Abstract
Understanding the basic principles of pharmacology is fundamental to the practice of medicine in general, but it is perhaps most relevant to the practice of anesthesiology. It is now widely accepted that cells contain a host of specific receptors that mediate the medicinal properties of drugs. Although the use of plant-derived medicinal compounds dates back to antiquity, the mechanisms by which these drugs act to modify disease processes remained mysterious until relatively recently. As late as 1964, de Jong wrote, “To most of the modern pharmacologists the receptor is like a beautiful but remote lady. He has written her many a letter and quite often she has answered the letters. From these answers the pharmacologist has built himself an image of this fair lady. He cannot, however, truly, claim ever to have seen her, although one day he may do so.” 1 This chapter briefly reviews the history of the receptor concept from the abstract notion alluded to by de Jong to the modern view of receptors as specific, identifiable cellular macromolecules to which drugs must bind to initiate their effects. Also introduced and defined are basic concepts that describe drug-receptor interactions such as affinity, efficacy, specificity, agonism, antagonism, and the dose-response curve. Finally, the evolving discipline of molecular pharmacology is discussed as it relates to modern drug development. Mathematic representations of the concepts are included in the form of equations for the reader seeking quantitative understanding, although the explanations of key concepts in the text are intended to be understood without reliance on mathematics.
Keywords
receptor, agonist, affinity, efficacy, potency, antagonist, signal transduction
Chapter Outline
Understanding the basic principles of pharmacology is fundamental to the practice of medicine in general, but is perhaps most relevant to the practice of anesthesiology. It is now widely accepted that cells contain a host of specific receptors that mediate the medicinal properties of drugs. Although the use of plant-derived medicinal compounds dates back to antiquity, the mechanisms by which these drugs act to modify disease processes remained mysterious until relatively recently. As late as 1964, de Jong wrote, “To most of the modern pharmacologists the receptor is like a beautiful but remote lady. He has written her many a letter and quite often she has answered the letters. From these answers the pharmacologist has built himself an image of this fair lady. He cannot, however, truly, claim ever to have seen her, although one day he may do so.”
This chapter briefly reviews the history of the receptor concept from the abstract notion alluded to by de Jong to the modern view of receptors as specific, identifiable cellular macromolecules to which drugs must bind in order to initiate their effects. Also introduced and defined are basic concepts that describe drug-receptor interactions such as affinity, efficacy, specificity, agonism, antagonism, and the dose-response curve. Finally, the evolving discipline of molecular pharmacology is discussed as it relates to modern drug development. Mathematical representations of the concepts are included in the form of equations for the reader seeking quantitative understanding, although the explanations of key concepts in the text are intended to be understood without reliance on mathematics.
The Receptor Concept
Historical Beginnings
The specificity of drugs for a particular disease has been known since at least the 17th century. The best known example of this is the efficacy of Peruvian bark, the predecessor of quinine, in the treatment of malaria. Sobernheim (1803–1846) first applied the concept of selective affinity to explain the apparent specificity of drugs. He believed, for example, that strychnine had an affinity for spinal cord while digitalis had affinity for the heart. Blake (1814–1893) first demonstrated that inorganic compounds with similar macroscopic crystalline structures exert similar effects when administered intravenously. This triggered a vigorous scientific debate at the turn of the 20th century regarding whether it was the chemical structure or physical properties of drugs that endow them with medicinal properties. This debate was particularly relevant for the theories of actions of general anesthetics because it was believed until recently that their relatively simple and diverse chemical structures precluded the possibility of a specific drug-receptor interaction.
The term receptor was first coined in 1900 by Ehrlich (1854–1915) as a replacement for his original term “side chain” (Seitenkette) that he used to explain the specificity of the antibacterial actions of antitoxins (antibodies). Ehrlich did not originally believe that specific receptors existed for small molecules such as medicinal compounds because they could easily be washed out of the body by solvents. This belief was at odds with the remarkable experimental findings of Langley (1852–1925), who was investigating whether the origin of the automatic activity of the heart resided in the heart muscle itself or was imposed on the heart by the nervous system. He demonstrated that the effect of the plant-derived drug jabonardi—bradycardia—occurred even when innervation was blocked, and that this effect was reversed by applying atropine directly to the heart. He went on to show that the relative abundance of the agonist (jabonardi) over its antagonist (atropine) determined the overall physiologic effect. This observation led Langley to propose that competition of the two drugs for binding to the same substance explained their antagonistic effects on the heart rate. However, the key experiment that led Langley to formulate his receptor concept came 30 years later in 1905, when he showed that the contractile effect of nicotine on skeletal muscle can be antagonized by curare. From the observation that even after application of curare the relaxed muscle contracted following direct application of electric current, he concluded that neither curare nor nicotine acted directly on the contractile machinery. Instead, Langley argued that the drugs interacted with a “receptive substance” that was essential for the initiation of the physiologic actions of the drug.
Modern Development
Langley’s concept of “receptive substance” forms the basis of the modern concept of a receptor, but it was not accepted without debate. It took years of work by Clark (1885–1941) and Gaddum (1900–1965), among others, to solidify the receptor concept. Clark demonstrated that the relationship between drug concentration and the physiologic response formed a hyperbolic relationship (the familiar sigmoidal dose-response curve; see later). Clark concluded that the relationship arose from equilibration between the drug and its receptor and argued that the effect was directly proportional to the number of drug-receptor complexes. Ariëns (1918–2002) elaborated on Clark’s theory and showed that the affinity of the drug for the receptor is distinct from the ability of the drug-receptor complex to elicit a physiologic response. This distinction was further elaborated by Stephenson (1925–2004), who mathematically defined and quantified efficacy— the propensity of a drug to elicit a response. Through his investigation of sympathomimetic compounds, Ahlquist (1914–1983) found that responses in various tissues occurred with two distinct orders of potency. This led him to propose multiple types of receptors for the same drug (α- and β-adrenergic receptors in this case), and the concept of specificity, which was finally published in 1948 after multiple rejections.
Ahlquist’s work is the foundation of modern pharmacology, including the development of the first and still widely used receptor-specific drugs—β-blockers—by Black (1924–2010), who also developed H 2 -histamine receptor blockers used to diminish stomach acid production in the treatment of peptic ulcer disease. Since Black’s fundamental discovery, many receptors have been identified, structures of many drug-receptor complexes have been solved using x-ray crystallography and nuclear magnetic resonance (NMR), and the concept of drug-receptor interactions is now universally considered as the basis of the physiologic actions of drugs.
Pharmacodynamics
Drug Binding
Pharmacodynamics is broadly defined as the biochemical and physiologic effects of drugs. Proteins constitute the largest class of drug receptors, but other biomolecules can also be targeted. Proteins and other complex macromolecules can exist in a number of different conformational states. For simplicity, assume just two receptor conformations: physiologically active and inactive. In the case of an ion channel, for instance, the active conformation is an open conformation that allows ion permeation across the membrane, and the inactive conformation is the closed ion channel.
The following equation describes the relationship between the active and inactive states of the receptor (R):
R I ⇄ k i k a R A
R I denotes the inactive (closed) ion channel and R A denotes the active (open) ion channel, and k a and k i are rate constants for the forward and reverse conformational changes, respectively. In this example, rate k i is higher than k a (shown by arrow thickness) to illustrate a situation in which the channel is mostly closed in the absence of drug. The equilibrium relationship between the active and inactive conformations can be written as the ratio of the rate constants:
[ R A ] [ R I ] = k a k i
R I ⇄ k i k a R A + D ⇄ k 2 k 1 R A ∗ D

Although it might seem at first counterintuitive that even in the absence of agonist a receptor can be found in its active form, modern experimental methods such as single-channel patch clamp recordings can show this directly. An example of such a recording is shown in Fig. 1.2 .

This model of drug stabilizing a receptor in its active conformation nicely explains the actions of gamma-aminobutyric acid (GABA) on GABA A receptors (see Fig. 1.2 ), but it is a very simplified view in several ways: (1) receptors can have more than two states (e.g., voltage-gated sodium ion [Na + ] channel). (2) Different conformational states can have different levels of activity rather than the all-or-none view presented here (e.g., nicotinic acetylcholine receptors). (3) Drugs can bind to more than one state of the receptor or at more than one site. However, this model of drug-receptor interactions serves as a foundation for building more sophisticated models. This simplified description is used in the following discussion to derive the basic pharmacologic concepts.
Rearranging the equilibrium expression for drug binding to receptor yields the following expression in which the ratio of the two rate constants is defined as the dissociation constant K D :
[ R ] [ D ] [ R ∗ D ] ≈ k 2 k 1 ≡ K D
Note that if K D is small, then k 1 ≫k 2 and the complex of drug and its receptor is favored (as illustrated in Eq. 3 ). When the converse is true and K D is large, the drug-receptor complex is not favored. Thus K D reflects the propensity of the drug-receptor complex to break down. One can alternatively define affinity as the inverse of K D , which reflects the propensity of the drug to form a complex with the receptor:
A ≡ 1 K D = k 1 k 2

Full access? Get Clinical Tree
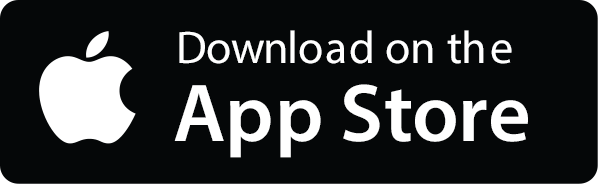
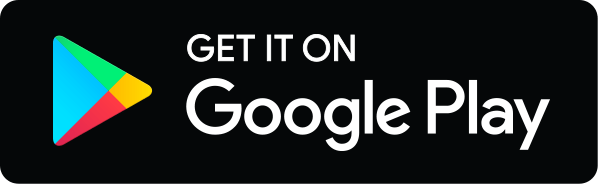