Mechanical Ventilation during Resuscitation: Introduction
Before the arrival of an emergency medical service unit, ventilation given by bystanders must employ techniques that do not require special equipment. Safar, Elam, and Ruben first showed that obstruction of the upper airway by the tongue and soft palate occurs commonly in victims who lose consciousness or muscle tone and that ventilation with manual techniques is markedly reduced or prevented by such obstruction.1–3 Subsequently, Safar et al4,5 developed techniques that prevent obstruction by extending the neck and jaw and applied these in conjunction with mouth-to-mouth ventilation. The “gold standard” today for airway maintenance during resuscitation is intubation of the trachea, which provides a route for ventilation with oxygen, allows suctioning of the upper airway, protects the airway from aspiration of gastric contents, and prevents inflation of the stomach.
Spectrum of Emergency Care Skills
Airway management should be mastered by all properly trained prehospital personnel. This involves both airway assessment and airway control. Compromise of the airway may occur suddenly or slowly and progress over time; therefore, continuous assessment of the airway is vitally important. Pulse oximetry is helpful in identifying hypoxia, although hypoxia may be a relatively late sign of airway compromise. It is therefore important to evaluate breathing pattern, level of consciousness, and shortness of breath continuously.
When endotracheal intubation of children was added to paramedic practice, fifteen of 177 (approximately 8%) children were either intubated esophageally or dislodgment of the endotracheal tube were unrecognized; fourteen of these fifteen children subsequently died.6 Accordingly, invasive pediatric airway equipment was removed from emergency medical service units in Los Angeles County; instead, bag-valve-mask ventilation was recommended. A similarly alarming experience was seen in Orlando, Florida, when the esophagus was intubated in eighteen of 108 (approximately 16%) patients being managed by the emergency medical services.7 This suggests that the skills and experience of a rescuer performing basic and advanced airway management may determine if these maneuvers achieve effective oxygenation and carbon dioxide elimination or result in extremely serious complications, such as severe neurologic impairment, or even death.8
Endotracheal intubation is the “gold standard” for providing emergency ventilation. Thus, every advanced emergency medical service provider must acquire and, especially, maintain intubation skills. Such a goal may be difficult to guarantee because of the large numbers of individuals who require training and/or the infrequent performance of intubations. This experience is similar to observations of the emergency medical services in Houston, Texas, where airway device-related complications were associated more with training with the devices than with the devices themselves.9 This confirms that the success rate of airway management interventions depends on three factors: (a) initial training, (b) continuous quality assurance, and (c) actual frequency of performing the specific intervention. For example, when the actual frequency of performing endotracheal intubation is relatively low, as in the case of the Los Angeles County emergency medical services (e.g., 2584 trained individuals performing 420 actual endotracheal intubations over 33 months), it is not surprising that endotracheal intubation performed by paramedics (who were allowed to perform bag-valve-mask ventilation) did not improve survival or neurologic outcome; instead, it caused some catastrophes.6 In a recent study in Germany, however, trained emergency medical service physicians intubated the esophagus in approximately 7% of all out-of-hospital intubation scenarios.10 These high incidences of intubation disasters even by highly trained rescuers actually may tip the scales toward supraglottic airway devices or bag-valve-mask ventilation for emergency ventilation of patients with a respiratory or even cardiac arrest performed by emergency medical services personnel without excellent intubation skills. Thus, teaching emergency medical services personnel a limited number or even a single airway procedure may follow the axiom “the simpler, the better” and ensure adequate oxygenation and avoid airway-related catastrophes.
Distinction between Airway Protection and Assisted Ventilation
Discussion of whether health care professionals or lay bystanders should or should not perform mouth-to-mouth ventilation has emotional connotations. The question of whether satisfactory lung ventilation in an unintubated cardiac arrest patient can be achieved, however, is clearly a scientific issue. If it is possible to identify ventilation strategies that are beneficial to patients and not harmful, then resuscitation outcomes may be improved.
The distribution of ventilation volume between lungs and stomach in a patient with an unprotected airway depends on factors such as lower esophageal sphincter pressure, airway resistance, and respiratory system compliance.11 Of equal importance are specifics of techniques used for basic or advanced airway support, such as head position, tidal volume, inflation flow rate, and duration, all of which determine upper airway pressure.12,13 The combination of these variables determines gas distribution between the lungs and the esophagus and, subsequently, the stomach. Several fundamental differences exist between respiratory mechanics in a healthy, awake adult, an anesthetized supine patient, and a victim of a cardiac arrest (Table 26-1).
Rescuer | Patient | Conscious | Anesthetized | Cardiac Arrest |
---|---|---|---|---|
Chin support | LESP (cm H2O) | ∼20 to 25 | ∼20 | 5? |
Tidal volume | Crs (mL/cm H2O) | ∼100 to 150 | ∼50 | ∼20 to 50 |
Inflation time | Raw (cm H2O/L/s) | ∼2 to 4 | ∼15 | ? |
Ventilator setting | ||||
Bag-valve-mask size |
Lower esophageal sphincter pressure is the pressure that prevents regurgitation of stomach contents into the pharynx and insufflation of air into the gastrointestinal tract during ventilation. Sphincter pressure in a healthy adult is approximately 20 to 25 cm H2O but may be lower in patients with chronic esophageal reflux disease, during induction of anesthesia, and after insertion of a laryngeal mask airway.14 It is unclear, however, whether these changes in sphincter pressure result from induction of anesthesia. Animal investigations showed that the lower esophageal sphincter pressure deteriorated rapidly from a baseline level of 20 to 5 cm H2O within 5 minutes of untreated cardiac arrest.15,16 This decrease in lower esophageal sphincter pressure was also measured in human subjects following cardiac arrest.17 In a healthy adult, air flows freely from the lips to the alveoli, and therefore, minimal inspiratory pressure is required to move gas during spontaneous ventilation. In a patient with chronic obstructive lung disease, airflow through the respiratory system may be impaired by mucus or airway spasm.18 Data from clinical studies showed an airway resistance of 2 to 4 cm H2O/L/s in healthy volunteers, 8 to 15 cm H2O/L/s in patients with varying degrees of lung disease, and 17 cm H2O/L/s with positive-pressure bag-valve-mask ventilation in a patient during induction of anesthesia.19 In a healthy awake adult, 1 cm H2O of inspiratory pressure moves approximately 100 mL of air into the lungs; compliance decreases to 50 mL in an anesthetized supine adult and may decrease to approximately 20 to 50 mL in a cardiac arrest patient.20 Many factors can change lung compliance. Pulmonary vascular congestion during cardiac arrest increases the volume of the parenchymal interstitium and reduces compliance. Chest compressions and pulmonary edema secondary to left-ventricular failure also contribute to a reduction in compliance.21
Chin support and backward tilt of the head are the two most important maneuvers during rescue ventilation of paralyzed and nonparalyzed patients with an unprotected airway. Moreover, a slight lateral tilt of the head may reduce upper airway obstruction arising from backward relaxation of the tongue and soft tissues.22 The settings of an automatic ventilator or the technique of bag-valve-mask ventilation governs inflation flow rate, inflation time, and tidal volume, all of which affect peak inflation pressure, assuming that there is no significant upper airway obstruction.23 The relationship between peak inflation pressure and lower esophageal sphincter pressure determines gas distribution between the stomach and lungs.24 For example, when peak inflation pressure exceeds sphincter pressure, some air will flow into the stomach; if sphincter pressure is higher than peak inflation pressure, inspiratory air will flow completely into the lungs. The preceding scenario, however, depends on the assumption that the lower esophageal sphincter pressure acts like a mechanical valve, which may not be the case. For example, Safar25 stated that stomach inflation in healthy volunteers was self-limiting, but lower esophageal sphincter pressure was not reported. This observation implies that the physiology of respiratory mechanics in human subjects may be more complex than has been considered previously. Until a better understanding of these mechanisms is obtained, the goal of ventilation with an unprotected airway is to keep it permanently patent and to keep peak inflation pressure to a minimum at all times to prevent stomach inflation.
Stomach inflation is a complex problem that may cause regurgitation,26 aspiration,27 pneumonia,28 and possibly death.29 Stomach inflation will increase intragastric pressure,30 elevate the diaphragm, restrict lung movements, and so reduce respiratory system compliance.31 A reduced respiratory compliance may direct even more ventilation volume into the stomach when the airway is unprotected,10 thereby inducing a vicious cycle with each breath.32 The life-threatening complication of stomach inflation, regurgitation, and subsequent aspiration pneumonia (induced by gastric acid) causes a loss of alveolocapillary integrity and pulmonary surfactant. As a result, fluid and proteins pass into the interstitial spaces, alveoli, and bronchi, causing pulmonary edema, decreased pulmonary compliance, increased lung weight, and significant intrapulmonary shunting or ventilation–perfusion mismatching. Consequently, alveolar gas exchange is impaired markedly. Data from an animal model showed that aspiration of more than 0.8 mL/kg of body weight resulted in 50% mortality. Extrapolation of these results to humans suggest a critical aspiration volume of 50 mL at a pH of 1.33 The results from recent studies of anesthetic practice, however, suggest that the true morbidity from acid aspiration has been exaggerated greatly.34
A maneuver for preventing stomach inflation during ventilation with an unprotected airway is to apply cricoid pressure; this is a simple, effective maneuver to prevent stomach inflation35 that was first described 200 years ago.36 In a model of human cadavers, an intraesophageal pressure of 75 cm H2O was required to overcome cricoid pressure, indicating that the Sellick maneuver may be able to prevent gastric distension even when ventilating with a high peak inflation pressure.37 One study investigating the efficacy and minimum inflation pressure at which gas entered the stomach in pediatric patients found that appropriately applied cricoid pressure was invariably effective in preventing gas insufflation into the stomach in all children with and without paralysis.38 The value of cricoid pressure, however, has been established based on case report series and cadaver and animal studies, and has never been validated in a large, controlled prospective study. Because of the lack of prospective studies, some authors regard the level of evidence for cricoid pressure as V (expert opinion) and the level of recommendation as D (levels being A to D, with A being the highest recommendation level).39 The value of cricoid pressure has been further questioned because of the risk of esophageal rupture when vomiting occurs.40 A further technical limitation is that applying both cricoid pressure and bag-mask ventilation by a single rescuer is nearly impossible and requires a second person to assist.
Moreover, a possible complication of cricoid pressure is airway obstruction.40,41 In fifty-two anesthetized patients, airway obstruction did not occur without cricoid pressure, occurred in one patient (2%) with cricoid pressure of 30 N (3 kg or approximately 6.6 lb), in twenty-nine patients (56%) with cricoid pressure of 30 N (3 kg or approximately 6.6 lb) applied in an upward and backward direction, and in eighteen (35%) patients with cricoid pressure of 44 N (4.4 kg or approximately 9.7 lb).40 These findings were validated in a recent study in children.42 Furthermore, cricoid pressure deforms the larynx and impedes laryngoscopic visibility for intubation and thus may result in more intubation difficulties.43 Finally, modern magnetic resonance imaging has questioned whether cricoid pressure truly closes the hypopharyngeal space or upper esophagus secondary to lateral displacement of the esophagus.44 Thus, in regard of all these limitations it is not surprising that the European Resuscitation Council’s 2010 cardiopulmonary resuscitation (CPR) guidelines stated: “The routine use of cricoid pressure in cardiac arrest is not recommended. If cricoid pressure is used during cardiac arrest, the pressure should be adjusted, relaxed or released if it impedes ventilation or intubation.”45
Role in Different Settings
Securing the airway by tracheal intubation in the prehospital setting may be very difficult. If airway management is difficult in a prehospital patient, maintenance of oxygenation can be in doubt despite use of a bag-valve-mask. Definite securing of the airway can be done later in the emergency department with more experienced personnel and equipment. In acute life-threatening situations, however, a standardized procedure, such as an algorithm, may be fast and reliable (Fig. 26-1).
Figure 26-1
Algorithm for airway management. BURP, backward, upward, rightward pressure maneuver; CT, Combitube; ILMA, intubating laryngeal mask airway; LMA, laryngeal mask airway; LT, laryngeal tube; PTLA, pharyngotracheal lumen airway; PETCO2, end-tidal carbon dioxide; SaO2, arterial oxygen saturation.
Outcome after CPR in adults with chest compression alone was similar to that after chest compressions combined with mouth-to-mouth ventilation; Hallstrom et al. concluded that chest compressions alone may be the preferred approach for bystanders inexperienced in CPR.46 It has been suggested that more lives could be saved if only rapid chest compressions are performed (producing immediate reperfusion of vital organs but with decreased oxygen content) in contrast to the “gold” solution of rapid chest compressions combined with ventilation, which may produce less perfusion but with higher oxygen content.
In a more recent prospective study in Japan, chest-compression-only CPR resulted in increased survival rates in adults.47 Results, however, have been inconsistent in follow-up studies.48,49 In a 2011 study, there seemed to be a time-dependent factor with regards to survival rates: The longer CPR was performed by lay rescuers, the more dependent ventilation became. This is rational from a physiologic viewpoint; at the beginning of a cardiac arrest caused by cardiac disease, there is still enough air in the lungs to enable sufficient oxygen uptake as long as chest compressions are able to deliver any circulatory output. Moreover, directly after cardiac arrest gasping may additionally ventilate the patient’s lungs if the airway is in a patent state.50 This reasoning is in accordance with a study in children where compression-only CPR seemed to be less effective. Because pediatric cardiac arrest contrasts with adult cardiac arrest, being more often caused by hypoxemia than cardiac disease, superiority of ventilation CPR is not surprising in pediatric patients.51 In a metaanalysis, authors detected a potential benefit for compression-only CPR, with a “number needed to treat” of forty-one, based on prospective studies; in contrast, analysis of retrospective studies did not reveal any benefit for chest-compression-only CPR.52 The 2010 CPR guidelines recommend that trained lay rescuers provide ventilation; untrained or unwilling rescuers should provide chest-compression-only CPR.53 Chest compressions and ventilation are performed in a 30:2 ratio (thirty compressions to two ventilations) in a cardiac arrest when the airway is not secured.53 After intubation, ventilation is performed out of synchrony with chest compressions; a respiratory rate of 10 breaths/min is recommended.
One argument that ventilation during CPR could be abandoned is that gasping provides sufficient gas exchange. The value of gasping in human subjects has to be interpreted with caution because fundamental differences in human and animal upper airway anatomy make it difficult to extrapolate results from laboratory studies, where gasping was beneficial. The human upper airway is kinked and is subject to rapid occlusion by the tongue and/or head position in the supine position, whereas the upper airway in swine, dogs, and rats is straight and may not be occluded by the tongue or head position in the supine position.54 In addition, animal studies were performed in fasting animals that, as a consequence of fasting, are extremely unlikely to aspirate gastric contents. Furthermore, it is unknown if humans gasp as frequently or as deeply as animals during cardiac arrest. Whether gasping in humans results in effective gas exchange has not been studied, and for ethical reasons, such a study would be extremely difficult to perform. If a patient is gasping and the rescuer is unwilling or unable to perform mouth-to-mouth ventilation during basic life-support CPR, one strategy is to keep the airway open and perform chest compressions alone until emergency medical services arrive so as to provide a minimum of ventilation.53 In the absence of a dedicated airway, extending the head by placing a pillow under the shoulder or gently tilting it laterally could reduce the acute pharyngeal obstruction from the tongue falling backward over the palate.
Ventilation consumes time during CPR that could be devoted to chest compressions.53 Because only rapid chest compressions are effective, some argue that time spent for ventilation should be decreased and the time for chest compressions increased. Because cardiac output during CPR is at best approximately 25% of normal, some argue that the low cardiac output does not need to be accompanied with a normal minute ventilation. This argument has yet to be proven in a clinical study. Until evidence is available, assisted ventilation should be performed during CPR whenever possible, although the rate of chest compressions should not be decreased critically by ventilation attempts.
Another method, first being described in the late 1990s, is to decrease intrapulmonary pressure by intermittent airway occlusion during the chest-decompression phase. Therefore, a special impedance-threshold valve was designed that is adjusted to a tracheal tube and occludes the airway in the decompression phase.55 This occlusion results in decreased intrapulmonary pressure that enhances venous return to the heart and subsequently increases cardiac output with the next chest compression, improving oxygen transport to vital organs in cardiac arrest. The impedance-threshold valve is especially effective when intrapulmonary pressure is further reduced by active chest decompression using special suction cups attached to the sternum.55 In a clinical study, use of such an impedance-threshold device significantly increased the proportion of patients that “survived to hospital discharge with favorable neurological function” (from 6% to 9%).56 It should be noted, however, that active ventilation is mandatory using the impedance-threshold device, because continuous airway occlusion may otherwise result in atelectasis compromising pulmonary function.57
The underlying principle for care of trauma patients is the ABCs (airway, breathing, circulation) of resuscitation. Initial priorities after trauma are the same for the field and the hospital, but field providers usually have only limited equipment and limited assistance. They also provide care in the least-controlled circumstances, such as the roadside. Airway management is the highest priority and should occur as early as possible; in severe cases, it may be necessary in the field. Physical examination may reveal subcutaneous emphysema, absent breath sounds, and unilateral hyperresonance, identifying a tension pneumothorax, which can be decompressed. Progressive clouding of consciousness indicates nearly always a loss of airway protection, risking aspiration; endotracheal intubation is required. Oral intubation is the most common method because adequately sized tubes can be placed under direct vision. Nasal intubation requires significant expertise, especially when performed blind, and may exacerbate agitation; it is contraindicated in patients with midface fractures. Laryngoscopy in an awake patient nearly always worsens agitation; in these instances, rapid-sequence intubation (RSI) is advised.
RSI involves administration of a sedative followed by a short-acting muscle relaxant, typically succinylcholine. If endotracheal intubation fails, however, the patient must be ventilated with a bag-valve-mask device until short-term paralysis resolves. A retrospective analysis of trauma patients revealed that RSI in the field and in hospital were equally successful (97.9% vs. 98.5%) and safe.58 Other studies reported success rates of 84% to 90.5% for field intubations, suggesting that training and experience are the major determinants of success.59 Failed intubation was associated mainly with inadequate relaxation and difficult anatomy (i.e., morbid obesity or inability to visualize the vocal cords). Although the length of hospital stay and mortality were comparable, pneumonia occurred more frequently with RSI in the field than in hospital.60 In a prospective study of severely injured trauma patients who were intubated in the field via RSI or immediately intubated on admission to the hospital, total out-of-hospital times were twice as high for the field group versus the hospital group (26 vs. 13 minutes).59,61 Patients intubated in the field versus in the hospital had more ventilator, hospital, and intensive care unit days, higher mortality (23% vs. 12.4%), and a 1.5-fold increased risk of nosocomial pneumonia.62 These data argue against spending extra time in the field with severely or even moderately injured patients (injury severity score 15 to 25).63 Even in recently published studies, conducted several years after the problems of high failure rates in out-of-hospital emergency airway management had been identified, the rate of failed intubations is still unacceptably high.64
The high failure rate may indicate that although proper ventilation and perfect intubation skills in the field had been identified as being of utmost importance, it is not possible to achieve in all cases. Rescue personnel must recognize the importance of skill retention. Thus, before intubation attempts, a risk-to-benefit assessment is needed to prevent harm with multiple failed attempts. For example, if conditions are difficult (morbid obesity or midface fractures), it may be prudent to use a bag-valve-mask device and subsequently intubate immediately after arrival in the emergency department. Health care personnel, however, must understand that bag-valve-mask ventilation needs to be performed carefully. In laboratory shock models, excessive ventilation with a bag-valve-mask caused increasing positive intrathoracic pressure, inhibition of venous return, decreased coronary perfusion, and thereby decreased survival.65,66 In contrast, reduced intrapulmonary pressure resulted in better venous return in severe hemorrhagic shock and thus improved blood flow in an animal model comparable to CPR.67 In contrast to the impedance-threshold device during CPR, however, large prospective studies determining the effect of decreased intrapulmonary pressure in shock patients are still lacking.
Cervical spine injuries occur in 2% to 5% of blunt trauma patients,68 and approximately 10% of these are unstable.69 The most common fracture was C2, accounting for 24% of fractures. Dislocations are most common at C5-6 and C6-7.70 Missed cervical spine injury can have disastrous consequences. The incidence of missed or delayed diagnosis is 1% to 5%, and up to 30% of these patients develop secondary neurologic damage.71 The primary objective when managing the airway of such a patient is to minimize neck movement while the airway is secured rapidly and efficiently. Flight paramedics had only a 52% success rate with blind nasal intubation in spontaneously breathing patients in an out-of-hospital setting but were successful with this procedure in fourteen of fifteen patients in the hospital.72 Traumatologists working in a prehospital environment used RSI with a success rate of 97% to 99%.73 When emergency physicians were compared with anesthesiologists using RSI in an out-of-hospital environment, the nonanesthesiologists were twice as likely to fail to intubate and needed to undertake a surgical airway.74
During normal direct laryngoscopy and oral intubation, significant extension occurs between the occiput and C1 and between C1 and C2.75 In an emergency department, the most common approach to intubating the multiple-injury patient with a potential cervical spine injury is RSI while maintaining cricoid pressure and manual inline neck stabilization. Manual inline neck stabilization is provided by an assistant who holds the patient’s mastoid processes firmly down, opposing the upward forces generated during laryngoscopy.76 In anesthetized patients, this maneuver reduced head extension by 50% and should increase the safety of direct laryngoscopy.77 Axial traction must be avoided because excessive distraction may injure the spinal cord. The view at laryngoscopy is better with manual inline neck stabilization than with rigid-collar immobilization78; if a cervical collar is left on during laryngoscopy, the view will be often grade 3 or 4.79 Once manual inline neck stabilization has been applied, the rigid collar should be removed and then reapplied when successful placement of the tracheal tube has been confirmed. Direct laryngoscopy and oral intubation are the quickest method for securing the airway in a patient with potential cervical spine injury.
Many clinicians advocate awake intubation as the safest approach in a patient with a cervical spine injury. It is thought that preservation of muscle tone provides protection, and spinal integrity can be monitored during airway manipulation.80 Prolonged hypotension or malposition after intubation is at least as likely to cause neurologic injury as the intubation itself.81

Full access? Get Clinical Tree
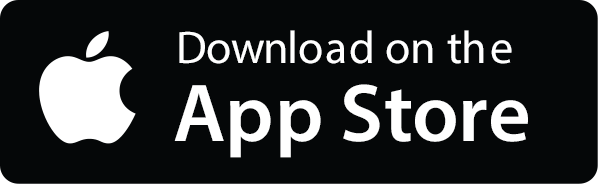
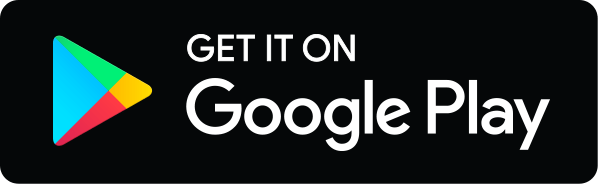
