does not usually occur with an ileal bladder.
Table 71.2 Causes of Metabolic Acidosis with a Normal Anion Gap | |
---|---|
|
Table 71.3 Some Causes of Types 1 and 2 Renal Tubular Acidosis (RTA) | ||||||
---|---|---|---|---|---|---|
|
Pancreatic HCO3– losses are also observed in essentially all patients with a pancreatic allograft anastomosed directly to the urinary bladder. Bicarbonate secreted into the bladder cannot be reabsorbed.
Renal bicarbonate losses can cause or contribute to acidemia in type 2 (proximal) renal tubular acidosis (RTA; Table 71.2),5 during recovery from ketoacidosis (see previous discussion), and in patients who are posthypercapnia. In patients with proximal RTA (Table 71.3), the normal reabsorptive threshold for HCO3– is reduced. As a result, HCO3– can no longer be reabsorbed at a rate adequate to maintain the normal plasma level of approximately 25 mEq per L. As a consequence, the urine pH is alkaline (> 5.3), and the fractional excretion of HCO3– is elevated (> 15% of the filtered load).6 Normally, this value is less than 3% because more than 97% of HCO3– filtered through the glomerulus is reclaimed, primarily in the proximal tubule (Fig. 71.1). HCO3– wasting ceases, however, and the urine becomes acidic (pH < 5.3) once the plasma HCO3– concentration has stabilized at the new (lower) level. This process explains why the urine pH may be high or low in proximal RTA.
Renal HCO3– losses also occur as compensation for chronic respiratory alkalosis (chronic hypocapnia). During chronic hyperventilation, the blood pH increases as the PCO2 decreases. As can be seen in Figure 71.1, an increase in intracellular pH diminishes H+ excretion, leading to a concomitant decrease in HCO3– reabsorption. These changes cause the plasma HCO3– concentration to fall, partially compensating for the alkalemia. If the stimulus for hyperventilation (e.g., hypoxemia) is suddenly eliminated, the PCO2 rapidly returns to normal. Renal compensation, by comparison, continues for 1 to 2 more days, causing a persistent reduction in the plasma HCO3– concentration. The resulting posthypocapnic metabolic acidosis normally resolves spontaneously.

Full access? Get Clinical Tree
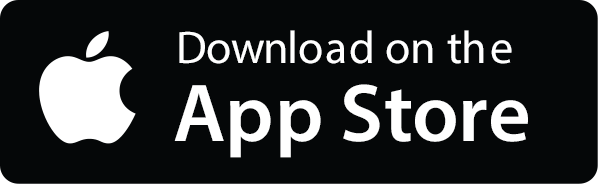
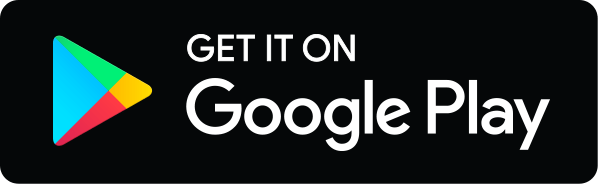
