Management of the Postoperative Cardiac Surgical Patient
Sajid Shahul
Cathy Dudick
Alan Lisbon
The management of the postoperative cardiac surgical patient is a dynamic process that requires modern intensive care unit (ICU) technology and sharp clinical skills. Early detection of acute complications has a significant impact on morbidity and mortality. The postoperative care of cardiac surgical patients is best handled using a systematic approach [1,2].
Monitoring
The restoration and maintenance of physiologic homeostasis without further injury to the heart and other organs represent the most important goal in the care of the postoperative cardiac surgical patient and requires proper patient monitoring. An arterial cannula, usually in the radial artery, permits easy access to blood for various laboratory tests (see Chapter 3) and provides the ability to measure systemic blood pressure continuously, mean arterial pressure (MAP) being the value of most interest. The MAP is the least dependent on site or technique of measurement and the least affected by measurement damping; it also determines tissue blood flow by autoregulation [3].
At least one lead of the surface electrocardiogram also should be displayed, with several leads being monitored for ST-segment changes. Pulse oximetry allows assessment of oxygen saturation and reduces the need for arterial blood gases.
A triple-lumen pulmonary artery catheter (PAC) inserted through an internal jugular vein permits measurement of the right atrial, pulmonary artery, and pulmonary artery occlusion (PAOP) pressures and the determination of cardiac output (CO) and mixed venous saturation. Pulmonary artery catheters with an oximeter probe at the distal end allow continuous monitoring of mixed venous oxygen saturation and cardiac index. However, based on multiple, randomized controlled clinical trials in a variety of settings, the routine use of pulmonary artery catheterization does not lead to improved clinical outcomes [4,5,6,7,8,9]. Although the PAC-Man trial, an open randomized trial involving 65 UK ICUs and over 1,000 patients, demonstrated no clear benefit or harm in using a PAC [4], the use of a PAC carries attendant risks such as infection, pulmonary artery rupture, and arrhythmia.
Transesophageal echocardiography is now used both as a monitoring and a diagnostic tool, both in the operating room and the ICU. It allows real-time evaluation of intracardiac blood flow, anatomy, and function. It may be superior to invasive monitoring [10], particularly in the setting of valvular disease or respiratory disease when pressure-based readings may not accurately reflect volume status. In both cardiac and noncardiac patient populations, several studies demonstrated that TEE provided unexpected information that significantly altered the therapeutic plan, even in patients with an indwelling PAC [10]. The therapeutic management decisions gleaned from TEE ranges from 10% to 69%, with the majority of studies demonstrating the 60% to 65% range. The diagnostic yield of TEE approaches 78% [11].
Initial Assessment
A brief but systematic physical examination of the patient is mandatory on arrival in the ICU. Inspection of the skin and extremities may reveal intraoperative injuries, infiltration or disconnection of intravenous (IV) infusions, absence of pulses, signs of drug or transfusion reactions, or evidence of hypoperfusion. Auscultation of the chest may reveal unilateral absence of breath sounds due to malposition of the endotracheal tube or pneumothorax. The abdomen should be inspected to ensure that no abdominal distention is present. Mediastinal and chest tubes should be examined for drainage.
Initial laboratory studies should include arterial blood gas, hematocrit, sodium, potassium, glucose, calcium, magnesium, prothrombin time (PT), partial thromboplastin time (PTT), and platelet count. A portable chest radiograph and a 12-lead electrocardiogram with atrial electrograms should be obtained immediately on admission to the ICU. The postoperative chest radiograph should be inspected with specific attention to the following: (a) pneumothorax and mediastinal shift; (b) position of the endotracheal tube, nasogastric tube, and intravascular catheters; (c) size and contour of the mediastinal silhouette; and (d) pleural and extrapleural fluid collections.
Physiologic Principles of Cardiac Function
Cardiac function is determined by intrinsic myocardial properties as well as by ambient loading conditions. The inotropic state (contractility) of the myocardium during systole is a determinant of systolic stroke volume (SV). Systolic function is also determined by ambient hemodynamic conditions (heart rate [HR], preload, and afterload). The conceptual framework that provides maximal information about intrinsic myocardial properties, as well as the interrelationships between systolic contractility, preload, and afterload, is represented by the ventricular pressure–volume (PV) relationship (Fig. 148.1). The cardiac cycle has four phases: (a) passive ventricular filling during diastole (which, in Fig. 148.1, has been extended as a curvilinear line to describe the distensibility of the ventricle beyond the range of the illustrated cardiac cycle), (b) isovolemic systole (before aortic valve opening), (c) systolic ejection, and (d) isovolemic relaxation.
The SV for an individual cardiac cycle can be obtained by subtracting end-systolic ventricular volume from the end-diastole volume (EDV). The systolic ejection fraction can be determined from the fractional relationship between SV and EDV. This framework aids in conceptualizing and predicting the effects of changes in loading conditions and contractility on measurable hemodynamic parameters.
Left atrial pressure can be measured, or its mean can be estimated by the measurement of PAOP pressure or pulmonary diastolic pressure. These three pressures are equal only under
ideal circumstances. Generally, pulmonary diastolic pressure exceeds pulmonary artery occlusion pressure, which exceeds mean left atrial pressure. These differences are determined by gravitational effects related to pulmonary artery catheter position and by diastolic pressure gradients in the pulmonary vasculature.
ideal circumstances. Generally, pulmonary diastolic pressure exceeds pulmonary artery occlusion pressure, which exceeds mean left atrial pressure. These differences are determined by gravitational effects related to pulmonary artery catheter position and by diastolic pressure gradients in the pulmonary vasculature.
Although the systolic SV of the left ventricle is not measured directly, it can be determined from measurements of CO and HR. If LV systolic ejection fraction (EF) has been determined, the end-diastolic volume (EDV) and end-systolic volume (ESV) of the left ventricle can be determined: EDV = SV/EF and ESV = EDV – SV.
Preload is an estimation of average end-diastolic myocardial fiber length and correlates best with ventricular EDV. As the left ventricle distends, EDV, rather than end-diastolic pressure, is a highly predictive determinant of systolic function. Mechanical interaction between the two ventricles and between each ventricle and the surrounding mediastinal and thoracic structures can also influence ventricular distensibility. LV end-diastolic pressure (rather than EDV) can be used to monitor preload only when those factors that alter ventricular distensibility are constant. When ventricular distensibility is changing (due to, for example, the loss of myocardial compliance that occurs with transient ischemia), the measurements or estimates of ventricular diastolic pressure do not accurately represent preload.
The term afterload usually is used to describe the forces that retard the ventricular ejection of blood. The afterload of the right and left ventricles is determined primarily by the resistive and capacitive characteristics of the pulmonary and systemic circulations. As blood is ejected from the ventricle, the actual afterload forces that oppose the shortening of myocardial fibers are distributed as stresses throughout the ventricular walls.
The Frank-Starling principle is useful in predicting the hemodynamic outcome of therapeutic interventions. This is illustrated by the curvilinear relationship between ventricular stroke work (y-axis) and ventricular end-diastolic pressure (x-axis). When preload is represented by EDV, rather than by end-diastolic pressure, this relationship becomes linear and is minimally affected by afterload and HR [12]. The slope of this relationship is a sensitive indicator of intrinsic myocardial performance and responds appropriately to inotropic interventions. The augmentation of stroke work by increases in preload is referred to as preload recruitable stroke work (Fig. 148.2).
Increases in CO, afterload, preload, inotropic state, and HR are all achieved with increased myocardial oxygen demand. Intraoperatively, myocardial oxygen demand is eliminated by hypothermia and chemical cardioplegia. Postoperatively, if the myocardial work is too intense or the blood supply is too small, myocardial ischemia, failure, and infarction may result. An important feature of myocardial oxygen consumption is that oxygen extraction is nearly maximal at rest, so that increases in myocardial oxygen consumption can only be achieved by increases in coronary blood flow. Increased afterload is, to a degree, self-compensatory in that increased diastolic coronary perfusion pressure tends to increase coronary blood flow. Increases in inotropic activity may also be associated with increases in myocardial blood flow and a correspondent increase in diastolic aortic pressure.
Maximizing cardiac function to meet metabolic demands, therefore, involves the manipulation of volumes and pressures that affect preload and afterload and the support and enhancement of myocardial contractility. Andre and DelRossi [13] note that the postoperative myocardium is cold and stiff and generally behaves as a pressure-overloaded system. Volume may be needed despite high measured filling pressures. As the patient recovers and the myocardium warms, compliance improves and the relationship of filling pressures to ventricular volumes changes.
Initial Status
On arrival to the ICU, a systematic assessment should include preoperative history with attention to medications and cardiac function, intraoperative history, vital signs, and physical examination. Immediate goals and short-term goals need to be established. Many patients arrive hypothermic with temperatures ranging from 34°C to 36°C as a result of deliberate systemic cooling during cardiopulmonary bypass. Persistent peripheral vasoconstriction can be the result of elevated angiotensin levels [14]. Shivering during rewarming increases metabolic and circulatory demands, increases carbon dioxide production, and complicates ventilator management. Shivering can be eliminated with paralyzing agents and sedation [15,16]. The patient is generally maximally warm by 4 to 6 hours after operation.
As the patient rewarms and awakens, the goal is to support the recovering myocardium until it is independently able
to meet metabolic demands. Cardiac output is measured and normalized to cardiac index (CI) by dividing it by the patient’s body surface area. Efforts to correct an initial cardiac index of less than 2 L per m2 per minute should be made because low cardiac index is associated with an increased risk of death [17,18]. The clinical correlates of reduced cardiac index are pale and cool skin, cyanotic mottling of the skin (occurring first over the knees), decreased urine output, and deterioration of mental status or slowness in awakening from anesthesia. A low CI and decreased peripheral perfusion also cause metabolic acidosis (from lactic acid accumulation in poorly perfused tissues; see Chapter 71), which, to a mild degree, occurs even after routine operations.
to meet metabolic demands. Cardiac output is measured and normalized to cardiac index (CI) by dividing it by the patient’s body surface area. Efforts to correct an initial cardiac index of less than 2 L per m2 per minute should be made because low cardiac index is associated with an increased risk of death [17,18]. The clinical correlates of reduced cardiac index are pale and cool skin, cyanotic mottling of the skin (occurring first over the knees), decreased urine output, and deterioration of mental status or slowness in awakening from anesthesia. A low CI and decreased peripheral perfusion also cause metabolic acidosis (from lactic acid accumulation in poorly perfused tissues; see Chapter 71), which, to a mild degree, occurs even after routine operations.
Normally, the mixed venous hemoglobin saturation (SvO2) should be 60% or higher. If it is less than 50%, a high likelihood of death exists [17,19]. The SvO2 should be interpreted in light of the cardiac index and hemoglobin. In the worst situation, and the one that often leads to death, the SvO2 may be adequate only because so much of the peripheral tissues are underperfused [17]. In this case, however, the cardiac index also is reduced. The value of SvO2 is limited because it does not describe the balance of oxygen in those tissues with fixed oxygen extraction. The kidney, skin, and resting muscle can maintain viability during reduced blood flow by augmenting oxygen extraction. The heart and brain, on the other hand, extract oxygen nearly maximally at rest, and their vulnerability to ischemia is not reflected by widened oxygen extraction.
Postoperative hypertension is common and may be a consequence of several factors, such as inadequate sedation, hypoxemia, hypercarbia, activation of cardiogenic reflexes, vasoactive drug administration, and withdrawal of beta-blocking agents; however, intense vasoconstriction accounts for most of the hypertension. Failure to control the blood pressure increases the risk of aortic tear, elevates myocardial oxygen demand, leading to the possibility of decreased subendocardial perfusion and ischemia.
As a consequence of fluid administration, the patient seen in the ICU just after an operation on cardiopulmonary bypass usually weighs 2 to 5 kg more than preoperatively. Urine output is typically high in patients with good LV function. If urine output is low, intravascular volume or CO may be low. Inappropriate antidiuretic hormone excretion commonly exists as a consequence of operative trauma. The patient is frequently treated with IV nitroglycerin and other afterload-reducing and venodilating agents. These agents shift blood volume to the periphery and consequently decrease preload. These factors tend to reduce urine output.
Treatment of Low Cardiac Output
Low CO in the postoperative period is associated with a higher incidence of respiratory, renal, hepatic, and neurologic failure. Treatment of low CO first requires an analysis of possible causes (Table 148.1). Operative complications, such as coronary graft closure, inadequate revascularization, poor myocardial protection, valve malfunction, or paravalvular leak, can cause pump dysfunction. Graft closure or acute coronary occlusion can have immediate hemodynamic effects (a fall in CO and a rise in left-sided filling pressures). Early graft failures are usually due to technical factors, but perioperative myocardial infarction due to coronary spasm can also occur in operated or in nonoperated vessels [20]. When the diagnosis of spasm is entertained and ST-segment changes as well as wall motion abnormalities occur, aggressive management with nitroglycerin and diltiazem should be instituted [21]. If these drugs are unsuccessful in reversing the hemodynamic deterioration, cardiac catheterization or reexploration, or both, inspection of the grafts should be considered [22]. Myocardial depression can be seen in the first 24 hours as a result of the operation. Common causes of perioperative pump dysfunction include arrhythmias, tamponade, hypovolemia, myocardial infarction, systemic acidosis, electrolyte imbalance, and hypoxia.
Table 148.1 Causes of Low Cardiac Output | |
---|---|
|
Early graft patency is an important determinant of postoperative ventricular function and performance on stress tests. On the other hand, the occurrence of perioperative myocardial infarction without hemodynamic compromise has not been shown to be significantly related to graft patency, late survival, or cardiac performance status [23]. The treatment of perioperative infarction consists of therapy to maintain CO, including afterload reduction, especially with nitroglycerin and beta-blockade, if tolerated.
If an obvious cause of low CO is not identified, a systematic approach toward optimizing pump function should be undertaken (Table 148.2). An easy way to organize this approach is by examining preload, afterload, rate, contractility, and rhythm. Because CO is the product of SV and HR (CO = SV ∞ HR), either can be increased.
On arrival to the ICU, many patients exhibit intravascular volume depletion, despite an increase in total body water. The rewarming that is actively done during the early postoperative period causes progressive peripheral vasodilatation and relative
hypovolemia. The goal MAP is 70 to 80 mm Hg [13]. Normovolemia is essential and can be accomplished with autotransfusion, normal saline, lactated Ringer’s solution, albumin (25% solution), or hydroxyethyl starch (hetastarch). In the Saline versus Albumin Fluid Evaluation (SAFE) study involving almost 7,000 patients, albumin had no proven advantage over crystalloids in critically ill patients, although a larger volume of crystalloid is necessary compared to colloid [24]. Hetastarch can provide volume expansion for more than 24 hours. At doses more than 20 mL per kg, it can cause a decrease of factor VIII levels and platelets. Urticarial and anaphylactoid reactions as well as pancreatitis can occur with the use of this product [25].
hypovolemia. The goal MAP is 70 to 80 mm Hg [13]. Normovolemia is essential and can be accomplished with autotransfusion, normal saline, lactated Ringer’s solution, albumin (25% solution), or hydroxyethyl starch (hetastarch). In the Saline versus Albumin Fluid Evaluation (SAFE) study involving almost 7,000 patients, albumin had no proven advantage over crystalloids in critically ill patients, although a larger volume of crystalloid is necessary compared to colloid [24]. Hetastarch can provide volume expansion for more than 24 hours. At doses more than 20 mL per kg, it can cause a decrease of factor VIII levels and platelets. Urticarial and anaphylactoid reactions as well as pancreatitis can occur with the use of this product [25].
In addition to ensuring adequate volume resuscitation, clinician should optimize cardiac rate and rhythm. Ventricular filling occurs during diastole and is augmented by a properly timed atrial contraction. If the heart rate is excessive to the extent that there is inadequate time for ventricular filling, cardiac output will be affected. This is particularly true of the hypertrophied or pressure overloaded ventricle and a heart rate of 90 to 100 beats per minute is optimal [13]. After cardiac surgery, atrial fibrillation, sinus bradycardia, and varying degrees of heart blockage can occur. These arrhythmias are usually transient and may be related to perioperative beta-blockade, hyperkalemic damage during the administration of cardioplegia, or unprotected ischemia of the conduction system [26]. Permanent injury to the conduction system is usually the result of surgically induced trauma.
Temporary atrial and ventricular wires are placed at the time of surgery and can be used to maintain CO. Simple atrial pacing (at a rate of 80 to 100 beats per minute) for the treatment of sinus bradycardia may effectively augment CO. Atrial pacing can aggravate a first-degree heart blockage and introduce an atrioventricular dyssynchrony. In this situation, atrioventricular sequential pacing should be attempted. The optimal atrioventricular interval is usually in the range of 100 to 175 milliseconds, depending on the HR. The advantage of atrial pacing over atrioventricular sequential pacing is the maintenance of the normal anatomic pattern of ventricular activation. Loss of the normal sequence of activation depresses ventricular function by approximately 10% to 15%.
Although a low MAP is most common, occasionally one must lower excessive afterload to improve cardiac output. Decreasing systemic vascular resistance (SVR) decreases the heart’s oxygen demand. In patients with relatively normal LV function, nitroprusside reliably decreases SVR and increases CO, whereas nitroglycerin may lower CO, perhaps as a result of too great a decrease in cardiac preload (left atrial pressure).
The PV relationship of the left ventricle can be used to predict improvements in stroke volume secondary to reductions in afterload. The therapeutic results depend on the inotropic state of the ventricle. Ventricles with the poorest contractility benefit the most from afterload reduction. If the ventricle is operating on an end-systolic PV relationship with a shallow slope (depressed contractility), reducing afterload (and end-systolic pressure) results in a relatively large increase in SV (Fig. 148.3).
Afterload reduction is also beneficial when residual mitral regurgitation and aortic insufficiency are present.
Afterload reduction is also beneficial when residual mitral regurgitation and aortic insufficiency are present.
![]() Figure 148.3. The improvement in stroke-volume that can be achieved with a reduction in afterload (and consequently, a reduction in end-systolic pressure) depends on the inotropic state of the myocardium. There is more to be gained by afterload reduction in a ventricle with depressed inotropic state (a smaller slope of the end-systolic pressure-volume relationship). |
Table 148.3 Vasodilators Used in Postoperative Cardiac Surgery Patients | |||||||||||||||||||||||||||||||||||||||||||||||||||||||||||||||||||||||||||||||
---|---|---|---|---|---|---|---|---|---|---|---|---|---|---|---|---|---|---|---|---|---|---|---|---|---|---|---|---|---|---|---|---|---|---|---|---|---|---|---|---|---|---|---|---|---|---|---|---|---|---|---|---|---|---|---|---|---|---|---|---|---|---|---|---|---|---|---|---|---|---|---|---|---|---|---|---|---|---|---|
|
The postoperative patient with a low CO and an adequate blood pressure may benefit from afterload reduction using incremental doses of nitroprusside. Cardiac index and SV rise as filling pressures and blood pressure fall. Nitroprusside must be used with caution because of its potential for causing cyanide or thiocyanate poisoning, or both. Nitroprusside infusions generally should not exceed 8 μg per kg per minute (Table 148.3). In the presence of ischemia or an acute myocardial infarction, nitroglycerin increases regional myocardial flow and decreases ischemic ST segments toward normal, whereas nitroprusside may have an opposite and deleterious effect [27,28]. Improvement in cardiac function with inotropic agents is generally at the expense of increased myocardial oxygen demand. Inotropic agents, therefore, should be used only when manipulation of HR, rhythm, preload, and afterload are ineffective. When LV depression and low output persist, inotropic therapy must be used. A number of drugs and drug regimens can be used, including dopamine, dobutamine, epinephrine, norepinephrine, and amrinone or milrinone (Table 148.4).
Dopamine usually causes a small increase in HR, although in some patients severe tachycardia can be seen. Dopamine increases cardiac index by stimulating β-adrenergic receptors. At doses less than 3 μg per kg per minute, dopamine causes renal, splanchnic, coronary, and cerebral arterial vasodilatation by the activation of dopaminergic receptors. When dopamine is infused at a rate below 7.5 μg per kg per minute, it causes little change in SVR; above this rate, systemic vasoconstriction, due to stimulation of α-adrenergic receptors, increases. The usual dose range for dopamine is 1 to 20 μg per kg per minute.
Dobutamine is a synthetic catecholamine with minimal α-adrenergic activity but pronounced β1– and β2-adrenergic activity. It increases CO by increasing ventricular contractility and rate as well as causing peripheral vascular dilatation. For patients with a low CO and marked peripheral vasoconstriction, dobutamine is preferable to dopamine when the latter is used alone. Nevertheless, because dobutamine is a vasodilator, use of this drug in the presence of hypotension may lead to further hypotension. The usual doses for dobutamine are 5 to 20 μg per kg per minute.
Epinephrine is an α-, β1-, and β2-receptor agonist. It increases myocardial contractility and rate. It also increases ventricular irritability. Peripherally, its β-mediated effects (vasodilation) predominate at low doses, whereas α-mediated effects (vasoconstriction) predominate at high doses. The usual epinephrine dose is 1 to 10 μg per minute (0.015 to 0.15 μg per kg per minute).
Norepinephrine has α- and β-adrenergic activity. It increases systemic and pulmonary blood pressure myocardial contractility and CO. Internal mammary grafts remain innervated and are responsive to vasoactive drugs; saphenous vein grafts are not. Norepinephrine has been shown to decrease flow in internal mammary grafts less than phenylephrine in the early postoperative period [29]. The usual dosage is 4 to 10 μg per minute (0.06 to 0.150 μg per kg per minute).
Milrinone is an “inodilator,” producing a positive inotropic independent of adrenergic stimulation and causing a reduction in systemic and pulmonary vascular resistance. Milrinone is a phosphodiesterase inhibitor that increases intracellular concentrations of cyclic adenosine monophosphate. Milrinone is a bipyridine derivative that is 20 times more potent than amrinone [30,31]. They are usually used as a second-line medication when a low CO persists despite catecholamines. Concomitant use of catecholamines usually offsets any associated vasodilation. The usual dosage of milrinone is a loading dose of 50 μg per kg over 10 minutes, followed by an infusion of 0.375 to 0.75 μg per kg per minute. Administration of milrinone over a period of 10 minutes prevents the vasodilation that is observed with rapid loading [32,33,34].
Arginine vasopressin may be helpful if hypotension persists despite adequate cardiac output, despite use of vasoactive
substances like epinephrine; “vasoplegia” or autonomic failure may be present. Vasopressin levels are low in normotensive patients after cardiac surgery and disproportionately low in patients with “vasodilatory shock.” Acting on vascular V1 and renal V2 receptors, in doses ranging from 0.1 to 0.4 U per min, vasopressin can be effective in improving vascular tone. Care in its use must be taken in patients with marginal cardiac output as vasopressin may further compromise splanchnic blood flow [13].
substances like epinephrine; “vasoplegia” or autonomic failure may be present. Vasopressin levels are low in normotensive patients after cardiac surgery and disproportionately low in patients with “vasodilatory shock.” Acting on vascular V1 and renal V2 receptors, in doses ranging from 0.1 to 0.4 U per min, vasopressin can be effective in improving vascular tone. Care in its use must be taken in patients with marginal cardiac output as vasopressin may further compromise splanchnic blood flow [13].
Table 148.4 Inotropic Agents Used in Postoperative Cardiac Surgery Patients | ||||||||||||||||||||||||||||||||||||||||||||||||||||||||||||||||||||||||||||||||||||||||||||||||||||||||||||||||||
---|---|---|---|---|---|---|---|---|---|---|---|---|---|---|---|---|---|---|---|---|---|---|---|---|---|---|---|---|---|---|---|---|---|---|---|---|---|---|---|---|---|---|---|---|---|---|---|---|---|---|---|---|---|---|---|---|---|---|---|---|---|---|---|---|---|---|---|---|---|---|---|---|---|---|---|---|---|---|---|---|---|---|---|---|---|---|---|---|---|---|---|---|---|---|---|---|---|---|---|---|---|---|---|---|---|---|---|---|---|---|---|---|---|---|
|

Full access? Get Clinical Tree
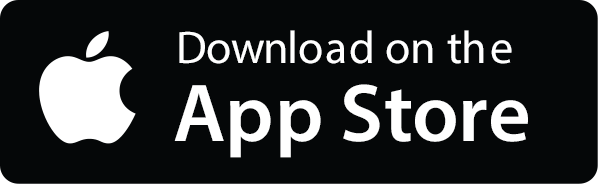
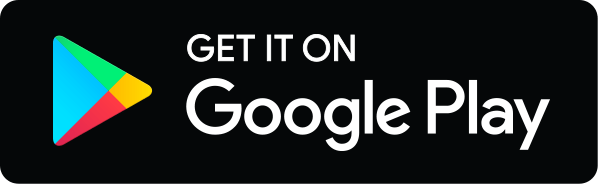