Mechanical Support for Heart Failure
Jeffrey J. Teuteberg
Firas E. Zahr
During the past two decades the incidence of cardiogenic shock has not significantly declined despite important progress in the management of patients with acute myocardial infarction and advanced heart failure [1,2]. Cardiogenic shock is characterized by persistent hypotension with systolic arterial pressures typically less than 80 mm Hg and marked reduction of cardiac index (< 2 L per minute per m2) in conjunction with elevated left ventricular (LV) filling pressures and evidence of end-organ hypoperfusion. Patients may present in shock as a complication of acute myocardial infarction, cardiac surgery, acute myocarditis, or an acute decompensation of chronic heart failure. Although the mortality of patients presenting with acute myocardial infarction and cardiogenic shock declined during the 1990s, the 1-month mortality remains nearly 50% despite aggressive efforts at reperfusion therapy [2,3,4,5]. In many cases of cardiogenic shock, medical therapy alone may be inadequate, and the patient may require temporary or even permanent mechanical support. The proper application of mechanical circulatory support (MCS) requires knowledge of the underlying mechanism of heart failure, understanding of the potential benefits and limitations of both medical and device therapy, familiarity with the full range of devices available for support, and perhaps most critically, careful selection of the appropriate timing for intervention.
Mechanical Circulatory Support
Over the past five decades, mechanical circulatory support technology has evolved substantially from partial temporary support with intra-aortic balloon counterpulsation to a broad array of ventricular assist devices (VADs) capable of providing long-term complete support for one or both ventricles. In the 1990s, extensive experience with bridging patients to transplantation spurred the evolution from bulky extracorporeal devices to smaller, implantable designs, which allowed patients to be discharged from the hospital and have substantial improvements in functional status and quality of life. More recently, the prior generations of larger pulsatile pumps have been superseded by the introduction of smaller, more durable continuous flow devices with superior survival and fewer adverse events.
Benefits of Mechanical Circulatory Support
Hemodynamic
As the left ventricle begins to fail, cardiac output falls and intracardiac filling pressures rise. The main goals of MCS are to decompress the failing ventricle and augment systemic perfusion [6]. Mechanical unloading of the left ventricle leads to a decrease in the severity of mitral regurgitation, less pulmonary congestion, and a reduction in pulmonary arterial hypertension, all of which, in turn, can result in improved right ventricular (RV) function. Partial support pumps provide several liters of flow to augment the reduced native ventricular contribution to the total output, whereas full support pumps provide upwards of 6 to 7 L of flow with the native heart contributing little to the total output. Restoration of forward flow and the normalization of filling pressures also reduces neurohormonal activation, with attendant benefits on cardiorenal function; as a result, temporary VAD support may allow reverse ventricular remodeling and sufficient recovery of ventricular function to permit explantation in selected patients [7].
Biologic
The hemodynamic benefits of mechanical circulatory support with a LV assist device (LVAD) are also associated with favorable structural changes within the cardiac myocytes and extracellular matrix. In studies of isolated human cardiac myocytes, LVAD support increased the magnitude of contraction, shortened the time of peak contraction, and reduced the time to 50% relaxation. In addition, responses to beta-adrenergic stimulation were greater in isolated myocytes after LVAD support. This suggests that mechanical unloading might reverse the downregulation of beta-adrenergic receptors and improve cardiac responsiveness to inotropic stimulation [8,9,10,11,12,13,14,15]. In vivo, mechanical unloading with an LVAD is known to be associated with alteration of gene and protein expression within the cardiac myocyte [16,17], a reduction in nuclear size and DNA content, and a reduction in fibrosis and collagen content within the cardiac extracellular matrix [10,11].
Selection of Appropriate Mechanical Support
The clinical application of MCS grew from early experience with its application as temporary support in the operating room to supporting patients for months until transplant. A broad array of different ventricular support devices is now available (Table 45.1). Broadly speaking, the devices may be configured for isolated right ventricular (RVAD), left ventricular (LVAD), or biventricular (BiVAD) support and for short-term (bridge to recovery or bridge to decision), short-term (bridge to transplant), or long-term (destination therapy) support [18]. Some devices are extracorporeal or paracorporeal in location, with cannulae traversing the skin allowing for inflow and outflow of blood, whereas others are totally implantable with the pump and the cannulae housed in the thoracic and/or abdominal cavity with only a single percutaneous line supplying the power
and providing the connections to the external control systems. Early generation devices were volume displacement pumps, which had a volume chamber sequentially filled and emptied of blood, mimicking the native heart and providing pulsatile flow. However, the need for a volume displacement chamber resulted in a larger pump size and also required more moving parts resulting in mechanical wear and shorter pump life. The current generation of devices no longer has a displacement chamber, but rather has a continuously rotating impeller. This results in a continuous flow of blood and thus limited pulsatility, but allows for substantially smaller pump profiles and longer pump life.
and providing the connections to the external control systems. Early generation devices were volume displacement pumps, which had a volume chamber sequentially filled and emptied of blood, mimicking the native heart and providing pulsatile flow. However, the need for a volume displacement chamber resulted in a larger pump size and also required more moving parts resulting in mechanical wear and shorter pump life. The current generation of devices no longer has a displacement chamber, but rather has a continuously rotating impeller. This results in a continuous flow of blood and thus limited pulsatility, but allows for substantially smaller pump profiles and longer pump life.
Table 45.1 Approved Mechanical Circulatory Support Devices | |
---|---|
|
Cannulation
VADs are typically implanted in parallel with the native right- or left-sided circulation. For long-term LVADs, the pump inflow is from a cannula placed directly into the LV apex and the pump outflow is a cannula that is anastomosed to the
ascending aorta just distal to the aortic valve. Pulsatile systems typically have valves in the inflow and outflow cannulae, whereas continuous flow devices do not. For percutaneous systems, the pumps may be placed across the aortic valve and into the left ventricle or into the left atrium via transcatheter puncture of the interatrial septum. RVADs typically have inflow from the right atrium rather than the RV apex as RV apical cannulation typically provides less reliable flow. The venous blood may also be accessed from the cavae or femoral veins. Outflow is directed to the main pulmonary artery just distal to the pulmonic valve through either direct or transvenous cannulation.
ascending aorta just distal to the aortic valve. Pulsatile systems typically have valves in the inflow and outflow cannulae, whereas continuous flow devices do not. For percutaneous systems, the pumps may be placed across the aortic valve and into the left ventricle or into the left atrium via transcatheter puncture of the interatrial septum. RVADs typically have inflow from the right atrium rather than the RV apex as RV apical cannulation typically provides less reliable flow. The venous blood may also be accessed from the cavae or femoral veins. Outflow is directed to the main pulmonary artery just distal to the pulmonic valve through either direct or transvenous cannulation.
Pulsatile Flow
Early-generation VADs are volume displacement pumps which fill and empty asynchronously with the cardiac cycle creating pulsatile arterial flow. The pulsatile pumps mostly fill passively or have limited ability to augment their filling; thus, the beat-to-beat filling of the pump depends partially on the cardiac cycle. Although most of the blood volume entering the left ventricle is diverted into the pump, the left ventricle does occasionally fill enough to eject and contribute to the total cardiac output. In settings of hypovolemia, the pump will fill less quickly and thus the pump rate will slow down, the converse is true in the setting of hypervolemia, thus maintaining a relatively constant state of decompression of the left ventricle.
Continuous Flow
In contrast to pulsatile flow pumps, continuous flow pumps have a continuously rotating impeller which produces forward flow. The left ventricle is continuously and actively unloaded and therefore the left ventricle rarely can fill to the point where it can eject blood during systole. Thus, the patient has little pulsatile contribution from their native ventricles and hence has little to no pulse pressure, but rather have a mean blood pressure. Patients supported with continuous flow LVADs therefore require Doppler ultrasound to assess their blood pressure. Continuous flow pumps are generally one of two major types: axial or centrifugal flow. Axial flow pumps have the impeller rotating in the same plane as the blood flow, whereas centrifugal pumps accelerate the blood perpendicularly to the axis of inflow. They typically have only one moving part (the impeller) which is magnetically or hydrodynamically suspended resulting in little wear over time. Given the size and wear considerations, among others, continuous flow pumps are now the pump of choice for long-term support. The internal and external components of representative pulsatile and continuous flow pumps are as seen in Figure 45.1.
Most recent data suggests that implantation of a continuous-flow LVAD, as compared with a pulsatile-flow device, significantly improved the probability of survival free of stroke and reoperation for device repair or replacement at 2 years in patients with advanced heart failure in whom medical therapy had failed and who were ineligible for transplantation. In addition, the 2 year actuarial survival with an LVAD was significantly better with a continuous-flow device than with a
pulsatile-flow device. The continuous-flow LVAD was also associated with significant reductions in the frequency of adverse events and the rate of repeat hospitalization, as well as with an improved quality of life and functional capacity [19,20,21,22].
pulsatile-flow device. The continuous-flow LVAD was also associated with significant reductions in the frequency of adverse events and the rate of repeat hospitalization, as well as with an improved quality of life and functional capacity [19,20,21,22].
Extracorporeal Membrane Oxygenation
Extracorporeal membrane oxygenation (ECMO) can provide pulmonary or cardiopulmonary support for up to a week or more. Blood is withdrawn from the circulation via an inflow cannula to an extracorporeal continuous flow pump, an oxygenator, and then back to the patient through an outflow cannula. There are two basic types of ECMO: venovenous (VV) and venoarterial (VA). In VV ECMO, the blood is withdrawn from a large central or peripheral vein (jugular or femoral) and oxygenated blood is returned via another large vein. Thus, VV ECMO does not provide hemodynamic support, but rather pulmonary support. For VA ECMO the inflow is typically via the femoral vein and the outflow is typically through the femoral artery and thus provides both oxygenation and mechanical circulatory support. VA ECMO is most commonly used in the setting of severe shock in the setting of acute infarction, fulminant myocarditis or cardiac arrest or after a failure to wean from cardiopulmonary bypass. In the setting of a failure to wean from bypass, the intraoperative cannulation that was used for cardiopulmonary bypass can be attached to the ECMO circuit, rather than having new cannula placed peripherally. Outside the setting of the operating room, both VV and VA EMCO can be rapidly instituted even at the bedside, as either configuration can be achieved through peripheral access, but should only be performed by experienced personnel.
Complications
Although the focus of this chapter is the preoperative assessment and management of patients being considered for MCS, knowledge of some of the common postoperative complications of MCS are necessary to understand the implications of some of these preoperative considerations. The three most common are bleeding, infection, and thromboembolism.
Bleeding
Placement of an intracorporeal pump requires a sternotomy and cardiopulmonary bypass. The degree of perioperative bleeding can be affected by preexisting coagulopathy, liver congestion, and prior sternotomies or other concomitant corrective surgeries at the time of MCS. Most current-generation devices, whether temporary or permanent, require anticoagulation with heparin after post-operative bleeding subsides and then chronically with warfarin and, depending on the center, an antiplatelet agent(s). Most pulsatile devices have mechanical prosthetic valves requiring an INR of 2.5 to 3.5, whereas some of the current generation continuous flow devices may only require an INR of 1.5 to 2 [23]. Thus, there is a risk of continued or new onset bleeding throughout the duration of support, but current devices have a risk of bleeding requiring transfusion of about 0.85 per patient year beyond 30 days, which is a substantial improvement in comparison to previous generation pulsatile devices [24,25]. However, the continuous flow pumps present a unique risk for gastrointestinal bleeding. The high shear stress on the blood from the impeller can cause destruction of large multimers of von Willebrand factor (vWF), which results in a picture of acquired von Willebrand’s disease [26,27]. Bleeding risk is mostly manifest from gastrointestinal arteriovenous malformation (AVMs), it is unknown if the loss of vWF multimers results in bleeding from pre-existing AVMs or the lack of pulsatile flow predisposes to the development of AVMs [27,28,29]. Although most patients have a demonstrable loss of vWF multimers, only a minority of patients develop bleeding. For those who are awaiting transplant, bleeding requiring transfusion carries the additional risk of sensitization to human leukocyte anitgen (HLA) antigens that may limit the pool of suitable donor organs [30].
Infection
Aside from the infection risks associated with surgery and indwelling lines postoperatively, there is the additional chronic risk associated with the presence of the VAD itself and the associated driveline or cannulae. However, sepsis from any source can result in seeding of interior of the VAD or its components, which may necessitate more urgent and higher risk transplant or even device replacement [31]. Vegetations on LVAD prosthetic valves may also be a source of thromboembolism [32].
Thromboembolism and Stroke
Embolism may result from the pump due to inadequate anticoagulation, the cardiac chambers due to arrhythmias such as atrial fibrillation, or may arise from the native vasculature as a result of the patients’ preexisting vascular atherosclerosis. The overall incidence of ischemic stroke varies greatly with type of device, however with the current generation devices the rate is 0.09 per patient-year overall and 0.05 per patient-year after 30 days [33]. Maintenance of goal INR is critical to minimize the risk of thromboembolism.
Indications
MCS may be appropriate for either short-term (< 1 week) or long-term support of patients with heart failure and shock. In the majority of cases, long-term MCS is intended as a hemodynamic ‘bridge’ to subsequent cardiac transplantation (BTT) [34]. For patients who are not candidates for transplant, an LVAD may be used for long-term support (destination therapy, DT) [19]. The only two devices that have been approved for DT in the United States are the HeartMate XVE, and more recently Thoratec’s HeartMate II LVAD [19,22]. Occasionally, patients are placed on MCS in anticipation of ventricular recovery and device explantation (bridge-to-recovery), as in selected patients with postcardiotomy shock or acute heart failure due to potentially reversible causes (e.g., fulminant myocarditis) [34].
Univentricular Versus Biventricular Support
Selection of the appropriate device for MCS depends initially on the type of support that is required. Most patients presenting with acute heart failure or shock predominantly have LV failure and may be candidates for isolated LV support with an LVAD. Successful LVAD implantation, however, relies heavily on confirmation of adequate native RV function, since RV function is required for LVAD filling [35]. For patients with concomitant, severe RV dysfunction, biventricular support may be necessary. Although recent experience suggests that selected BiVAD patients can be successfully discharged to home, outcomes are generally poorer than with LVAD alone, perhaps in part due to greater severity of illness and end-organ dysfunction amongst patients presenting initially with biventricular
failure [36]. Since BiVAD treatment is currently available only for patients who are candidates for eventual cardiac transplantation, up-front BiVAD support should only be considered in transplant-eligible patients with prolonged shock, giant cell-myocarditis, refractory ventricular tachyarrhythmias, or a high likelihood of postoperative RV failure. Even with the current generation of continuous flow LVADs, there is an approximately 7% incidence of RV failure requiring an RVAD postoperatively, highlighting the need for careful assessment of RV function prior to VAD implantation [37,38,39,40,41,42]. In select centers, explant of the native heart and implantation of a total artificial heart (TAH) may provide an alternative to the use of BiVADs [43].
failure [36]. Since BiVAD treatment is currently available only for patients who are candidates for eventual cardiac transplantation, up-front BiVAD support should only be considered in transplant-eligible patients with prolonged shock, giant cell-myocarditis, refractory ventricular tachyarrhythmias, or a high likelihood of postoperative RV failure. Even with the current generation of continuous flow LVADs, there is an approximately 7% incidence of RV failure requiring an RVAD postoperatively, highlighting the need for careful assessment of RV function prior to VAD implantation [37,38,39,40,41,42]. In select centers, explant of the native heart and implantation of a total artificial heart (TAH) may provide an alternative to the use of BiVADs [43].

Full access? Get Clinical Tree
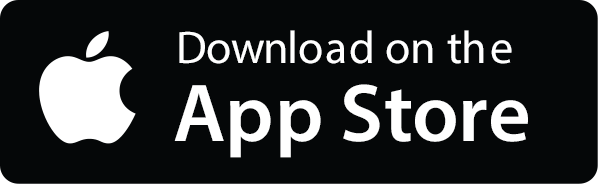
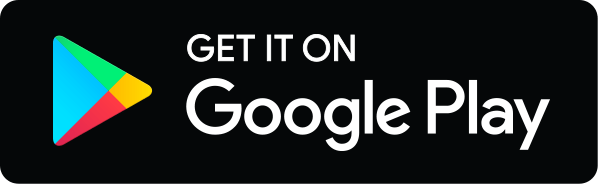