Maternal Physiology
Physiologic Changes during Pregnancy and Delivery
During pregnancy and the peripartum period, substantial changes in maternal anatomy and physiology occur secondary to changes in hormone activity, increased maternal metabolic demands, and biochemical alterations from the fetus and the mechanical effects of an enlarging uterus.
Cardiovascular Changes
Pregnancy-induced changes in the maternal cardiovascular system include increased blood volume and cardiac output, decreased vascular resistance, and supine hypotension.
Intravascular Volumes and Hematology
Maternal intravascular fluid volume begins to increase in the first trimester of pregnancy as the result of increased production of rennin, angiotensin, and aldosterone, which together promote sodium absorption and water retention. These changes are likely induced by progesterone.83 With increases in plasma volume, there is an associated reduction in maternal plasma protein concentration. By term gestation, the plasma volume increases approximately 50%, and the red cell volume increases about 25%. The greater increase in plasma volume is the cause of the “physiologic anemia of pregnancy” (Fig. 45-1).84 Because the increase in red cell mass lags behind the increase in plasma volume, hematocrit typically reaches a nadir in the second trimester and increases by term. Maternal hemoglobin normally remains at 11 g/dL or greater even at term, and lower values at any time during pregnancy represent anemia. The physiologic anemia of pregnancy does not cause a reduction in oxygen delivery because of a coincident increase in cardiac output. The additional intravascular fluid volume (1,000 to 1,500 mL at term) compensates for an average 300 to 500 mL blood loss with vaginal delivery and 800 to 1,000 mL estimated blood loss with cesarean section. Following delivery, uterine contraction creates an autotransfusion of blood often in excess of 500 mL that also compensates for the acute blood loss from delivery. Mild thrombocytopenia is a normal finding.85 However, 8% of otherwise healthy women have thrombocytopenia with a platelet count less than 150,000/mm3. In the absence of other hematologic abnormalities, the cause for gestational thrombocytopenia is a diagnosis of exclusion. Platelet count does not usually drop below 70,000/mm3 and is not associated with abnormal bleeding. Gestational thrombocytopenia is thought to result from a combination of hemodilution and accelerated platelet turnover.

Mild leukocytosis unrelated to infection is common during pregnancy.86 In particular, the neutrophil count increases at term and is further increased during labor. These changes revert to normal during the week after delivery.
In spite of mild thrombocytopenia, pregnancy is a hypercoagulable state with important increases in fibrinogen and factor VII.85 Factor XI and XIII and antithrombin III are decreased and factors II and V typically remain unchanged. These changes result in an approximately 20% decrease in prothrombin time (PT) and partial thromboplastin time (PTT) during a normal pregnancy. One specific type of antithrombin III deficiency (homozygous type II antithrombin deficiency) is a relatively common, genetically determined form of this disorder that is exacerbated during pregnancy and may result in fetal loss, thrombosis, and pulmonary emboli.
Cardiac Output
By the end of the first trimester, maternal cardiac output increases, on average, by 35% above prepregnancy values and continues to increase to 50% above nonpregnant values by the end of the second trimester, remaining at similar, elevated levels throughout the third trimester. This increased cardiac output is the result of both increases in stroke volume and heart rate (Fig. 45-2).87 Labor is associated with further increases in cardiac output, which increases with each uterine contraction. Increases above prelabor values of 10% to 25% are noted during the first stage of labor and a 40% increase occurs during the second stage. The largest increase in cardiac output occurs immediately after delivery, when cardiac output can be increased by 80% to 100% above prelabor values. This large increase is secondary to the autotransfusion from the final uterine contraction, reduced vascular capacitance from loss of the placenta, and decreased lower extremity venous pressure from release of the aortocaval compression. This massive increase in cardiac output represents a moment of unique risk for patients with cardiopulmonary disease, particularly those with valvular stenosis and pulmonary hypertension. Cardiac output returns toward pre-labor values by about 24 hours postpartum and returns to nonpregnant levels within 12 weeks after delivery. It is critical to monitor patients with cardiac disease closely in the postpartum period.

Systemic Vascular Resistance
In spite of increases in cardiac output and plasma volume, systemic blood pressure normally decreases secondary to a 20% reduction in systemic vascular resistance by term. Blood pressure decreases approximately 20% by 20 weeks gestational age and then increases toward nonpregnant values as the pregnancy reaches term. Central venous pressure and pulmonary capillary wedge pressure are unchanged during normal pregnancy despite the increase in plasma volume because of the concurrent increase in venous capacitance.
Aortocaval Compression
In the supine position, blood pressure commonly decreases as the result of aortocaval compression by the gravid uterus. Supine hypotension is manifest by symptoms of diaphoresis, nausea, vomiting, and dizziness. At term, there is almost complete occlusion of the inferior vena cava in the supine position, with return of blood from the lower extremities through the epidural, azygos, and vertebral veins. Vena caval compression may exacerbate lower extremity venous stasis and thereby result in ankle edema, varices, and the risk of venous thromboembolism.
Compensatory adaptations mitigate supine hypotension due to aortocaval compression. One example is a reflexive increase in peripheral sympathetic nervous system activity. This enhanced sympathetic activity increases systemic vascular resistance and maintains systemic blood pressure in spite of reduced cardiac output. Reduced sympathetic tone resulting from neuraxial or general anesthesia will impair this compensatory response and worsen the hypotensive response to supine positioning. A lateral tilt is used to avoid the hypotension that can be associated with supine positioning with neuraxial techniques for labor analgesia and operative deliveries.
The earlier described cardiovascular changes of a pregnancy produce significant changes in the normal cardiac exam. Auscultation reveals an accentuated first heart sound (S1) with an increased splitting of the first heart sound caused by dissociated closure of the tricuspid and mitral valves. A third heart sound (S3) is often heard in the final trimester and a fourth heart sound (S4) can also be heard in a minority of pregnant patients as a result of increased volume and turbulent flow. A mild systolic ejection (grade 2/6) murmur is commonly noted over the left sternal border and is secondary to mild regurgitation at the tricuspid valve from increased cardiac volume.
Pulmonary Changes
Pregnancy results in significant alterations in the upper airway, lung volumes, minute ventilation, oxygen consumption, and metabolic rate.
Airway
During pregnancy, there is vascular engorgement with friability and edema of the mucosal lining of the oro- and nasopharynx. As a result, there is danger of bleeding with instrumentation of the airway and increased risk of difficult ventilation and intubation. Attempts at laryngoscopy should be minimized and a smaller size cuffed endotracheal tube (6.0- to 6.5-mm internal diameter) should be considered to avoid airway edema and bleeding. Airway edema can be particularly problematic in the setting of preeclampsia, upper respiratory tract infections, and after prolonged pushing due to the associated increases in venous pressure. In addition, pregnancy-associated weight gain, particularly in women of short stature or with preexisting obesity, can exacerbate difficult laryngoscopy because of a shorter neck and heavy breast tissue. The patient’s position should be optimized and all necessary equipment for airway management should be available before attempts are made at intubation.
Minute Ventilation and Oxygenation
In order to accommodate the increased oxygen demand and carbon dioxide production of the growing placenta and fetus, minute ventilation is increased 45% to 50% above nonpregnant values during the first trimester and remains at this increased level for the remainder of the pregnancy. This greater minute ventilation is attained primarily as a result of a greater tidal volume with a small increase in the respiratory rate (Fig. 45-3).88 Maternal PaCO2 is commonly reduced from 40 mm Hg to approximately 30 mm Hg during the first trimester. Arterial pH, however, is only slightly increased (pH 7.42 to 7.44) because of metabolic compensation from increased renal excretion of bicarbonate (HCO3− is typically 20 or 21 mEq/L at term). During the first trimester, maternal PaO2 may be above 100 mm Hg due to hyperventilation and decreased alveolar CO2. Later, PaO2 becomes normal or even slightly decreased, most likely reflecting small airway closure with normal tidal volume ventilation and intrapulmonary shunt. Arterial oxygenation can be significantly improved by changing position from supine to lateral. Maternal hemoglobin is right-shifted with the P50 increasing from 27 to approximately 30 mm Hg (Fig. 45-4).89 The higher P50 in the mother and lower P50 in the fetus favors off-loading of oxygen across the placenta. At term, oxygen consumption is increased by 20%. During the first stage of labor, oxygen consumption increases above pre-labor values by 40% and during the second stage it is increased by 75%. In the absence of analgesia, the pain of labor can result in severe hyperventilation causing PaCO2 to decrease below 20 mm Hg.


Lung Volumes
During pregnancy, the growing uterus elevates the diaphragm and causes a reduction in functional residual capacity by 20% at term. This reduction in functional residual capacity is a result of equal reduction in both the expiratory reserve volume and residual volume (see Fig. 45-3). The closing capacity remains unchanged. The reduced ratio of functional residual capacity to closing capacity favors small airway closure with reduced lung volumes and in the supine position causing atelectasis. Vital capacity is not significantly changed with pregnancy. The combination of increased minute ventilation and decreased functional residual capacity results in a greater rate at which changes in the alveolar concentration of inhaled anesthetics can be achieved with spontaneous ventilation in the case of mask induction.
During induction of general anesthesia in a pregnant patient, desaturation occurs more rapidly than in a nonpregnant patient because of decreased functional residual capacity and increased metabolic rate. Administration of 100% oxygen prior to the induction of general anesthesia is critical to allow as much time as possible for safe airway management. Inhalation of 100% oxygen for 3 minutes or, in an emergency, 4 maximal breaths over the 30 seconds will significantly prolong the period between apnea and arterial oxygen desaturation.
Gastrointestinal Changes
After midgestation, pregnant women are thought to be at increased risk of aspiration pneumonia with administration of general anesthesia. This increased risk is caused by several factors. There is upward movement of the esophageal sphincter by the gravid uterus. Increased progesterone and estrogen concentrations during pregnancy also contribute to reduced esophageal sphincter tone. Gastrin is secreted by the placenta which increases gastric acid secretion. Together, these changes result in an increased incidence of gastroesophageal reflux during pregnancy. Gastric emptying is not changed during pregnancy before the onset of labor in women who are of normal weight or obese.90,91 However, during labor, pain, anxiety, and the administration of opioids (including those administered neuraxially) decrease gastric emptying. An increase in the residual volume of gastric content can further increase the risk of aspiration, which is already elevated during pregnancy.92 As a result, all women in labor are considered to have full stomachs and to be at increased risk for pulmonary aspiration with induction of anesthesia. To reduce the risk of aspiration, administration of a nonparticulate antacid, rapid sequence induction with cricoid pressure, and placement of a cuffed endotracheal tube are all commonly a routine part of induction of general anesthesia in pregnant woman past midgestation. While use of an antacid reduces the risk of severe sequelae of aspiration by raising the pH of the gastric content, cricoid pressure is only of theoretical benefit and has not been proven effective in preventing aspiration. Significant pressure needs to be applied in order to compress the esophagus. Multiple attempts at airway manipulation have been associated with aspiration and if good intubating conditions are not possible with cricoid pressure, it is reasonable to release pressure and reposition the head to improve intubating conditions prior to additional attempts at intubation.
While blood flow to the liver does not change during pregnancy, markers of liver function including aspartate aminotransferase (AST), alanine aminotransferase (ALT), and bilirubin all increase to the upper limits of normal. Alkaline phosphatase levels more than double secondary to placental production of this enzyme. Hemodilution during pregnancy is responsible for lower plasma protein concentrations. Lower serum albumin concentrations can result in elevated free blood levels of highly protein-bound drugs. Plasma cholinesterase (pseudocholinesterase) activity is decreased about 30% from the 10th week of gestation up to 6 weeks postpartum, but this decreased cholinesterase activity is not associated with clinically relevant prolongation of neuromuscular blockade from succinylcholine or mivacurium in patients with normal baseline levels of the enzyme. Gallbladder disease is common during pregnancy due to incomplete gallbladder emptying and changes in bile composition.
Renal Changes
Renal blood flow and the glomerular filtration rate are increased 50% by the second trimester and remain elevated until 3 months postpartum. As a result, clearance of creatinine, urea, and uric acid are increased during pregnancy. Increased urine protein and glucose result from decreased renal tubular resorption capacity. The upper limit of the normal 24-hour urine elimination during pregnancy is 300 mg of protein and 10 g glucose.
Neurologic Changes
Pregnant patients are more sensitive to both inhaled and local anesthetic agents. MAC is reduced by 30% by the first trimester of pregnancy. MAC or immobility in response to volatile anesthetics occurs at the level of the spinal cord. A recent electroencephalographic study suggests that anesthetic effects of sevoflurane on the brain are similar in the pregnant and nonpregnant state.93 Fortunately, anesthetic concentrations required for hypnosis and amnesia are approximately half of MAC or the concentration required to prevent activation of spinal reflexes. Unanticipated awareness is more common during cesarean section. The reasons are likely multifactorial. There is often a desire to prevent anesthetic exposure to an already depressed fetus and the requirement for rapid surgery to allow for neonatal resuscitation may not allow the anesthetic to come to steady state before surgery begins.
Pregnant women are more sensitive to the local anesthetics and a lower dose is able to obtain the same level of either spinal or epidural neuraxial blockade compared to nonpregnant women. At term, distention of epidural veins decreases the size of the epidural space, and volume of cerebrospinal fluid (CSF) in the subarachnoid space. CSF pressure is not increased during the course of pregnancy until labor, when it is increased both during uterine contractions and expulsion of the fetus. Although the decreased volume of these spaces may facilitate spread of local anesthetics, the decreased local anesthetic required during pregnancy occurs as early as the first trimester, before mechanical or pressure related changes occur. As such, hormonal changes likely play a role in causing increased nerve sensitivity to local anesthetics.
Uteroplacental Physiology
The placenta is composed of both maternal and fetal tissues and is the interface of maternal and fetal circulation systems. It provides a substrate for physiologic exchange between mother and fetus without immunologic rejection. Maternal blood is delivered to the placenta by the uterine arteries and enters the intervillous space via the spiral arteries. The deoxygenated fetal blood arrives at the placenta via two umbilical arteries that form umbilical capillaries that cross the chorionic villi. Following placental exchange, oxygenated, nutrient-rich and waste-free blood is returned from the placenta to the fetus through a single umbilical vein (Fig. 45-5).

Uterine Blood Flow
An understanding of uteroplacental blood flow is important for the anesthesiologist caring for a pregnant patient. Uterine blood flow increases progressively during pregnancy from about 100 mL per minute in the nonpregnant state to 700 mL per minute (about 10% of cardiac output) at term gestation. Uterine blood flow has minimal autoregulation and the vasculature remains essentially fully dilated during normal pregnancy. Uterine and placental blood flows are dependent on maternal cardiac output and are directly related to uterine perfusion pressure. Decreased perfusion pressure can result from maternal hypotension secondary to hypovolemia, aortocaval compression, or decreased systemic resistance from either general or neuraxial anesthesia. Increased uterine venous pressure can also decrease uterine perfusion. This can occur from supine positioning with vena caval compression, frequent or prolonged uterine contractions, or significant prolonged abdominal musculature contraction (Valsalva) during pushing. Additionally, extreme hypocapnia (PaCO2 <20 mm Hg) associated with hyperventilation secondary to labor pain can reduce uterine blood flow with resultant fetal hypoxemia and acidosis. Neuraxial blockade does not alter uterine blood flow as long as maternal hypotension does not occur.
Endogenous maternal catecholamines and exogenous vasopressors may cause an increase in uterine arterial resistance and a decrease in uterine blood flow, depending on the type and dose given. In early studies in pregnant ewes, ephedrine was found to have no effect on uterine blood flow despite drug-induced increases in maternal arterial blood pressure, whereas other vasopressors including phenylephrine resulted in vasoconstriction and fetal acidosis.94,95 From this animal data, ephedrine was long considered the vasopressor of choice for the treatment of hypotension caused by the administration of neuraxial anesthesia to pregnant women. In complete contrast, however, clinical trials demonstrate the use of phenylephrine is not only effective in preventing hypotension but is associated with less fetal acidosis and base deficit than the use of ephedrine.96,97 Thus, many clinicians have now switched from ephedrine to phenylephrine as the vasopressor of first choice for treating hypotension during labor and delivery.
Oxygen Transfer
The delivery of oxygen from the mother to the fetus is dependent on a variety of factors including placental blood flow, the oxygen partial pressure gradient between the two circulations, the diffusion capacity of the placenta, the respective maternal and fetal hemoglobin concentrations and oxygen affinities, and the acid–base status of the fetal and maternal blood (Bohr effect). The fetal oxyhemoglobin dissociation curve is left-shifted (P50 = 19 mm Hg, greater oxygen affinity), whereas the maternal oxyhemoglobin dissociation curve is right-shifted (P50 = 27 mm Hg, less oxygen affinity). This occurs because fetal hemoglobin does not interact with 2,3-disphosphoglycerate (2,3-DPG), facilitating oxygen transfer to the fetus (see Fig. 45-4). Fetal PaO2 is normally 40 mm Hg and does not exceed 60 mm Hg even if the mother is breathing 100% oxygen. This is because significant oxygen has been extracted by the mother’s tissues prior to arrival at the fetoplacental unit. Carbon dioxide easily crosses the placenta and is limited by blood flow and not diffusion.
Fetal Heart Rate Monitoring
After 18 weeks, fetal heart rate monitoring is practical, and after 25 weeks, variability in fetal heart rate is a reliable sign of well-being. The American College of Obstetrics and Gynecology states, “although there are no data to support specific recommendations regarding non-obstetric surgery and anesthesia in pregnancy, it is important for non-obstetric physicians to obtain obstetric consultation before performing non-obstetric surgery. The decision to use fetal monitoring should be individualized and each case warrants a team approach for optimal safety of the woman and her baby.”98 Under general anesthesia and with the use of opioids and maternal cooling, the loss of fetal heart rate variability may not be indicative of fetal acidemia but may be a result of anesthetic alteration of autonomic tone. Fetal bradycardia is more concerning but can also be affected by hypothermia, maternal acidosis, or the maternal administration of drugs such as β blockers that cross the placenta and reduce fetal heart rate.
Pain Management
When nonobstetric surgery is conducted under regional anesthesia, effective analgesia can be provided by administering a long-acting narcotic neuraxially or by continuation of an epidural infusion postoperatively. Systemic opioids including those used in patient-controlled intravenous analgesia cross the placenta and may reduce fetal heart rate variability. However, there is no evidence that this is detrimental to the fetus. If the fetus is born prematurely shortly after exposure to maternal systemic opioids, reversal with naloxone and/or respiratory support may be necessary. Maternal pain control after nonobstetric surgery is of paramount importance to maternal and fetal well-being. Nonsteroidal antiinflammatory drugs, although useful adjuvant analgesics outside of pregnancy, should be used with caution in pregnancy. They are associated with an increased risk of miscarriage and fetal malformation when used in early pregnancy and premature closure of the ductus arteriosus and oligohydramnios when used after 30 weeks’ gestation. Acetaminophen is generally accepted as safe in pregnancy.
Following surgery, both the fetal heart rate and uterine activity should be evaluated. Preterm labor can be managed with appropriate tocolytic drugs. Postoperative pain medications may make it difficult for the patient to note early contractions and patient perception should not be considered a substitute for standard monitoring. In addition, venous thrombosis prophylaxis should be instituted unless surgically contraindicated.
Fetal Physiology
Characteristics of the Fetal Circulation
Approximately two-thirds of the fetal-placental blood volume is contained within the placenta. The fetal blood volume increases throughout gestation. During the second and third trimester, the fetal blood volume has been estimated to be approximately 120 to 160 mL/kg of fetal body weight.
The fetal blood passes from the placenta through the umbilical vein (see Fig. 45-5).99 Approximately one-third of the blood volume passes through the ductus venosus to the inferior vena cava. The rest of the blood volume passes to the fetal liver, joins the portal vein, and passes to the right atrium. As a result, drugs and toxic substances are detoxified by the fetal liver prior to exposure to the fetal brain and heart. The fetal circulation is characterized by high pulmonary vascular resistance, low systemic vascular resistance (including the placenta), and right-to-left cardiac shunting via the foramen ovale and ductus arteriosus. As such, the majority of the blood bypasses the fetal lungs. The blood is pumped from the fetal left ventricle through the body to the internal iliac arteries where it passes through the umbilical arteries back to the placenta to be detoxified and reoxygenated.
Drug Transfer
Maternal-fetal exchange of most drugs and other substances with molecular weights of less than 1,000 Daltons occurs primarily by diffusion. The rate of diffusion and peak levels in the fetus depend on maternal-to-fetal concentration gradients, maternal protein binding, molecular weight of the substance, lipid solubility, and the degree of ionization of that substance. The maternal blood concentration of a drug is normally the primary determinant of how much drug will ultimately reach the fetus. The high molecular weight and poor lipid solubility of nondepolarizing neuromuscular blocking drugs result in minimal transfer of these drugs across the placenta.16–23 Succinylcholine has a low molecular weight but is highly ionized and therefore does not readily cross the placenta unless given in very large doses.13–15 Thus, during administration of a general anesthetic for cesarean delivery, the fetus/neonate is not paralyzed. If paralysis is desired, for example, during fetal surgery, muscle relaxants must be injected directly into the umbilical vein. Both heparin and glycopyrrolate have minimal placental transfer because they are highly charged.52 Placental transfer of volatile agents,24–30 benzodiazapines,2,57–60 local anesthetics,42–51 and opioids31–41 is facilitated by the relatively low molecular weights, neutral charge and relative lipophilicity of these drugs.
Fetal blood is more acidic than maternal and the lower pH creates an environment where weakly basic drugs such as local anesthetics can cross the placenta as a nonionized molecule and become ionized in the fetal circulation. Because the newly ionized molecule has more resistance to diffusion back across the placenta, the drug can accumulate in the fetal circulation and reach levels higher than the maternal blood. This process is named “ion trapping.” During fetal distress (lower pH in the fetal circulation), higher concentrations of weakly basic drugs, such as local anesthetics, can be trapped. High concentrations of local anesthetics in the fetal circulation decrease neonatal neuromuscular tone. Extremely high levels such as those associated with unintended maternal intravascular local anesthetic injection result in a variety of fetal effects that include bradycardia, ventricular arrhythmias, acidosis, and severe cardiac depression.
Fetal Liver Function and Drug Metabolism
Although fetal liver function is not yet mature, coagulation factors are synthesized independent of the maternal circulation. These factors do not cross the placenta and their serum concentrations increase with gestational age. However, fetal clot formation in response to tissue injury is less robust in comparison to adults. The anatomy of the fetal circulation helps to decrease fetal exposure to potentially high concentrations of drugs in umbilical venous blood. Approximately 75% of umbilical venous blood initially passes through the fetal liver, which may result in significant drug metabolism by the fetal liver before the drug reaches the fetal heart and brain (first-pass metabolism). Fetal/neonatal enzyme activities are less developed than those of adults, but most drugs that cross the placenta can be metabolized. In addition, drugs entering the fetal inferior vena cava via the ductus venosus are initially diluted by drug-free blood returning from the fetal lower extremities and pelvic viscera of the fetus. These anatomic characteristics of the fetal circulation markedly decrease fetal plasma drug concentrations compared to maternal concentrations.
Anesthetic Toxicity in the Fetus
All general anesthetic drugs cross the placenta. While there is no clear evidence for toxicity of specific anesthetic drugs in humans, there is concerning animal data in rodents and primates that suggest that prolonged exposure to general anesthetic drugs including inhaled anesthetics,100 propofol,101 and ketamine102 may induce inappropriate neuronal apoptosis that is associated with long lasting behavioral abnormalities. Although these preclinical results are concerning, it is not clear whether or when these drugs might cause toxicity in humans. The critical period of rapid synaptic development is extended in humans from the prenatal period through 2 years of postnatal life. Analyses of existing human data have yielded conflicting results. In population-based cohorts evaluating the effect of general anesthesia before birth, there was a suggestion that multiple exposures increase the risk for learning disability.103 The results of these types of studies are difficult to interpret because the reason for the anesthesia cannot be separated for the impact of the anesthesia itself. Also, the timing, type, and duration of anesthesia were not considered. Changes in practice have not been recommended in the absence of any anesthetic regimen that has been proven to provide more safety than others.
Studies have found an excess of birth defects in women who underwent general anesthesia during pregnancy but most have found a small increase in the risk of preterm delivery or miscarriage during the first trimester. In general, the second trimester is preferred for surgical intervention as this is a period after much organogenesis has taken place and yet minimizes the risk of preterm labor associated with the third trimester. Monitoring for contractions is recommended and in some situations suppression with magnesium is recommended after surgery. As the long-term impact of general anesthesia on the fetus is unknown, regional anesthesia is favored when possible for the surgical procedure but should not be undertaken unless both the anesthesiologist and surgeon are experienced in using the technique for a given procedure.
Fetal Neurophysiology
Fetal Pain
The gestational age at which the fetus can feel pain is highly controversial. The experience of pain requires two conditions: (a) nociception and (b) perception with emotional response. Afferent sensory fibers required for nociception are in place and a functional spinal reflex is present by 20 weeks’ gestation (Fig. 45-6).104 The second requirement for the experience of pain, perception with emotional response, is much more difficult to establish. In the verbal patient, pain is established by self-report. In the nonverbal, it is measured with observation of complex behaviors thought to be representative of emotional response such as grimace. Neither measurement is practical or necessarily representative in the fetus because of coincident development of motor and intermediary circuitry.


Full access? Get Clinical Tree
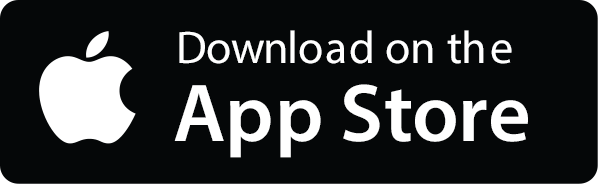
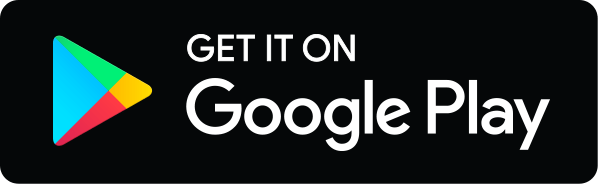