Management of the Post–Cardiac Arrest Patient
BACKGROUND
In the United States, out-of-hospital cardiac arrest (OHCA) affects roughly 300,000 people each year.1 Despite herculean efforts in improving outcome, mortality from cardiac arrest remains high, with only 8% to 10% of these patients surviving to hospital discharge.1,2 Between 35% and 61% of OHCA victims will have return of spontaneous circulation (ROSC) either in the prehospital setting or in the emergency department (ED),3–5; of those, between 36% and 79% will rearrest in the acute care setting.6
Anoxic brain injury is the leading cause of morbidity in patients with cardiac arrest who survive to hospital admission. Over the last decade, the development and use of therapeutic hypothermia has made neuroprotection the cornerstone of therapy for hemodynamically stable survivors of cardiac arrest. Sustained reperfusion is also fundamental to neurologic salvage, and identifying and addressing the initial cause of cardiac failure is essential if further episodes of hemodynamic instability are to be prevented.
ACUTE CARE AND DIAGNOSTIC EVALUATION
A sense of relief often comes over ED staff in the aftermath of successful ROSC, once the flurry of CPR activity has subsided and the patient has regained a pulse. However, a continued sense of urgency is required for the phases of evaluation and treatment that follow. As with all critically injured patients, the first priorities of management for a post–cardiac arrest patient are to secure and confirm the placement of a definitive airway and obtain intravenous access for the delivery of fluid and vasoactive medications. Although multiple large-bore peripheral IVs are acceptable initially, a central venous catheter (CVC) is preferred, in order to accommodate potential vasopressor administration. The goals of post–cardiac arrest management in the ED are subsequently organized along two parallel paths: identifying and treating the primary cause of the arrest; and initiating therapeutic cooling in appropriate candidates.
Understanding the context of the arrest can be critical in determining its cause. Witnesses—whether emergency medical services (EMS), family members, or bystanders—should be questioned about symptoms that preceded the collapse, such as chest pain, shortness of breath, dizziness, or severe headache. It is equally important to identify preexisting conditions that predispose to cardiac events, including coronary artery disease (CAD), congestive heart failure (CHF), deep venous thrombosis (DVT), or renal disease.
A twelve-lead electrocardiogram (ECG) should be obtained as early as possible to look for evidence of cardiac ischemia. Multiple studies have shown that acute coronary thrombosis is the single most common cause of sudden atraumatic cardiac arrest. Other potential causes include primary arrhythmia, electrolyte disturbances, dilated cardiomyopathy, pulmonary embolus, hypoglycemia, accidental hypothermia, tension pneumothorax, cardiac tamponade, toxic overdose, and subarachnoid hemorrhage. Of these, several can be eliminated based on history and physical exam. A basic metabolic panel or an arterial blood gas can be used to identify dangerous electrolyte abnormalities, while pneumothorax and tamponade, although uncommon in the absence of trauma, can be identified with chest radiography and bedside cardiac ultrasound.
Given the diagnostic uncertainty that can be present after ROSC, the International Liaison Committee On Resuscitation (ILCOR) has emphasized the importance of a comprehensive search for reversible causes of OHCA.7 A recent study retrospectively reviewed 896 cases of OHCA in PROCAT, a cardiac arrest registry in Paris, France, in which all patients received a diagnostic procedure to identify the cause of arrest.1 The authors describe an algorithm in which, following ROSC, patients suspected of underlying cardiac etiology were taken for immediate cardiac catheterization regardless of initial rhythm and ECG changes. For patients suspected of having a noncardiac etiology, brain computed tomography (CT) and chest CT angiography (CTA) could be performed to assess for intracranial hemorrhage or pulmonary embolism (PE), respectively. When cardiac catheterization did not reveal a culprit lesion, the patient could then be taken for CT; similarly, if an initial CT did not reveal a cause of arrest, the patient could be taken for cardiac catheterization. Out of 896 patients without an obvious cause of OHCA, 729 (81%) received an immediate diagnostic coronary angiogram. In approximately 39% of patients taken to the catheterization lab initially, no culprit lesion could be identified. Following a negative coronary angiogram, 188 patients underwent CT, which revealed a diagnosis in 33 cases (17.6%). Conversely, 167 patients underwent initial CT at the time of hospital admission that demonstrated a cause of arrest in 39 cases (23.4%). Sixteen of the patients whose initial CT was negative went on to receive a cardiac catheterization, which revealed a cause of arrest in 5 cases. Overall, using this approach, physicians were able to identify a cause of cardiac arrest in 524 of 896 cases based on the results of 452 cardiac catheterizations and 72 CT studies. The most common extracardiac cause of OHCA identified by CT was stroke, followed by PE.
The routine use of head CT is not endorsed in established guidelines on the management of cardiac arrest after ROSC; however, CT effectively rules out brain hemorrhage, which, if detected, would affect the decision to treat the patient with anticoagulants fundamental to therapy for both coronary and pulmonary thrombosis.
CARDIAC CATHETERIZATION
The single most common cause of OHCA is myocardial infarction due to acute coronary artery occlusion. Because of neurologic injury, survivors of OHCA are often unresponsive after CPR and/or are heavily sedated and paralyzed. Few physicians would disagree that in the presence of true ST-segment elevation >0.5 mm in two or more contiguous leads, patients should be taken emergently for cardiac catheterization and percutaneous coronary intervention (PCI) regardless of the patient’s mental status. Considerably more controversy exists regarding the proper course of action for the comatose patient for whom a twelve-lead ECG is nondiagnostic following ROSC. In one study, among survivors of OHCA without a history of chest pain or diagnostic ECG changes, 26% had evidence of recent coronary occlusion on angiography.8 Similar rates of significant coronary occlusion have been demonstrated in other cohorts of patients lacking diagnostic ECG findings. In a study of OHCA patients with angiographic evidence of acute myocardial infarction (MI), 12% were without evidence of ST-segment elevation on their postresuscitation ECG.9
The high rate of electrographically silent myocardial ischemia in cardiac arrest patients has led some authors to suggest that all survivors of OHCA should be taken for coronary angiography, regardless of ECG findings. Others reason that such aggressive screening has not been shown to improve outcomes in this group of patients; not only is their prognosis frequently dictated by the degree of neurologic damage rather than that of myocardial necrosis, but also, unselected angiography of all OHCA patients is economically wasteful. Proponents counter by pointing out that neurologic prognosis based on clinical exam and imaging is unreliable immediately after ROSC, and that the potential for lethal, untreated coronary artery occlusion necessitates immediate investigation and treatment in patients with amenable lesions, in order to limit myocardial damage.
A 2012 study of 240 patients after resuscitation from cardiac arrest found improved survival to discharge among the 25% of patients who were taken for coronary angiography within 6 hours of reaching the hospital compared to patients who were taken later or not at all (72% vs. 49% discharged alive).10 In another report of 72 angiograms performed on survivors of OHCA (at an institution that performed cardiac catheterization on all OHCA patients with successful ROSC), the diagnosis of acute MI was made in 37.5% of all patients. ST-segment elevation on admission ECG yielded a positive predictive value (PPV) for a final diagnosis of acute MI of 82.6% and a negative predictive value (NPV) of 83.7%. In this study, the roughly 16% of missed MI in patients with a normal ECG strongly suggests that ECG alone is not a sufficiently sensitive test to rely on in a potentially lethal disease process.11
Another recent study attempted to analyze the impact of emergency cardiac catheterization on in-hospital outcome among unconscious survivors of OHCA. The study reviewed the outcomes of 93 patients with OHCA and ROSC after <20 minutes; of these, 66 received a cardiac catheterization, and of those, 52% were found to have an acute or recent culprit lesion. Forty-two percent of those with an acute or recent culprit lesion identified did not demonstrate ST-segment elevation on their post-ROSC ECG. Sixty percent of patients who received emergency coronary angiography were discharged from the hospital alive compared to 54% overall.12
A large prospective cohort study completed in 2012 analyzed the 1- and 5-year outcomes of 5,958 patients with OHCA who were admitted alive to the hospital. One thousand and one (16.8%) were discharged alive; of these, 384 (38.4%) received cardiac catheterization, 80% of whom had PCI performed. Therapeutic hypothermia (TH) was performed in 241 of 941 (25.6%) patients comatose at hospital admission. Patient outcomes were analyzed in groups according to whether they received PCI, therapeutic hypothermia, both therapies, or neither. At 1 and 5 years after discharge, survival was highest in those patients who had received both PCI and TH.13
CARDIAC ARREST WITH MASSIVE PULMONARY EMBOLISM
The percentage of OHCA caused by PE is estimated to be between 0.2% and 13.3%.14–16 Differentiating between PE and other causes of cardiac arrest has profound implications for patient treatment, since PE may be amenable to treatment with thrombolytics and catheter or surgical embolectomy. In a 2008 study, patients with OHCA and a suspected cardiac etiology (without a confirmed ST segment elevation myocardial infarction [STEMI]) were randomized to empiric intravenous tissue plasminogen activator (tPA) or placebo; there was no demonstrable benefit from tPA, effectively limiting this therapy to cardiac arrest cases with a strong suspicion of PE etiology.17 Making this distinction, however, is challenging and complicated by considerable overlap of symptoms among arrest-inducing etiologies. In the ED, CTA of the chest is the optimal diagnostic test for PE, and should be considered in all patients without an obvious etiology for their arrest.7 Echocardiography is another useful diagnostic modality in massive PE (discussed in detail in Chapter 11), and will typically demonstrate right ventricular dysfunction, including RV/LV end-diastolic diameter ratio ≥1 in four-chamber view, paradoxical septal motion, and pulmonary hypertension with RV/atrial gradient ≥30 mm Hg.
Intravenous tPA is the standard of care for a massive PE that results in hemodynamic instability or cardiac arrest, and is given in a dose of 100 mg, with 10 mg given as a bolus and the remaining 90 mg infused over a period of 2 hours.18 Catheter-directed embolectomy and open thoracotomy are other, albeit less well-researched, interventions for massive PE. Catheter-directed embolectomy is performed using a combination of aspiration, clot fragmentation, rheolysis, and direct administered thrombolysis at the site of the clot. In a cohort of patients with persistent hemodynamic instability after receiving thrombolytics for PE, one study observed that patients who received a rescue embolectomy with a catheter-based approach had lower mortality (7% vs. 38%) and lower risk of recurrent PE (0% versus 35%).19 Catheter-directed embolectomy is still rarely performed in most medical centers and should be considered only when cardiothoracic surgical services exist to manage the potential vascular complications. ED staff who suspect PE as the cause of cardiac arrest or hemodynamic instability should involve interventional radiology and cardiothoracic surgery early in the decision-making process.
THERAPEUTIC HYPOTHERMIA
As already noted, mortality and long-term disability resulting from cardiac arrest are frequently due to severe neurologic injury during and after the ischemic period of the arrest. To date, most experiments with neuroprotective strategies aimed at improving clinical outcome after cardiac arrest have failed, with the notable exception of therapeutic hypothermia (TH). The benefit of postarrest hypothermia is likely mediated though multiple pathways. Neuronal injury after cardiac arrest is the result of disrupted calcium homeostasis, inflammatory cell migration, excitatory neurotransmission, and the activation of proteolytic and apoptotic pathways.20 Several investigators have shown that for every 1°C drop in body temperature, the cerebral metabolic rate decreases by approximately 7%, effectively blunting the effects of neuronal injury.21 Hypothermia is also thought to protect the integrity of the blood–brain barrier, which may attenuate ischemia-related cerebral edema.
Two randomized trials of therapeutic hypothermia after OHCA were published in 2002; both demonstrated benefits in neurologic outcome and survival. Both sets of investigators randomly assigned survivors of OHCA to a target temperature of between 32°C and 34°C after ROSC, for a duration of 12 to 24 hours. The first study showed a significant reduction in neurological morbidity in patients receiving TH; this finding was confirmed by the second study, performed by the Hypothermia After Cardiac Arrest (HACA) investigators.22,23 In addition, HACA also found a significant reduction in 30-day mortality associated with TH. Although it has been more than a decade since these and many confirmatory follow-up studies have been published, recent surveys show that TH is still underutilized. Based on the initial two studies, six patients need to be treated (NNT) to prevent one poor neurologic outcome, and seven treated to prevent one death. To put this statistic into perspective, the NNT to prevent one poor neurologic outcome using tissue plasminogen activator in stroke is eight. For thrombolytics in acute myocardial infarction, the NNT to save one life is between 20 and 33.
Current recommendations that guide which patient groups should receive therapeutic hypothermia are based on the populations included in the original two studies, and include those comatose patients with successful ROSC following ventricular fibrillation (VF) and pulseless ventricular tachycardia (VT).22,23 Patients with an initial rhythm of pulseless electrical activity have a poorer prognosis than do those with rhythms responsive to defibrillation; however, it is likely that once ROSC is established, these patients will benefit from the neuroprotective effect of TH as well. Treatment should thus be initiated as soon as possible in all nontraumatic cardiac arrest patients with significantly altered mental status or coma. TH should probably be withheld in those patients with significant ongoing hemorrhage or sepsis. Although the original trials demonstrating benefit for TH excluded patients with cardiogenic shock, more recent studies have confirmed that these patients also derive a mortality benefit from TH, and that cooling in fact decreases vasopressor requirements.24
In the setting of STEMI, immediate PCI or thrombolysis can be lifesaving; several reports have shown that both procedures are compatible with cooling. Early TH should be instituted prior to hospital transfer and continued throughout the catheterization procedure. Several published reports have demonstrated the compatibility of TH with anticoagulation, thrombolytics, and PCI without a significant increase in complications.25,26 When compared with historical controls that were not cooled, rates of bleeding complications in patients receiving TH were identical, even in those patients receiving clopidogrel, GPIIA/IIIB inhibitors, and heparin. Studies have also reported that TH can be initiated prior to cardiac catheterization with no impact on door-to-balloon time.25 To effectively implement early TH in cardiac arrest patients who need immediate PCI, the ED and the catheterization team must collaboratively create standing protocols on TH indications and method of use, and discern how best to achieve rapid goal temperature without sacrificing door-to-balloon time.
Therapeutic hypothermia should be initiated as soon as possible after ROSC is achieved. Animal models of VF arrest have shown that even a 15-minute delay in the initiation of cooling results in markedly worse neurologic outcome and more severe histologic damage of brain tissue.27,28 If ROSC is achieved in the field, induction of hypothermia should begin with EMS providers and continue through transfer to the ED. The application of therapeutic hypothermia consists of three phases: (1) The induction phase, during which core body temperature is actively decreased; (2) the maintenance phase; and (3) the rewarming phase, when body temperature is allowed to return to its physiologic baseline.
At the end of 2013, a randomized controlled trial of patients with OHCA compared therapeutic hypothermia with a standard target temperature of 33° Celsius to that with a target temperature of 36°.29 This multi-center trial enrolled 939 patients and demonstrated no significant difference in mortality or neurological outcome between management strategies, raising questions about the optimal target temperature for TH after OSCA. Patients in both arms of the study received bystander CPR roughly 73% of the time and had a median ROSC time of 25 minutes. The trail leaves unanswered whether patients surviving arrest under different circumstances would still benefit from more aggressive temperature lowering. Patients were eligible for inclusion in the trial if they were screened within 4 hours of their arrest. It is at least possible that more aggressive cooling has a larger beneficial impact when initiated earlier in the course, after reperfusion has occurred. The study has also been criticized for failing to test for subtle differences in cognitive ability between the groups and for failing to control for differing sedation strategies that may have influenced outcomes. Further research is warranted to determine which subgroups of cardiac arrest patient can safely be treated with therapeutic hypothermia using this less aggressive strategy.
Cooling Methods
Therapeutic hypothermia may be initiated via several methods, including surface cooling, endovascular cooling, and infusions of iced saline. Surface cooling employs ice packs, evaporative sprays, cooling blankets, or fitted pads with circulating cold water to lower temperature. Both of the original TH studies demonstrated profound clinical benefit using surface cooling alone, often only with the use of ice packs. Cold intravenous saline, which is easy to apply and provides for rapid induction of TH at low cost, is being utilized with increasing frequency. In healthy anesthetized volunteers, one study found that rapid infusion of 40 mL/kg of 4°C normal saline via a CVC resulted in a drop in core temperature of 2.5°C in 30 minutes.30 This drop was greater than expected due to peripheral vasoconstriction, which caused relative isolation of the core and peripheral body compartments. Conversely, peripheral vasoconstriction decreases the efficacy of cooling when surface techniques are used. In another study, OHCA victims were randomized to cold saline infusion of up to 2 L upon ROSC while still in the care of EMS providers. Field cooling was associated with a mean drop in temperature of 1.2°C from initiation of infusion to arrival in the ED.31 No significant differences were found between groups in the PaO2, vasopressor requirements, or pulmonary edema on initial chest radiograph. In a study examining the effects of large-volume cold saline infusion on respiratory function after cardiac arrest, 52 patients who received an average of 3 L of cold saline experienced only a small decrease in their PaO2 to FiO2 ratio—from 290 at admission to the intensive care unit (ICU) to 247.5—while maintaining oxygen saturation in the normal range.32
A 2005 prospective study reported on 134 cardiac arrest patients, including those with cardiogenic shock, who received 4°C saline in addition to surface cooling for the induction of hypothermia. On average, patients without shock received approximately 2 L of cold fluid in 60 minutes and experienced a drop in temperature from 36.9°C to 32.9°C.33 In the patients with cardiogenic shock, a more conservative protocol with slower fluid administration was used, but still resulted in a change in core temperature from 36.8°C to 33.1°C over 120 minutes. Only 8 of 134 patients in the study required modest increases in positive end-expiratory pressure (PEEP) to maintain PaO2 during fluid administration, of which 5 demonstrated evidence of cardiogenic shock prior to fluid infusion. Overall, the data support the routine use of large volume (30 mL/kg) of ice-cold (4°C) saline for the induction of HACA.
Physiologic Effects of Hypothermia
Induction of mild hypothermia alters normal cardiac, pulmonary, endocrine, and renal functions and carries a risk of adverse effects. At temperatures between 32°C and 34°C, myocardial contractility increases and heart rate declines as a result of decreased spontaneous depolarization of pacemaker cells.34 Peripheral vasoconstriction causes an increase in systemic vascular resistance that may manifest as an increased blood pressure during induction. Metabolic rate decreases by 7% to 8% for every 1°C decrease in core body temperature, so decreases in cardiac output as a result of induced bradycardia do not usually result in unmet oxygen demand or tissue ischemia. A brisk diuresis occurs in response to an expansion of central blood volume and may require aggressive fluid repletion to maintain cardiac filling pressures.35 Below 30°C, the risk of clinically relevant arrhythmias increases steeply, with atrial fibrillation occurring most commonly, and ventricular tachycardia and fibrillation becoming more likely as temperature drops further.35 Careful observation of body temperature during induction is important to avoid overcooling and the associated risk of malignant arrhythmia.
As core body temperature drops, observed changes in serum electrolytes are due to intracellular shifts in potassium, magnesium, calcium, and phosphate.36,37 Hourly monitoring of electrolytes, with repletion as needed, is recommended during the induction phase of cooling to maintain normal levels. Later on, as rewarming is started, potassium that shifted into cells during the induction phase may suddenly exit the cells, creating a risk of rebound hyperkalemia. Ensuring slow rates of rewarming—no faster than 0.25°C per hour—helps minimize this complication, as well the risk of rebound cerebral edema.21
Mild hypothermia causes decreased insulin secretion and insulin resistance, which may result in hyperglycemia and increased insulin requirements during the induction and maintenance phases of cooling.21 In brain-injured patients, the optimal serum glucose level has not been determined, but maintaining levels between 100 and 180 mg/dL is reasonable.
Platelet function is impaired and clotting times are increased with TH. These effects however, have not been associated with increased rates of clinically significant bleeding after OHCA, even when TH is used in conjunction with antiplatelet drugs, anticoagulants, and thrombolytics.38
Hypothermia also impairs leukocyte migration and the production of inflammatory mediators, thereby reducing the body’s ability to fight off infection. The HACA trial demonstrated a trend toward increased risk of pneumonia and sepsis with cooling, and other studies have confirmed the association between TH and increased rates of infection.23,39 Close observation for signs of evolving infection should be maintained throughout the cooling process. During active cooling, fever may not reliably be observed, but the work required to achieve cooling goals—a measure indicated on multiple newer devices—can be used as a surrogate for the development of fever.
Shivering
The normal shivering response is the greatest obstacle to rapid cooling; if not aggressively controlled, shivering can contribute to increased metabolic demand and undermine the beneficial effects of TH. Management follows a stepwise protocol that begins with measures to decrease the shivering threshold, and proceeds to include nonvolatile anesthetics such as propofol and, rarely, paralytics for severe refractory shivering. Initial agents used for shivering control include buspirone, meperidine, magnesium sulfate, and alpha-2 agonists. Buspirone, a serotonin (5HT)-1A partial agonist, is thought to lower the shivering threshold by activating hypothalamic heat loss mechanisms. Meperidine, which acts synergistically with buspirone, is a unique opiate agonist of both kappa and mu receptors shown to be effective in decreasing shivering. Magnesium sulfate, when targeted to a serum level of between 3 and 4 mg/dL, reduces shivering by promoting peripheral vasodilation and muscle relaxation. Alpha-2 agonists, such as clonidine and dexmedetomidine, are thought to inhibit neuronal firing related to thermosensitivity. Finally, skin surface rewarming, although counterintuitive, has also been shown to decrease the core temperature shivering threshold by 1°C for every 4°C rise in skin temperature.40
When the above therapies fail, fentanyl and propofol infusions can be employed to suppress refractory shivering. During the rapid induction phase of cooling, a bolus dose of a long acting paralytic such as rocuronium or vecuronium is encouraged; however, because of the concern for critical illness myopathy, ongoing infusions of paralytic medications to suppress shivering are discouraged for all but the most refractory cases.
Continuous EEG
Seizure activity is common in victims of anoxic brain injury but may be easily missed in the setting of therapeutic hypothermia where deep sedation or paralysis is required. Nonconvulsive status epilepticus (NCSE), defined as unremitting epileptiform activity in the absence of clinical seizures, has been reported in 8% to 9% of patients in undifferentiated coma.41–43 The presence of NCSE has been associated with increased risk of mortality and severe disability at hospital discharge. In one study, 10% of comatose patients in the ICU had continuous seizure activity on cEEG, 68% of which was not detected clinically. Seizure activity was associated with a 19-fold increase in the odds of death or severe disability.44 It is not clear whether seizures are the direct cause of poor outcome or merely a marker of severe brain injury; nevertheless, in those patients for whom aggressive care is appropriate, it is reasonable to monitor and treat seizures in the immediate postarrest period under the assumption that unceasing epileptiform discharges inhibit neurologic recovery.
PERCUTANEOUS HEMODYNAMIC SUPPORT
Following resuscitation from cardiac arrest, many patients will experience significant hemodynamic instability requiring varying degrees of vasopressor and inotropic support. In patients with cardiogenic shock from acute myocardial infarction or other cardiac insult, high-dose vasopressors and inotropes may be required. By augmenting cardiac contractility and afterload, however, these medications can increase myocardial oxygen consumption and exacerbate cardiac ischemia. In patients requiring cardiac catheterization, extracorporeal membrane oxygenation (ECMO) and intra-aortic balloon pump (IABP) are two temporizing percutaneous interventions that may be used to maintain hemodynamic stability.
As percutaneous hemodynamic support devices have become smaller, they have begun to gain wider use in the management of acute cardiogenic shock. Unlike veno–veno ECMO that can only be used to support oxygenation, venoarterial (VA) ECMO can be used to provide hemodynamic support. These devices typically consist of two 18-gauge French catheters that are inserted into the femoral artery and vein, essentially creating a right atrial-to-aortic shunt. Blood is removed through the femoral vein catheter where it enters the ECMO circuit for oxygenation and is returned via pump in retrograde fashion to the aorta. A side-port is inserted into the distal femoral artery to prevent limb ischemia.
A 2011 study described the creation of an Extracorporeal life support (ECLS) team capable of quickly establishing a VA ECMO circuit to provide rapid hemodynamic support, and demonstrated that ECLS could be used to facilitate CT imaging and PCI in survivors of cardiac arrest.45 Over the course of 6 years, the ECLS team was activated for 144 patients, 58 of whom had ECLS established. Overall survival in the ECLS group was 38%. A 2012 study described initiating percutaneous hemodynamic support with a VA ECMO circuit in a combination of 28 patients with cardiac arrest from acute myocardial infarction (AMI) or PE. In all AMI patients, hemodynamic support was initiated while the patient was still in arrest; in the patients with PE, 10 of 12 survived, 70% with good neurologic outcome.46 A similar study of 22 patients who received VA ECMO initiated during cardiac arrest reported that 41% were discharged home from the hospital with no disability.47 In the last two studies, an IABP was frequently used in conjunction with VA ECMO to reduce LV afterload.
Percutaneous hemodynamic support of the type described above requires a cardiothoracic surgeon or interventional cardiologist to establish the perfusion circuit, and is only being used in a minority of academic medical centers. To date, no randomized trials of this technology in selected cardiac arrest patients have been performed.
CONCLUSION
OHCA remains a catastrophic event that continues to claim tens of thousands of lives each year in the U.S. alone. Rapidly identifying the etiology of the arrest is essential to initiating the correct treatment. Myocardial infarction is the single most common cause of OHCA and may present in approximately 10% of patients without characteristic ECG changes. Diagnostic algorithms that combine CT imaging and cardiac catheterization will increase accurate diagnosis of the etiology of cardiac arrest and may improve outcomes by prompting early identification and treatment. Therapeutic hypothermia is still the only therapy proven to have a mortality benefit after cardiac arrest and should be initiated as soon as possible in all eligible patients. Therapeutic hypothermia has been shown to be compatible with anticoagulants, thrombolytics, and cardiac catheterization, and should not be delayed for any of these treatments.
LITERATURE TABLE
CI, confidence interval; HR, hazard ratio; OR, odds ratio; RR, relative risk.

Full access? Get Clinical Tree
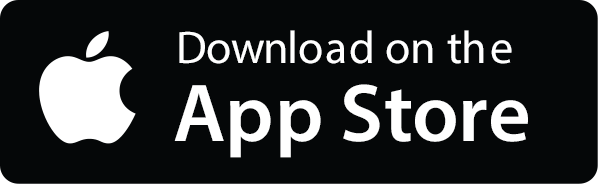
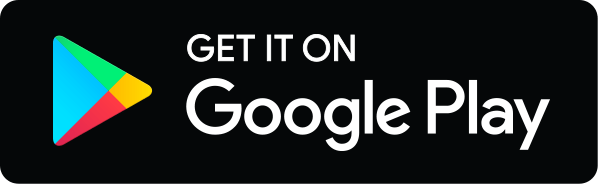