I. MECHANICAL VENTILATION provides artificial support of gas exchange.
A. Indications
1. Hypoventilation
a. Evaluation: arterial pH should be evaluated for evidence of hypoventilation. Chronic compensated hypercapnia is a stable condition that usually does not require mechanical ventilatory support and therefore arterial partial pressure of carbon dioxide (PaCO2) is not useful in isolation.
b. Hypoventilation resulting in an arterial pH of less than 7.30 is often considered an indication for mechanical ventilation, but patient fatigue and associated morbidity must be considered and may prompt initiation of mechanical ventilation at a higher or lower pH.
c. Noninvasive ventilation (NIV) may be considered for hypoventilation due to chronic obstructive lung disease (COPD).
2. Hypoxemia
a. Supplemental oxygen should be administered to all hypoxemic patients to attain arterial oxygen saturation by pulse oximetry (SpO2) >90% regardless of diagnosis (e.g., appropriate oxygen therapy should not be withheld from hypercapneic patients with chronic obstructive pulmonary disease [COPD]).
b. Patients with hypoxemic respiratory failure due to atelectasis and/or pulmonary edema may benefit from continuous positive airway pressure (CPAP) administered by face mask.
c. Endotracheal intubation and mechanical ventilation should be considered for severe hypoxemia (SpO2 <90% at a fraction of inspired oxygen [FIO2] equal to 1.0) unresponsive to more conservative measures and can be considered earlier for lung protection if ARDS is suspected.
3. Respiratory fatigue
a. Tachypnea, dyspnea, use of accessory muscles, nasal flaring, diaphoresis, and tachycardia may be an indication for mechanical ventilation before abnormalities of gas exchange occur.
4. Airway protection
a. Mechanical ventilation may be initiated in patients who require endotracheal intubation for airway protection, even in the absence of respiratory abnormalities (e.g., increased aspiration risk due to decreased mental status or massive upper GI bleed).
b. The presence of an artificial airway is not an absolute indication for mechanical ventilation (e.g., many long-term tracheostomy patients do not require mechanical ventilation).
B. Goals of Mechanical Ventilation
1. Provide adequate oxygenation and alveolar ventilation.
2. Avoid alveolar overdistension.
3. Maintain alveolar recruitment.
4. Promote patient–ventilator synchrony.
5. Avoid auto-PEEP.
6. Use the lowest possible FIO2.
II. THE VENTILATOR SYSTEM
A. The ventilator is powered by gas pressure and electricity. Gas pressure provides the energy required to inflate the lungs (Fig. 5.1).
1. Gas flow is controlled by inspiratory and expiratory valves. The electronics (microprocessor) of the ventilator controls these valves so that gas flow is determined by ventilator settings.
a. Inspiratory phase: The inspiratory valve controls gas flow and/or pressure, and the expiratory valve is closed.
b. Expiratory phase: The expiratory valve controls positive end-expiratory pressure (PEEP), and the inspiratory valve is closed.
B. The Ventilator Circuit delivers flow between the ventilator and the patient.
1. Due to gas compression and the elasticity of the circuit, part of the gas volume delivered from the ventilator is not received by the patient. This compression volume is typically about 3 to 4 mL for every cmH2O inspiratory pressure—some ventilators compensate for the compression volume while others do not.
2. The volume of the circuit containing gas that the patient rebreathes during the respiratory cycle is mechanical dead space. Mechanical dead space should be as low as possible. This is particularly an issue when low tidal volumes are used.
C. Gas Conditioning
1. Filters may be placed in the inspiratory and expiratory limbs of the circuit.
2. The inspired gas is actively or passively humidified.
a. Active humidifiers pass the inspired gas over a heated water chamber for humidification. Some also use a heated circuit to decrease condensate within the circuit.
b. Passive humidifiers (artificial noses or heat and moisture exchangers) are inserted between the ventilator circuit and the patient. They trap heat and humidity in the exhaled gas and return that on the subsequent inspiration. Passive humidification is satisfactory for many patients, but it is less effective than active humidification, increases the resistance to inspiration and expiration, and increases mechanical dead space.
c. The presence of water droplets in the inspiratory circuit near the patient (or in the proximal endotracheal tube if a passive humidifier is used) suggests that the inspired gas is adequately humidified.
D. Delivery of Inhaled Medications and Gases during Mechanical Ventilation
1. Inhaled medications can be delivered by metered-dose inhaler or nebulizer during mechanical ventilation. Dry-powder inhalers cannot be adapted to the ventilator circuit. Inhaled gases (e.g., inhaled NO) can be added directly to the inhaled gas mixture.
2. A variety of factors influence aerosol delivery during mechanical ventilation (Fig. 5.2).
3. With careful attention to technique, either inhalers or nebulizers can be used effectively during mechanical ventilation.
4. The mesh nebulizer is commonly used because it overcomes issues with the use of jet nebulizers (mostly related to additional gas flow into the circuit) and is cost-effective compared with HFA metered-dose inhalers.
5. Inhaled nitric oxide (NO) can be injected into the ventilator circuit. Mixtures of helium and oxygen (heliox) can also be delivered. However, gases with physical characteristics different from oxygen and air have the potential to interfere with ventilator function.
III. CLASSIFICATION OF MECHANICAL VENTILATION
A. Negative- versus Positive-Pressure Ventilation
1. The iron lung and chest cuirass create negative pressure around the thorax during the inspiratory phase. Although useful for some patients with neuromuscular disease requiring long-term ventilation, these devices are almost never used in the intensive care unit (ICU).
2. Positive-pressure ventilation applies pressure to the airway during the inspiratory phase.
3. Exhalation occurs passively with both positive-pressure ventilation and negative-pressure ventilation.
FIGURE 5.2 Factors affecting aerosol delivery during mechanical ventilation. MDI, metered-dose inhaler. (Modified from Dhand R. Basic techniques for aerosol delivery during mechanical ventilation. Respir Care 2004;49:611–622, with permission.)
B. Invasive versus Noninvasive Ventilation
1. Invasive ventilation is delivered through an endotracheal tube (orotracheal or nasotracheal) or a tracheostomy tube.
2. Although mechanical ventilation through an artificial airway remains the standard in the most acutely ill patients, NIV is preferred in some patients such as those with an exacerbation of COPD, those with acute cardiogenic pulmonary edema, or immunocompromised patients with acute respiratory failure. NIV is also useful to prevent postextubation respiratory failure. There are many patients, however, in whom NIV is not appropriate.
a. NIV can be applied with a nasal mask, oronasal mask, nasal pillows, total face mask, or helmet. Oronasal masks and total face masks are preferred in acutely ill dyspneic patients, in whom mouth leak is often problematic.
b. Although bilevel ventilators are most commonly used for NIV, any ventilator can be used to provide this therapy. Current generation ventilators designed for critical care have both invasive and noninvasive modes, and some have good leak-compensation algorithms.
c. Pressure support is most commonly used for NIV. For bilevel ventilators, this is achieved by setting inspiratory positive airway pressure (IPAP) and expiratory positive airway pressure (EPAP). The difference between IPAP and EPAP is the level of pressure support.
d. An algorithm for use of NIV (including evaluation of contraindications) in the critical care setting is provided in Figure 5.3.
C. Full versus Partial Ventilatory Support
1. Full ventilatory support provides the entire minute ventilation, with little interaction between the patient and the ventilator. This usually requires sedation and sometimes neuromuscular blockade. Full ventilatory support is indicated for patients with severe respiratory failure, patients who are hemodynamically unstable, patients with complex acute injuries while they are being stabilized, and all patients receiving paralysis.
2. Partial ventilatory support provides a variable portion of the minute ventilation, with the remainder provided by the patient’s inspiratory effort. The patient–ventilator interaction is important during partial ventilatory support.
a. Partial ventilatory support is indicated for patients with moderately acute respiratory failure or patients who are recovering from respiratory failure.
1. Advantages of partial ventilatory support include avoidance of muscle weakness during long periods of mechanical ventilation, preservation of the ventilatory drive and breathing pattern, decreased requirement for sedation and neuromuscular blockade, a better hemodynamic response to positive-pressure ventilation, and better ventilation of dependent lung regions.
2. Disadvantages of partial ventilatory support include a higher work of breathing for the patient and difficulty achieving lung protective ventilation if the patient has a strong respiratory drive.
IV. THE EQUATION OF MOTION
A. Delivery of gas into the lungs is determined by the interaction between the ventilator, respiratory mechanics, and respiratory muscle activity, which is described by the equation of motion of the respiratory system:
Pvent + Pmus = VT/C + × R
where Pvent is the pressure applied by the ventilator, Pmus is the pressure generated by the respiratory muscles, VT is tidal volume, C is compliance, V. is gas flow, and R is airway resistance.
1. Pressure generated from either the respiratory muscles (spontaneous breathing), the ventilator (full ventilatory support), or both (partial ventilatory support) applied to the respiratory system results in gas flow into the lungs.
2. For a given pressure, gas flow is opposed by airways resistance and the elastance (inverse of compliance) of the lungs and chest wall.
3. Larger tidal volumes, lower compliance, higher flow, and higher resistance all require a higher pressure.
V. PHASE AND CONTROL VARIABLES
A. The trigger variable starts the inspiratory phase.
1. The trigger variable determines when the ventilator initiates a breath.
2. The ventilator detects patient inspiratory effort by either a pressure change (pressure trigger) or a flow change (flow trigger).
3. The trigger sensitivity is set to both prevent excessive patient effort and avoid autotriggering. Pressure trigger sensitivity is commonly set at 0.5 to 2 cmH2O, and flow trigger sensitivity is set at 2 to 3 L/min.
a. Autotrigger can be caused by artifacts such as cardiac oscillations and leaks. This is corrected by making the trigger less sensitive.
b. Ineffective trigger can be due to neuromuscular disease or auto-PEEP. Switching the trigger variable is rarely effective to deal with failed triggers.
4. Pressure triggering and flow triggering are equally effective if sensitivity is optimized and closely monitored.
B. The control variable remains constant throughout inspiration. Most common of these are volume control, pressure control, and adaptive control (Table 5.1).
1. Volume control. Despite the name, the ventilator actually controls flow (the time derivative of volume) to maintain a constant delivered tidal volume regardless of airways resistance or respiratory system compliance.
a. A decrease in respiratory system compliance or an increase in airways resistance results in an increased peak inspiratory pressure during volume control. Decreased compliance also results in an increased plateau pressure (the pressure during an inspiratory pause when flow is zero).
b. With volume control, the inspiratory flow is fixed regardless of patient effort. This unvarying flow may induce patient–ventilator dyssynchrony.
1. Inspiratory flow patterns during volume control include constant flow (rectangular wave) (Fig. 5.4) or descending-ramp flow (Fig. 5.5).
2. Use of the constant-flow waveform results in a higher peak pressure, which is largely borne by the airways and not the alveoli.
3. Use of a descending-ramp waveform results in maximal flow early in the breath when lung volume is minimal. This reduces peak pressures but decreases expiratory time, which may increase the risk of auto-PEEP and dynamic hyperinflation.
c. The inspiratory time during volume control is determined by inspiratory flow, the inspiratory flow pattern, and tidal volume.
d. Volume control is preferred when an assured minute ventilation or tidal volume is desirable (e.g., avoiding hypercarbia in patients with intracranial hypertension or preventing overdistention in acute respiratory distress syndrome [ARDS]).
2. Pressure control
a. With pressure control (Fig. 5.6), the pressure applied to the airway is constant regardless of the airways resistance or respiratory system compliance.
b. The inspiratory flow during pressure control is exponentially descending and determined by the pressure-control setting, airways resistance, and respiratory system compliance. With low respiratory system compliance (e.g., ARDS), flow decreases rapidly. With high airways resistance (e.g., COPD), flow decreases slowly.
c. Some ventilators allow the adjustment of the rise time, which is the pressurization rate of the ventilator at the beginning of the inspiratory phase (Fig. 5.7). The rise time is the amount of time required for the pressure-control level to be reached after the ventilator is triggered.
A rapid rise time delivers more flow at the initiation of inhalation, which may be useful for patients with a high respiratory drive.
d. Factors that affect tidal volume during pressure control are respiratory system compliance, airways resistance, pressure setting, rise time setting, and the inspiratory effort of the patient. Increasing the inspiratory time will affect tidal volume during pressure-controlled ventilation only if the end-inspiratory flow is not zero. Once the flow decreases to zero, no additional volume is delivered.
e. Unlike volume control, inspiratory flow is affected by patient effort—increased patient effort will increase the flow from the ventilator and therefore delivered tidal volume. This may improve patient–ventilator synchrony, but results in unpredictable tidal volumes and the potential for overdistention.
f. With pressure control, the inspiratory time is set on the ventilator.
1. If the inspiratory time is set to be longer than the expiratory time, pressure-controlled inverse ratio ventilation (PCIRV) results. This strategy has been used to improve oxygenation in patients with ARDS, but has generally fallen out of favor in recent years because it has not been shown to improve patient outcomes and can adversely affect hemodynamics.
2. Pressure control can be desirable for improving patient–ventilator synchrony, but this is controversial. It is also used as an alternative to pressure support when a fixed inspiratory time or rate is desired.
3. Adaptive control
a. With adaptive control, the breath is ventilator- or patient-triggered, pressure-limited, and ventilator- or patient-cycled. With adaptive pressure control, the pressure limit is not constant, but varies breath to breath on the basis of a comparison of the set and delivered tidal volume.
b. Although the ventilator is capable of controlling only pressure or volume at any time, adaptive control combines features of pressure control (variable flow) and volume control (constant tidal volume) (Table 5.1).
c. Pressure-regulated volume control (PRVC on Servo, Viasys), AutoFlow (Draeger), and VC+ (Puritan-Bennett) are trade names that function in a similar manner. With this form of adaptive pressure control, the pressure control level increases or decreases in an attempt to deliver the desired tidal volume.
d. With Volume support (VS), the pressure support level varies on a breath-to-breath basis to maintain a desired tidal volume. If the patient’s effort increases (increased tidal volume for the set level of PSV), the ventilator decreases the support of the next breath. If the compliance or patient effort decreases, the ventilator increases the support to maintain the set volume. This combines the attributes of PSV with the guaranteed minimum tidal volume.
e. The clinical utility of adaptive control is yet to be determined and has potential drawbacks:
1. Tidal volumes may exceed the desired tidal volume if the patient makes vigorous inspiratory efforts, which could result in overdistention lung injury.
2. If the tidal volume exceeds the target volume, the ventilator will decrease the level of pressure support. This may increase the patient’s work of breathing in the setting of increased respiratory demand.
3. If the lungs become stiffer (i.e., compliance decreases) and the tidal volume does not meet the target, the ventilator will increase the pressure, which could result in overdistention lung injury.
4. The choice of volume control, pressure control, or adaptive control usually is the result of clinician familiarity, institutional preferences, and personal bias.
C. Cycle is the variable that terminates inspiration, which is commonly time (volume control or pressure control) or flow (pressure support ventilation).
VI. BREATH TYPES DURING MECHANICAL VENTILATION
A. Spontaneous Breaths are triggered and cycled by the patient.
B. Mandatory Breaths are either triggered by the ventilator or the patient and cycled by the ventilator.

Full access? Get Clinical Tree
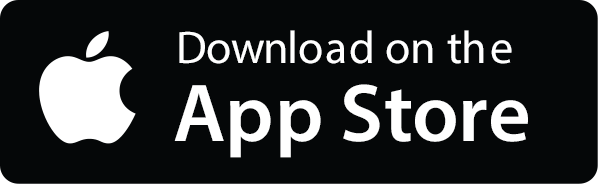
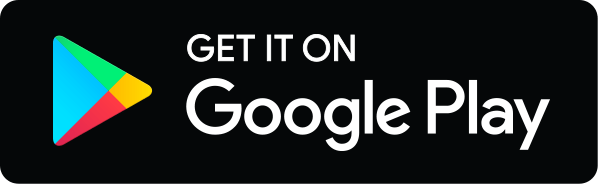