Fig. 37.1
The safe zone of mechanical ventilation. UIP upper inflection point, LIP lower inflection point
37.2 Classification of Mechanical Ventilation
Classifying mechanical ventilators has evolved into a confusing, complex, and frustrating task. There are now more than 170 different names of modes in the United States alone, and it may be expected that more will follow. Many current texts have highlighted and expanded on Robert Chatburn’s previous excellent work to provide a more manageable framework onto which to learn. I will do the same. The interested learner is encouraged to read Chatburn’s work in their entirety [5, 6].
To contrast with this complexity, merely classifying mechanical ventilators into either volume or pressure controlled modes is no longer adequate. Volume control and pressure control can be, however, thought of as the two basic methods of producing positive pressure lung inflation. Newer modes do exist that use a microprocessor to regulate both pressure and volume modes (eg, PRVC- pressure regulated volume controlled), and are termed “dual control modes”. It needs to be emphasized that pressure control and volume control are not modes per se, but rather they simply indicate which variable is held constant during breath delivery.
A more modern approach is to classify ventilators based upon control variables and phase variables. In order to make the concept of control and phase variables more understandable, we need to review the mechanics of breathing. There are four phases of the respiratory cycle:
- 1.
The change from expiration to inspiration
- 2.
Inspiration
- 3.
The change from inspiration to expiration
- 4.
Expiration.
One or more of these four phases of the respiratory cycle is what the ventilator controls or manipulates. Examples of aforementioned control variables include pressure, volume, flow, or time. Phase variables describe how a ventilator starts, sustains, and terminates an inspiration. Phase variables include trigger, limit, and cycle. A mode refers to the manner in which a ventilator breath is triggered, cycled, and limited. The trigger describes how the breath begins (starts inspiration). If the ventilator starts the breath, the trigger is time. If the patient initiates the breath, the inspiration begins when the ventilator detects a pressure or flow change. This would be a pressure trigger and a flow trigger, respectively. The limit describes the variable that controls the size of the breath. The limit is the maximum value a variable (pressure, volume, flow, or time) can reach. Although the limit helps define the range that these variables may reach, it does not end the inspiratory phase. An example is a pressure-limited breath. During a pressure-limited breath a maximum pressure limit is set by the clinician and this value is never exceeded.
37.3 Volume Vs. Pressure Controlled Breathing
In volume control ventilation (VCV) the lungs are inflated at a constant inspiratory flow rate to a preset tidal volume. VCV is also sometimes called volume-limited or volume-cycled ventilation. The advantage to VCV is the ability to deliver a constant tidal volume, and hence minute ventilation (if at a constant respiratory frequency). In the event of increased airway resistance (or decreased compliance), the ventilator will simply increase the inflation pressure to deliver the preset volume. In pressure controlled ventilation (PCV), a pressure target is selected on the ventilator by the physician. The inspiratory flow rate is not constant, but rather gas flow is provided rapidly at first and then exponentially decelerates. Both tidal volume and inspiratory time may vary from breath to breath during a pressure controlled breath. The major benefit during PCV is the ability to control the peak alveolar pressure, and possibly reduce the incidence of VILI. One problem with PCV is that tidal volume is not guaranteed. In the face of increased airway pressures, the delivered minute ventilation may not be adequate.
37.4 Essential Modes of Ventilation
Modes critical for the anesthesiologist to understand include controlled mandatory ventilation (CMV), assist control ventilation (ACV), intermittent mandatory ventilation (IMV), synchronized intermittent mandatory ventilation (SIMV), and pressure support ventilation (PSV or PS). The following paragraphs highlight their similarities and differences.
In controlled mandatory ventilation (◘ Fig. 37.2b) the minute ventilation is entirely determined by the preset respiratory rate and tidal volume. The patient is not able to initiate additional breaths above the set ventilator rate. This mode frequently needs deep patient sedation or even neuromuscular blockade. A potential use may be the brain injured patient where targeted a PaCO2 is easier to obtain with a fixed minute ventilation provided by CMV.
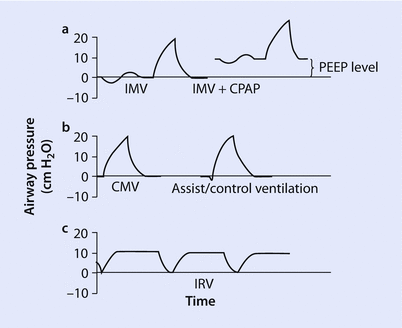
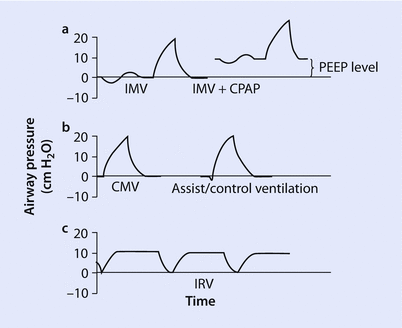
Fig. 37.2
Controlled mandatory ventilation. a Intermittent mandatory ventilation (IMV). b Controlled mandatory ventilation (CMV) and assist control ventilation (ACV). PEEP positive end expiratory pressure, CPAP continuous positive airway pressure, IRV inverse ratio ventilation
Assist control ventilation (◘ Fig. 37.2b) is a time triggered and/or patient triggered form of a CMV mode. The physician sets the tidal volume, minimum respiratory rate, inspiratory flow rate, and sensitivity level. The delivery of the ventilator breath may be either volume controlled or pressure controlled. The key feature is that the ventilator delivers the set tidal volume every time a breath is triggered by the patient. Therefore if a patient develops a rapid respiratory rate minute ventilation can be very high with ACV. In addition to over high minute ventilation, rapid firing of ACV can lead to breath stacking with insufficient time for exhalation. The negative deflection (below the baseline) in ◘ Fig. 37.2b identifies a patient triggered breath. The previous breath in the figure does not have a negative deflection and was generated by the ventilator. If the patient is not breathing spontaneously the ventilator will deliver breaths (at the set tidal volume) at the set minimal respiratory rate (time triggered). A key feature of both ACV and CMV is that all breaths are of equal tidal volumes.
Intermittent mandatory ventilation (◘ Fig. 37.2a) is designed to allow spontaneous breathing between ventilator breaths (unassisted). Similar to CMV and ACV, a set number of mandatory breaths are set on the ventilator. The patient may breath spontaneously between breaths without ventilator support. The tidal volume and frequency are determined by the patient’s respiratory drive and strength. Positive end expiratory pressure (PEEP) has been added to second breath in ◘ Fig. 37.2a; notice how this shifts the baseline airway pressure upward. As long as PEEP is applied the baseline airway pressure never returns to zero. Similar to IMV, synchronous intermittent mandatory ventilation was developed to combat the inherent asynchroncy associated with IMV. SIMV senses patient inspiratory effort and enables a patient to receive a synchronized patient triggered breath. SIMV prevents delivery of larger than intended tidal volumes (breath stacking) by synchronizing patient triggered breaths with mandatory breaths. SIMV has use in the operating room facilitating emergence from anesthesia as patients transition from controlled to spontaneous respirations.
During pressure support ventilation each patient triggered breath is supported by gas flow (this is also called inspiratory flow) to achieve a preset pressure on the ventilator (typically 5–20 cm H2O). The graphical display of PSV is shown in ◘ Fig. 37.3b. Work of breathing is inversely related to the level of PS; the higher the PS the easier it is for the patient to breathe. The ventilator’s inspiratory flow terminates when a certain threshold flow has been reached—typically 25% of the peak flow rate. All breaths are patient initiated, and all breaths are supported. Because the ventilator delivers support only when a patient triggers a breath, appropriate apnea alarms must be present. Most ventilators offer a “backup” rate in case a patient stops breathing. Should over-sedation or prolonged apnea occur the ventilator switches modes to control respiration (CMV for example). PSV is a very interactive mode that allows the patient to determine both the tidal volume and duration of inspiration. PSV has many uses including utilizing low levels of pressure to overcome the resistance to flow in the ventilator circuit (5–10 cm H2O), and at higher levels to increase a patient’s tidal volume (15–20 cm H2O). PSV is frequently combined with continuous positive airway pressure (CPAP) to increase the functional residual capacity. PSV may be instituted during either invasive or noninvasive ventilation.
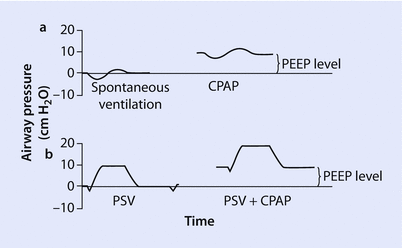
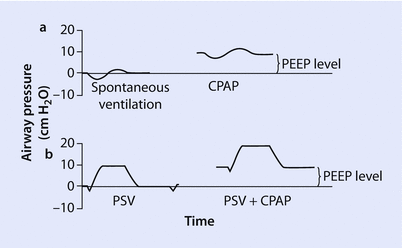
Fig. 37.3
Pressure support ventilation (PSV). CPAP continuous positive airway pressure, PEEP positive end expiratory pressure
37.5 Alternative Modes of Patient Ventilation
A normal I:E ratio during mechanical ventilation is usually set at 1:2 or below (for example 1:2.5) in an adult patient. An I:E ratio of 1:2 represents 1 s spent in inhalation and 2 s spent in exhalation. A shorter inspiratory time encourages lung emptying (because more time will be spent in exhalation), and a longer inspiratory time improves oxygenation. Reversing the normal I:E ratio results in higher airway and alveolar pressures, and is called inverse ratio ventilation (IRV) [7]. Inverse ratio ventilation (◘ Fig. 37.4) is defined as an I:E ratio of >1:1. IRV is not a mode per se, but rather a ventilatory strategy. Inverse ratio ventilation may be either volume cycled (VC-IRV) or pressure cycled (PC-IRV). PC-IRV is used much more commonly and is classified as pressure controlled, time triggered, pressure limited, and a time cycled mode of ventilation. IRV appears to improve oxygenation (primarily through an increase in mean airway pressure), ventilation/perfusion (V/Q) matching, decreased shunting, and reduced dead space (through recruitment of collapsed alveoli) [8].
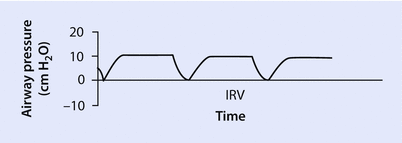
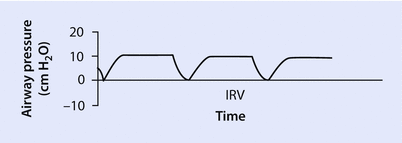
Fig. 37.4
Inverse ratio ventilation (IRV). Note that more time in the breath cycle is spent in inhalation (higher airway pressure) with periods of brief exhalation
IRV is uncomfortable and deep sedation or paralysis is often needed unless the IRV mode also allows spontaneous breathing (as in airway pressure release ventilation [APRV]). APRV is addressed later in this chapter. IRV may also lead to air trapping, and may also result in barotrauma secondary to increased mean airway pressures and auto-PEEP. The higher mean airway pressures seen in IRV may also lead to decreased venous return and decreased cardiac output. The use of inverse ratio ventilation is almost extinct as safer and more accepted methods of mechanical ventilation have prevailed.
37.6 Airway Pressure Release Ventilation (Aprv)
Airway Pressure Release Ventilation (APRV) may be thought of as the application of two alternating levels of CPAP that are applied for set periods of time. APRV has also been called CPAP with release. The patient is allowed to breathe spontaneously during APRV. The variables that must be selected include both the high and low airway pressures and the time spent at each pressure level. The high positive airway pressure is termed P high, and the low pressure is termed P low. Similarly, the time spent at each level is termed T high and T low. Theoretical advantages of APRV include a near complete recruitment of collapsed alveoli and improved oxygenation. The improved oxygenation is accomplished through relatively high mean airway pressures. Recruitment and improved oxygenation are not instantaneous, and quick result should not be expected with this mode of mechanical ventilation. Improvement with APRV may take up to 24 h. Contraindications include severe chronic obstructive pulmonary disease (COPD) and asthma, as APRV allows for very little lung emptying time. Use of APRV under these conditions make patients susceptible to hyperinflation and volutrauma [9].
37.7 High Frequency Ventilation
High frequency ventilation (HFV) is a collection of ventilator modes combining very high respiratory rates (60–3000 breaths per minute) and very low tidal volumes (sometimes smaller than the anatomic dead space). There are four types of HFV: (1) high frequency positive pressure ventilation (HFPPV), (2) high frequency jet ventilation (HFJV), (3) high frequency percussive ventilation (HFPV), and the most commonly employed mode, (4) high frequency oscillatory ventilation (HFOV). These devices combine smaller tidal pressure swings with moderate mean airway pressures. This combination arguably creates the ideal lung protective strategy. Specifically, APRV may lead to reductions in both lung overstretch, and the repetitive closing and opening injuries that conventional ventilation may cause.
High frequency positive pressure ventilation is delivered through a conventional mechanical ventilator at frequencies ranging between 60 and 150 breaths per minute. It is not technically a high frequency mode, as the United States Food and Drug Association (FDA) defines high frequency as any mode that uses >150 breaths per minute. In HFPPV the tidal volumes are small, usually approximately 3–4 mL/kg. The physician controls the respiratory rate, inspiratory flow rate, driving pressure, and PEEP. Expiration is passive, and for this reason there is concern for gas trapping with hyperinflation and volutrauma. HFPPV is rarely used, although it has been used successfully in anesthesia for upper airway surgical procedures and bronchoscopy [10].
High frequency jet ventilation can be used alone or in conjunction with a conventional mechanical ventilator. HFJV utilizes a cannula (14 or 16 gauge) inserted into a standard endotracheal tube or a special triple lumen endotracheal tube. Expiration is passive. The velocity of the expelled gas creates a drag effect to entrain gases along a jet stream. An initial pressure of approximately 35 psi, respiratory rate of 100–150 breaths per minute, and an inspiratory fraction of 20–40% are reasonable settings. The inspiratory fraction is the inspiratory time divided by the respiratory cycle time, and should not exceed 40%. An arterial blood gas should be measured after approximately 15 min to determine if settings should be adjusted. If the PaCO2 is elevated, steps taken should include increasing the driving pressure in 5 psi increments to a maximum of 50 psi, and increasing the inspiratory fraction by 5% (not to exceed 40%). Low PaO2 can be remedied by adding PEEP in 3–5 cm H2O increments and increasing the driving pressure. HFJV is used most commonly in the intraoperative setting for upper airway surgery and tracheal reconstructions. HFJV systems have also been used successfully to provide emergency airway ventilator support by placing a cannula through the cricothyroid membrane. Patients undergoing HFJV require deep sedation and frequently need neuromuscular blockade. A complication specific to HFJV is upper airway trauma including a traumatic necrotizing tracheobronchitis, and injuries related to a dislodged cannula.
High frequency percussive ventilation is a hybrid mode that combines HFV and a conventional mechanical ventilator. A gas driven piston is fitted to the end of an endotracheal tube and generates pressure oscillations of 3–15 Hz. These pressure oscillations are combined in conjunction with a typical pressure targeted breath. There is some data showing improved mucokinesis, potentially improving pulmonary toilet and reducing the requirements for endotracheal suctioning [11]. HFPV has been used most commonly in patients with trauma, burns, and inhalational injuries.
High frequency oscillatory ventilation, as the name implies, uses high frequency low volume oscillations. HFOV is the most common mode of high frequency ventilation. The oscillations create a higher mean airway pressure leading to alveolar recruitment and improved gas exchange. Very small tidal volumes are used (1–2 mL).
A proprietary machine is needed, and such machines are frequently referred to as “oscillators”. The ventilator piston pump oscillates between 3–15 Hz (up to 900 breaths per minute) against a diaphragm that is actively driven both ways. The respiratory rate is so fast the airway pressure is simply oscillating around a constant mean airway pressure. Because the diaphragm has a “to and fro” movement during oscillation, inspiration and expiration are both active components. Active expiration is what separates HFOV apart from other modes of HFV. The active expiration component is advantageous in controlling PaCO2 and in preventing hyperinflation.
Transport of gas during HFOV is thought to occur by five mechanisms [12]:
- 1.
Bulk flow of gas. This is also the delivery method of a conventional ventilator. Gas is delivered to the proximal alveoli with a small contribution going to low dead space volumes.
- 2.
Pendelluft effect is gas mixing between alveolar units where there is transient movement of gas into and out of some alveolar units at the end of inspiration. This gas movement reverses at expiration.
- 3.
Taylor dispersion produces a mixing of fresh gas and residual gas along the front of a flow of gas through a tube.
- 4.
Coaxial flow, where the gas in the center of a column flows inward, while gas on the periphery flows outward.Full access? Get Clinical Tree
