Fig. 8.1
Calibrated automated thrombography. ETP endogenous thrombin potential; TF tissue factor
Conventional tests offer a limited information on thrombin generation, even if prothrombin time and international normalized ratio are related to extrinsic pathway-dependent thrombin generation and activated partial thromboplastin time to intrinsic pathway-dependent thrombin generation.
Viscoelastic tests (thromboelastography, TEG®, and rotational thromboelastometry ROTEM®) may offer a contribution to indirect assessment of thrombin generation. In TEG and ROTEM, whole blood may be activated by kaolin (classical TEG) and ellagic acid (INTEM) or by TF (rapid TEG and EXTEM). The time required to change the physical properties of blood from the liquid phase to the gel phase (time to gel point [31]) is expressed as reaction time (R-time, TEG) or clotting time (CT, ROTEM) and may be considered as a surrogate for thrombin generation through the intrinsic or extrinsic pathway. However, it should be considered that the main determinant of a poor thrombin generation after cardiac surgery is a residual heparin activity [32]. Whereas some tests are insensitive to heparin (EXTEM), other tests like classical TEG should be run with and without heparinase to rule out the heparin role and address heparin-independent thrombin generation.
The main problem of viscoelastic tests in the setting of cardiac surgery is their very low positive predictive power for severe postoperative bleeding. In particular, there is a poor or even null relationship between R-time or CT and postoperative bleeding (Fig. 8.2). However, once there is the evidence for microvascular bleeding, viscoelastic tests may provide some information suggestive for poor thrombin generation. After cardiac surgery, a certain degree of reduced thrombin generation is usual and acceptable. Empirically, an R-time or CT time up to 20 % longer than the upper normal range may be considered a routine value. At present, we are lacking clear cutoff values suggestive for a bleeding mechanism related to poor thrombin generation. In the setting of pediatric cardiac surgery, a CT value >111 s was suggested [33], whereas other algorithms in adult cardiac surgery empirically suggest shorter values, around 80–90 s [34–36]. Given the low positive predictive power of these tests, and the multifactorial nature of postoperative bleeding in cardiac surgery, these cutoff values make sense only in the bleeding patient and once heparin effects or other reasons for bleeding are ruled out.
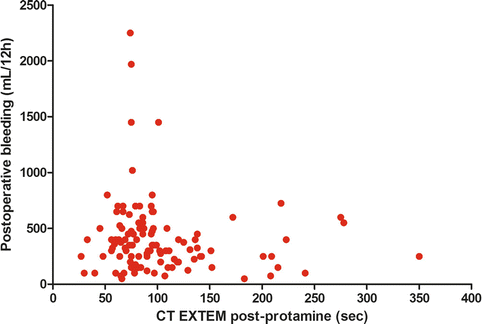
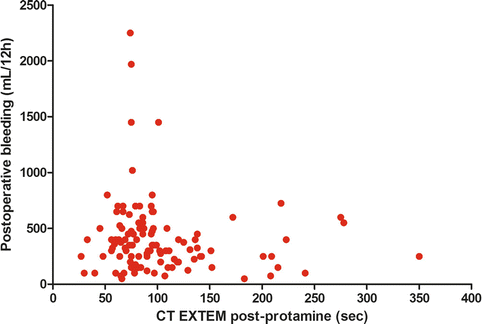
Fig. 8.2
Relationship between clotting time at ROTEM (after protamine administration) and postoperative bleeding. No significant association was found (personal data). CT clotting time
8.2.2.2 Therapeutic Interventions
Bleeding related to a poor thrombin generation may be corrected using fresh frozen plasma (FFP), 3–4 factors prothrombin complex concentrates (PCCs), activated factors like factor eight inhibitors bypassing activity (FEIBA), or recombinant activated factor VII (rFVIIa).
FFP is the most “natural” source of coagulation factors; however, large doses are required to restore thrombin generation. The dose ranges from 10 to 15 mL/kg in mild patterns of decreased thrombin generation to larger doses (30 mL/kg) in the most severe cases [37]. FFP is still largely used in the setting of cardiac surgery, basically due to its low cost and to its nature of “balanced” solution, containing both pro-coagulant and anticoagulant factors. However, FFP is associated with a number of possible adverse reactions (see Chap. 6) and to fluid overload.
PCCs are certainly more effective in terms of thrombin generation recovery. Their use for the treatment of dilution/consumption coagulopathy has been successfully tested in cardiac surgery patients [38, 39]. However, there are reports of increased thromboembolic complications linked to the use of PCCs [40].
Due to its ability to bypass the other coagulation factors, rFVIIa is able to induce a thrombin burst. The suggested dose ranges from 60 to 90 μg/kg i.v. rFVIIa is certainly effective in reducing bleeding in cardiac surgery as well as in other scenarios [41, 42]. However, there is a well-established risk of thromboembolic complications [41].
As a general rule, every correction leading to increased thrombin generation (with the exception of FFP) has been linked to an increased risk of thromboembolic events. Additionally, it should be considered that thrombin alone is unable to induce the formation of a stable clot unless adequate amounts of fibrinogen and well-working platelets (together with FXIII) are available. Therefore, a prerequisite for interventions aimed to increase thrombin generation is the presence of adequate levels of the abovementioned hemostatic factors.
8.2.3 Low Fibrinogen Levels
Fibrinogen is converted into fibrin by thrombin and binds platelets through the platelet receptor GPIIbIIIa, leading to a stable clot after the intervention of factor XIII.
After cardiac surgery, low levels of fibrinogen are a common finding [9]. Low levels of fibrinogen after surgery have been associated with postoperative bleeding in a number of studies [9, 43–45]. Additionally, other studies have found an association between preoperative fibrinogen levels and postoperative bleeding [46–49].
The clinical conditions leading to low fibrinogen levels after cardiac surgery are similar to those leading to a consumption of coagulation factors.
8.2.3.1 Functional Fibrinogen Tests
Low fibrin(ogen) levels lead to a poor clot firmness. In general, there is presently a general opinion that clot firmness may be most relevant than thrombin generation in the pathophysiology of severe postoperative bleeding in cardiac surgery.
Viscoelastic tests provide a measure of clot firmness based on the viscoelastic properties of the clot, namely, expressed in terms of “amplitude” (mm) of the formed clot. For TEG, this is represented by the maximum amplitude (MA) and for ROTEM as maximum clot firmness (MCF). However, these measures provide a combined assessment of the interaction between fibrin(ogen) and platelets and are therefore not specific for fibrin(ogen) contribution to clot firmness. To achieve this specific measure, the platelet contribution must be eliminated, and modified TEG/ROTEM assays are available. Basically, platelet contribution is blunted by a GPIIbIIIa inhibitor in functional fibrinogen (FF) TEG and by cytochalasin D in FIBTEM (ROTEM).
The resulting values of MA and MCF at FF-TEG or FIBTEM are representative of fibrin(ogen) alone contribution to clot firmness.
There is an acceptable correlation between viscoelastic tests measure of fibrin(ogen) contribution to clot firmness and fibrinogen levels as measured with the conventional Clauss method [50] (Fig. 8.3). However, the two measures are not interchangeable, since one represents fibrinogen concentration, the other a functional fibrinogen activity.
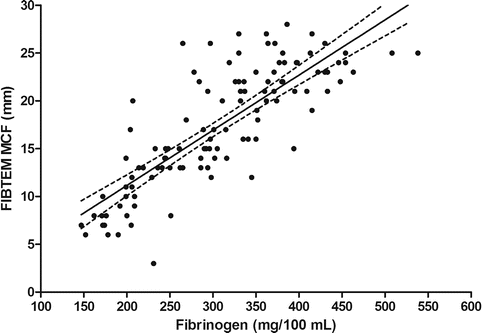
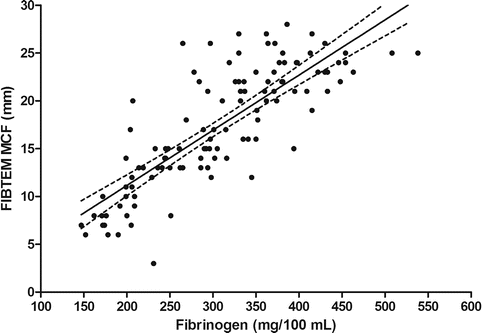
Fig. 8.3
Linear regression relationship between fibrinogen concentration (Clauss method) and maximum clot firmness at the FIBTEM ROTEM test (personal data). Dashed lines are 95 % confidence interval. MCF maximum clot firmness
At present, it is still unclear which levels of postoperative fibrinogen should lead to fibrinogen supplementation. Some authors suggest trigger values of 1.0–2.0 g/L [51–53], while others suggest higher values in the setting of cardiac surgery [54].
A specific issue is represented by the possibility of prophylactic correction of low preoperative fibrinogen levels. The recently released guidelines for the management of severe perioperative bleeding (European Society of Anaesthesiology) [54] consider the possibility of correcting values <3.8 g/L, based on a single, small randomized controlled trial [47]. However, an analysis of the existing literature [55] has demonstrated that preoperative fibrinogen levels have a very poor (about 10 %) positive predictive power for prediction of severe postoperative bleeding. A trigger value of 3.8 g/L would lead to an unacceptable 90 % rate of superfluous treatments. Trigger values settled around 2.0 g/L seems more adequate, but however a strategy based on postoperative values is certainly more reasonable.
8.2.3.2 Therapeutic Interventions
Fibrinogen can be supplemented using three different strategies. FFP contains fibrinogen but actually at a very variable concentration. A reasonable value can be settled at about 2 g/L [56], and therefore, the amount of FFP to increase fibrinogen levels by 1 g/L is about 30 mL/kg. Therefore, the same considerations applied to FFP as a source of factors increasing thrombin generation may be applied to FFP as a source of fibrinogen, the main problem remaining the large dose required to achieve a clinically relevant benefit.
A second source of fibrinogen is represented by cryoprecipitates. The fibrinogen content in cryoprecipitates is variable, but a value of 12 g/L seems reasonable [57]. In some countries, cryoprecipitates are the standard of care for fibrinogen supplementation in case of acquired bleeding; however, they are not available in many European countries [58].
Fibrinogen concentrate is probably the most effective treatment for fibrinogen deficiency. The high concentration (20 g/L) allows treatment without volume overload, and its purified nature limits the risks associated with FFP and cryoprecipitates. The suggested dose is 25–50 mg/kg [54]. Fibrinogen concentrate is not licensed for acquired bleeding in the UK and USA [58].
Many studies suggest to settle the fibrinogen dose on the value of MCF at FIBTEM [17–19]. A target value of 22 mm at FIBTEM is suggested, and an equation to achieve the target has been proposed [59, 60]:
However, following this equation leads to administration of large doses of fibrinogen concentrate. At present, we are lacking an evidence-based information with respect to the exact fibrinogen trigger values, targets, and dosage.
![$$ \begin{array}{l}\mathrm{F}\mathrm{ibrinogen}\;\mathrm{concentrate}\;\mathrm{dose}\;\left(\mathrm{g}\right)\hfill \\ {}\kern2em =\left(\begin{array}{l}\mathrm{target}\;\mathrm{F}\mathrm{IBTEM}\;\mathrm{M}\mathrm{C}\mathrm{F}\;\left[22\;\mathrm{mm}\right]\hfill \\ {}-\mathrm{actual}\;\mathrm{F}\mathrm{IBTEM}\;\mathrm{M}\mathrm{C}\mathrm{F}\;\left[\mathrm{mm}\right]\hfill \end{array}\right)\hfill \\ {}\kern2.84em \times \left(\mathrm{bodyweight}\;\left[\mathrm{kg}\right]/140\right).\hfill \end{array} $$](/wp-content/uploads/2017/07/A326222_1_En_8_Chapter_Equa.gif)
A recent RCT on postoperative fibrinogen concentrate administration demonstrated the efficacy of this strategy in reducing postoperative bleeding and transfusions [61]. This study suggested that a target MCF at FIBTEM in the range of 15–17 mm is enough to avoid severe bleeding.
Finally, FXIII has been proposed to increase clot firmness in the setting of the bleeding patient. However, a recent randomized controlled trial of FXIII supplementation in cardiac surgery failed to demonstrate any effect on bleeding and transfusions [62].
8.2.4 Thrombocytopenia
After cardiac surgery with CPB, a certain degree of thrombocytopenia is inevitable. Different factors concur in its determinism: platelet activation and adhesion to the CPB circuit, hemodilution, extensive use of cell-saver, platelet activation due to the elevated shear stress in critical parts of the CPB circuit, and thrombin-induced platelet activation.
Platelet counts about 30–40 % lower than the preoperative value are common findings and are not necessarily associated with bleeding. In adults, platelet count rarely reaches critical levels unless in case of preoperative thrombocytopenia. This last condition can be found in patients operated under septic conditions or in patients with congenital cyanotic heart disease.
8.2.4.1 POC Tests and Platelet Count
The diagnosis of thrombocytopenia is based on conventional cell count. However, thrombocytopenia may be suspected in case of low values of clot firmness (MA-MCF) with normal values of functional fibrinogen. When applying this concept, however, it should be considered that the values of MA/MCF are not linearly correlated with the real viscoelastic properties of the clot.
8.2.4.2 Therapeutic Options
The only therapeutic option is transfusion of platelet concentrates. Compensating a low platelet count with large doses of fibrinogen is a theoretical option which has never been confirmed in large studies.
8.2.5 Platelet Dysfunction
There are basically two mechanisms leading to acquired platelet dysfunction in cardiac surgery patients. The first is related to the preoperative use of antiplatelet agents (aspirin and P2Y12 receptor inhibitors); the second depends on the effects of surgery and CPB (platelet activation by the extensive thrombin generation and adhesion to foreign surfaces). As a result of these combined effects, after cardiac surgery, platelet dysfunction is an invariable finding. Both adhesion and aggregation are blunted, in a time-dependent fashion, by CPB [63].
Platelet function tests (PFTs) in this setting have an important role. However, they are still poorly diffused in the clinical practice, and their role in preventing and treating postoperative bleeding is still a matter of debate.
8.2.5.1 Platelet Function Tests before Surgery
Before surgery, coronary patients are usually treated with aspirin and/or P2Y12-inhibitors (ticlopidine, clopidogrel, prasugrel, and ticagrelor). Aspirin determines a mild increase in postoperative bleeding [64]; conversely, P2Y12-inhibitors are associated with patterns of severe bleeding and risk of reoperation [65, 66].
The existing guidelines suggest 5 days of drug discontinuation before surgery [67, 68], but it is admitted that even three days may be enough [67]. Platelet recovery after antiplatelet drug discontinuation is highly individual and somehow unpredictable [69]; for this reason, the use of PFTs to assess the correct timing of surgery after P2Y12-inhibitors discontinuation is considered a reasonable option [54, 70]. Conventional tests confirm that preoperative assessment of platelet function may predict postoperative bleeding: a value <40 % of ADP-dependent platelet activation at light transmission aggregometry was found predictive of severe bleeding in clopidogrel-treated patients [71].
Multiple-electrode aggregometry (MEA) has been proposed to assess ADP-dependent platelet reactivity in P2Y12-inhibitors treated patients. A study based on the Multiplate technology (Roche Diagnostics GmbH, Mannheim, Germany) proposed a minimal value of 31 [U] at the ADP test as a suitable cutoff level for admitting patients to surgery, with a negative predictive power of 92 % [72]. Subsequently, a combination of ADP test and TRAP test was found to offer better accuracy: a minimal value of TRAP test of 75 [U] in patients with an ADP test <22 [U] was associated with a 100 % negative predictive power for severe bleeding [73] MEA was found useful even to detect patients that may be operated after a time from drug discontinuation shorter than five days [74].
Other POC-PFTs have shown an association between ADP-dependent preoperative platelet function and postoperative bleeding. Modified TEG (TEG platelet mapping) may predict postoperative bleeding in clopidogrel pretreated patients [75]. A preoperative value of MAadp <42.5 mm had a 78 % sensitivity and 84 % specificity for predicting excessive bleeding [75].
The VerifyNow (Accumetrics Inc, San Diego, CA, USA) is another POC-PFT which can aid in determining the timing of cardiac surgery: preoperative values of ADP-dependent platelet inhibition >21 % in clopidogrel-treated patients have been found associated with a higher surgical revision rate and blood use in off-pump coronary surgery [76]. A previous study demonstrated an association between preoperative VerifyNow P2Y12 platelet inhibition and blood loss and blood use in coronary patients under double antiplatelet therapy until three days before surgery [77].
Recently, ROTEM has provided a ROTEM platelet device based on multielectrode aggregometry, but no data are available at present.
8.2.5.2 Platelet Function Tests after Surgery
After surgery, PFTs show different degrees of platelet dysfunction, depending on the preoperative use of antiplatelet agents, the type of surgery, and the duration of CPB. Low levels of platelet function after surgery have been associated with postoperative bleeding [78, 79]. Algorithms including PFTs for the diagnosis of postoperative bleeding exist, including trigger values for desmopressin infusion or platelet concentrate transfusions. A recent study proposed MEA test values for triggering interventions aimed to increase platelet function: a TRAP test <50 [U] and/or an ASPI test <30 [U] and/or an ADP test <30 [U] [34]. Previously, another study suggested the following values: TRAP test <50 [U] and/or an ASPI test <20 [U] and/or an ADP test <30 [U] to trigger platelet concentrates transfusions in actively bleeding patients after cardiac surgery [36]. These values, however, are empirical and not yet validated; they have been proposed within algorithms including other POC tests to rule out other possible causes of bleeding. The combination of drug-related factors with operation-related factors makes difficult a clear determination of a specific value of postoperative platelet activity responsible for severe bleeding.
8.2.5.3 Therapeutic Interventions
The most reasonable approach to the treatment of drug-related platelet dysfunction bleeding is the transfusion of platelet concentrates. In patients treated with ticagrelor, the transfused platelets may be attacked by the still active forms of the drug, given the pharmacokinetic of ticagrelor.
Desmopressin (0.3 μg/kg) may be used to treat surgical bleeding due to acquired platelet dysfunction, especially in the setting of acquired von Willebrand disease (aortic stenosis patients) [80] and after CPB. Despite a low level of evidence, this approach is included in the European Guidelines [54].
8.2.6 Hyperfibrinolysis
Hyperfibrinolysis has been identified as a cofactor of hemostatic impairment during cardiac surgery contributing to cardiopulmonary bypass coagulopathy and microvascular bleeding [81].
Fibrinolysis is responsible for fibrin degradation and consequently for clot breakdown. Fibrinolysis starts when plasmin cleaves fibrin and fibrinogen. The process is triggered by the plasminogen activators as tissue plasminogen activator (t-PA) released by the Weibel-Palade bodies of endothelial cells and urokinase plasminogen activator synthesis by monocytes, macrophages, and fibroblasts. The process is regulated by the plasminogen activator inhibitors (PAI) type 1 and 2, the thrombin activatable fibrinolytic inhibitor (TAFI), and the α-2-antiplasmin. The most important systemic plasminogen inhibitor PAI 1 is mainly released by platelets at the site of a forming thrombus. Hyperfibrinolysis occurs when the balance between fibrinolysis activators and inhibitors is altered [82, 83].
Activation of fibrinolysis depends on several simultaneous mechanisms [84], and both intrinsic and extrinsic coagulation pathways can initiate fibrinolysis [14].
Cardiac surgery using CPB is associated with increased fibrinolytic activity compared to off-pump cardiac surgery. During cardiopulmonary bypass, excessive fibrinolysis can due to either primary or secondary fibrinolysis (i.e., as related to reduced fibrinolysis inhibitors such as PAI-1, alpha 2-antiplasmin) [83]. Before weaning from CPB, a discrepancy between plasma levels of plasmin and alpha 2-antiplasmin is observed due to alpha 2-antiplasmin absorption to extracorporeal surfaces [85]. The high thrombin generation throughout CPB associated with the contributions of high levels of epinephrine, bradykinin and vasopressin trigger a high fibrinolytic activity [2]. Almost immediately after CPB initiation, t-PA levels rise 6-fold and plasmin generation suffers a 100-fold increase [86]. Besides, alternative pathways of fibrinolysis as neutrophil elastase-mediated fibrin digestion may also contribute to hyperfibrinolysis during CPB demonstrating that the systemic inflammatory response syndrome induced by CPB is closely related to hemostatic phenomena [87]. Plasmin interacts also with the inflammatory system triggering the complement cascade and kallikrein [14].
The fibrinolytic response to CPB is heterogeneous, and large interindividual variations in fibrinolytic response have been observed during CPB. The most frequent (40 %) pattern or typical response consists in a rapid rise in active and total t-PA during CPB followed postoperatively by elevated PAI-1 and reduced t-PA. Approximately one-third of patients show no change in fibrinolytic parameters. When present, the t-PA response is rapid, occurring within the first 30 min of CPB and is more common in patients undergoing valve surgery than in coronary artery bypass grafting [88, 89]. Some studies have described the influence of genetic factors as 675 (4G75G) polymorphism, on the plasma levels of PAI 1, t-PA, and t-PA/PAI-1 complex [90]. The main factors contributing to fibrinolysis are the t-PA released from CPB-mediated contact activation of factor XII, thrombin, or hypothermia or released from the traumatized shocked endothelial cells or returned from the blood suctioned from the pericardial cavity [91–93].
Moreover, hyperfibrinolysis could affect other aspects of hemostasis. For instance, plasmin may reduce platelet adhesion and aggregation by degradation of von Willebrand receptor glycoprotein Ib and platelet fibrinogen receptor glycoprotein IIb/IIIa, respectively [94].
Hyperfibrinolysis can be classified as primary and secondary hyperfibrinolysis. Primary is independent to high amounts of thrombin generation, whereas secondary hyperfibrinolysis is the consequence of activation of coagulation and thrombin formation. Secondary hyperfibrinolysis is not always harmful as it prevents end organ damage caused by microvascular fibrin deposition during disseminated intravascular coagulation [81].
8.2.6.1 Therapeutic Interventions
When bleeding is associated to primary hyperfibrinolysis, the use of an antifibrinolytic agent is appropriate. Several studies and meta-analyses have shown the synthetic lysine analogues and aprotinin to be effective in reducing bleeding and the need for blood transfusion during high-risk cardiac surgery, as compared with placebo [95–99]. However, the blood conservation using antifibrinolytics in a randomized trial (BART) demonstrated a strong and consistent negative mortality trend associated with aprotinin compared to lysine analogues, leading to aprotinin withdrawal worldwide by manufacturer in 2007 [100]. The synthetic lysine analogues tranexamic acid (TXA) and epsilon-aminocaproid acid (EACA) inhibit in a reversible way the fibrinolytic enzyme plasmin. Both are indicated to reduce the number of patients who require blood transfusion and to reduce total blood loss after cardiac operations. However, these agents are slightly less potent drugs, and the safety profile of these drugs is less well studied compared with aprotinin [66].
A wide range of dosages of antifibrinolytic drugs has been proposed [101]. Suggested TXA doses during cardiac surgery varies from a loading dose of 12.5 mg/kg followed by an infusion of 6.5 mg/kg/h [102] up to higher doses such as 20 g administered during a period of 12 h [103]. Often, the chosen regime is not denoted by pharmacokinetic studies. It has been observed that plasma levels are maintained over the therapeutic level for most of the duration of cardiopulmonary bypass with a regime of 10 mg/kg loading dose followed by an infusion of 1 mg/kg/h for up to 2 h after arrival in intensive care unit. The dosing of TXA is important because its side effects are dose related [104]. The administration of high doses of TXA has been associated to a higher rate of seizures in the postoperative period [104, 105]. The safer scheme and optimal dosing regimen for lysine analogues in cardiovascular surgery has still to be established [101]. Currently, a new antifibrinolytic drug CU-2010 is in clinical development [106].
Monitoring the fibrinolytic activity can be made by the determination of D-dimers, FDP, and XDP plasma levels or using viscoelastic tests. The D-dimer, FDP, an XDP levels are not quickly and immediately available. Besides, D-dimers are not useful to determine the etiology of coagulopathy in situations of perioperative bleeding, as its concentration is usually increased postoperatively in all patients [81]. Viscoelastic hemostatic assays such as TEG and ROTEM are the current most useful clinical hemostatic test used in perioperative setting to determine hyperfibrinolytic status in bleeding patients. However, it has been argued that the viscoelastic tests only detect the most severe forms of systemic hyperfibrinolysis, but lower degrees of fibrinolysis may critically influence the outcome too [107].
The TEG parameters of lysis are the Ly30 and Ly60 (percentage of lysis 30 and 60 min after maximum amplitude respectively) and the EPL (estimated percent lysis parameter). Both parameters measure the decrease in amplitude of the trace as a function of time and reflect the loss of clot integrity due to clot lysis [108]. ROTEM parameters of lysis are maximum lysis (ML) [%] and clot lysis index 30 or 60 min after maximum clot firmness (CLI30; CLI60) [%]. Monitoring can be performed in EXTEM or HEPTEM tests that allow for timely detection of hyperfibrinolysis even under the conditions of anticoagulation with heparin during cardiopulmonary bypass. In addition, the APTEM test consists in a specific ROTEM assays that test the in vitro effect of antifibrinolytics (i.e., aprotinin) in a whole blood sample of a bleeding patient [109]. According to some published protocols in cardiac surgery, hyperfibrinolytic state needing antithrombotic therapy is diagnosed in the presence of EPL >7.5 % in TEG analysis [110] or CLI60 <85 % according to ROTEM analysis during or after CPB [36].
8.2.7 Surgical Sources
The nature itself of cardiac surgery involves the risk of surgical sources of bleeding (suture lines, small arteries, collateral vessels, and others). In this setting, the value of POC tests relies on their high negative predictive value. In the setting of the bleeding patient, each test should be applied to rule out residual heparin effects, poor thrombin generation, poor clot firmness, and hyperfibrinolysis. Once none of these factors appear to be involved, surgical revision of the patient is warranted.
8.3 Bleeding in Pediatric Cardiac Surgery
Children that undergo cardiac surgery are particularly prone to surgical and CPB effects on the coagulation system. Early postoperative hemorrhage is independently associated with increased mortality in infants after cardiac surgery [111]. Besides the alterations of coagulation due to exposure to CPB, the risk for postoperative coagulation disorders in this population is increased by small age and body weight, as well as pre-existing congenital and acquired coagulation disorders, specific for cardiac congenital heart disease.
8.3.1 Preoperative Conditions
8.3.1.1 Developmental Hemostasis
The concept of developmental hemostasis, introduced by Andrew and colleagues [112–115], states that the hemostatic system of newborns and infants is effective and they do not suffer from spontaneous hemorrhagic complications, but their concentration of the majority of coagulation proteins, as measured by functional assays, varies significantly with age. At birth. plasma concentrations of the vitamin K-dependent and contact factors are decreased, whereas fibrinogen, FV, FVIII, and FXIII are similar or increased compared with adults. Plasma concentrations of plasminogen are decreased, whereas tissue plasminogen activator and plasminogen activator inhibitor are increased. The hemostatic system of the young is a dynamic process. Within the first 6 months of life, at times even later, the concentration of the coagulation factors reaches the adult levels. Reference ranges for ROTEM and TEG are determined for all pediatric age groups [116–118].
8.3.1.2 Congenital Heart Disease (CHD) and Coagulation
In patients with CHD, the heart defect itself compromises coagulation. Neonates and infants with single-ventricle or two-ventricle physiology have lower levels of pro-coagulant and anticoagulant factors compared to healthy children of the same age group [119, 120]. POC-based studies (TEG and ROTEM) indicate that children of less than 1 year affected by congenital heart disease have a lesser balance between coagulation and fibrinolysis compared to healthy children of the same age [121, 122] and that cyanosis and/or polycythemia has the major negative impact on hemostasis [123].
8.3.1.3 Special Considerations in Children with Cyanotic Heart Disease
Cyanotic heart disease is associated with a significant tendency to bleeding. Platelet count reduction in cyanotic children is an ongoing process. In 25 % of the patients of a study that included Eisenmenger syndrome patients, the authors found thrombocytopenia. They hypothesized that platelets are reduced due to right-to-left intracardiac shunts that bypass the lungs where megakaryocytes are fragmented into platelets [126]. Born aggregometry studies have shown a higher platelet reactivity compared to controls in children with cyanotic heart disease [127]. At hematocrit levels over 60 %, due to blood hyperviscosity, there is an overproduction of platelet microparticles and suppression of platelet aggregation [128]. Recently, some authors reported impaired fibrinogen functionality (detected by TEG and TEG FF) in patients with cyanotic heart disease [129, 130].
8.3.2 Intraoperative Bleeding Mechanisms in Pediatric Cardiac Surgery
Exposure to CPB puts in jeopardy the fragile hemostatic balance of the young. The mechanisms of intraoperative coagulation alterations are the same as for adult patients, but the impact they have on the children hemostasis is more pronounced. On CPB levels of coagulation, factors and platelet counts are lower than the adult ones because the preoperative coagulation alterations sum up with coagulation factors consumption and massive dilution by CPB priming. In an average adult, the CPB priming volume is 1/3–1/4 of total blood volume (i.e., 1000–1500 mL of priming to 4200–4500 mL of total blood volume). For a newborn, it can be 1–1.5 times the total blood volume (i.e., 250–300 mL of priming volume to 200–250 mL of total blood volume). In a study in neonates, at the initiation of CPB, the authors found 50 % decrease in circulating coagulation factors and antithrombin levels, as well as 70 % of platelet count reduction [131].
8.3.2.1 Low Thrombin Generation
In pediatric cardiac surgery, thrombin generation has been studied with the markers of thrombin generation such as TAT and F 1.2 and recently with calibrated automated thrombography [132]. Preoperative thrombin generation is a predictive risk factor for postoperative bleeding [133], but intraoperative data is lacking. The information that viscoelastic tests give on thrombin generation is used in the perioperative settings for its evaluation. To date, there are no cutoff values that can guide therapy. In a recent algorithm, CT ≥111 s was suggested to trigger hemostatic therapy [33].
FFP and cryoprecipitates are the sources of coagulation factors more used in pediatric patients. Where available, especially in small patients, cryoprecipitate (0.5–1 Unit/kg) is a much more efficient source of coagulation factors than FFP.

Full access? Get Clinical Tree
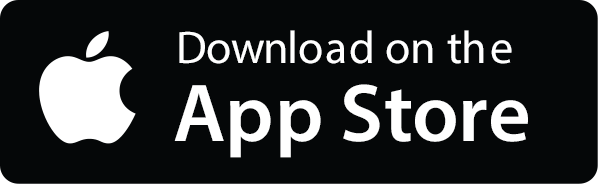
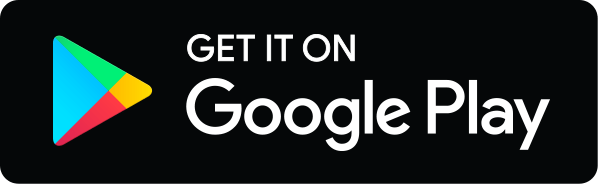