PEEP, positive end-expiratory pressure; ARDS, acute respiratory distress syndrome; LIS, lung injury score; ALI, acute lung injury; PAOP, pulmonary artery occlusion pressure; LV, left ventricle. aSum scores from each domain and divide by number used (ARDS = LIS >2.5;bdiagnose ARDS when all four criteria are present;cdiagnose ARDS when criteria 1-4 and/or 5b are present. Modified from Ferguson ND, Frutos-Vivar F, Esteban A, et al. Acute respiratory distress syndrome: underrecognition by clinicians and diagnostic acccuracy of three clinical definitions. Crit Care Med. 2005;33:2228-2234, with permission.
Ventilator Induced Lung Injury (VILI)
Studies conducted on experimental animals who were treated with large tidal volumes or excessive inspiratory pressures capable of producing alveolar overdistention have demonstrated physiologic, radiographic, and histologic evidence of lung injury that is similar to the injury pattern noted in ALI and ARDS.9,10,10a,10b,10c A classic demonstration of this finding was evidenced by the production of hemorrhagic, edematous changes in an isolated rat lung when it was subjected to an inspiratory pressure of 45 cm H2O for only 5 minutes.10a These changes were even more evident after 20 minutes, and the lung demonstrated the characteristic finding of diffuse alveolar damage on histology.10a This same form of injury can develop from negative pressure ventilation in open chest animals and supports the concept that the basic mechanism of this injury is from overdistention of alveoli.9 Alveolar overdistention is one form of ventilator induced lung injury and has also been termed volutrauma.
However, it is important to realize that over-expansion of the alveoli is just one of the mechanisms capable of producing VILI.10 Other causes of ventilator-induced lung injury include barotrauma, biotrauma, atelectatrauma, translocation of microorganisms or air (air embolism), and toxic oxygen radicals.10 For example, volutrauma occurs when the alveolus is over-distended. Atelectotrauma is lung injury associated with the recurrent opening and closing of alveoli and small respiratory bronchioles that my result in shear stress.10 The overdistention of alveoli can damage the capillary endothelial cells or alveolar epithelial cells (type 1 or type 2), which may then produce various active pro-inflammatory molecules and other active substances that can enter the systemic circulation and produce inflammatory or possibly anti-inflammatory reactions throughout the body. Translocation of O2 or bacteria through the gap junctions that occur between the alveolar cells and/or capillary endothelial cells can result in air embolization or translocation of bacteria or toxins. The elaboration of toxic O2 radicals can produce injury not only locally but also systemically. Barotrauma is lung damage induced by high pressure, as in pneumothorax, pneumomediastinum, pneumopericardium, pneumoperitoneum, or subcutaneous emphysema.
When discussing VILI, it is important to view it in both physical and mechanical terms. Physical aspects include strain, stress, stiffness, yield stress, and plastic deformation. Mechanically, it is all about mechanotransduction, in which there is a deforming stress of the alveolus, as it is overdistended, that pulls on the epithelial cells.11 Remember that it is the type 2 alveolar epithelial cell that produces surfactant. As these cell junctions get pulled apart and fluid and proteins enter the alveolus, they may not be able to produce surfactant, which can lead to increased surface tension and lung collapse. In addition, the fluid and protein that enter the alveolus can inactivate the surfactant that has been produced, also resulting in lung unit collapse and atelectasis. The mechanical stimulation of the alveolar epithelial and capillary endothelial cells can result in the elaboration of various cytokines and other active molecules into the systemic circulation which can induce a systemic inflammatory response syndrome (SIRS) reaction in various tissues and organs throughout the body.11a In the lung parenchyma this injury may manifest as widespread disruption of the endothelial and epithelial cells as well as plasma membrane blebbing and vesicle formation.
The impact of changes in lung volume and pressure are evident on a standard volume–pressure (compliance) curve. At low lung volume, there may be atelectasis of lung units, especially if there is not a minimum amount of PEEP to keep the alveoli and distal respiratory bronchioles from opening and closing with each breath. This process is termed atelectotrauma and can be prevented by using an amount of PEEP just greater than the lower inflection point on the volume-pressure cure. However, use of an inflation volume or pressure that is too high can produce overdistended alveoli. Unfortunately, the mechanism of PEEP and recruitment cause overdistended lung units. Recruitment is a process whereby the overdistention of adjacent alveoli pull open the neighboring collapsed alveoli. Figure 1 depicts the shear stress force that occurs at the small airways as a result of the alveoli being opened and closed. The shear stress may then lead to the formation of blebs and vesicles, which are manifestations of the mechanotransduction on the lung parenchyma.11 Evidence of the physical disruption that occurs during mechanotransduction is seen when propidium iodide is administered as a tracer. This molecule, when administered intravascularly, does not escape from the vasculature unless there has been a mechanical disruption. When experimental animals are ventilated with too large a tidal volume, histologic studies have demonstrated the presence of propidium iodide in the lung parenchyma,11
Figure 1. Ventilator-induced lung injury.
From Marini JJ Journal of Organ Dysfunction. 2007;3:224–231, with permission.
The disruptions in the tight junctions between the epithelial and endothelial cells have also been demonstrated with the use of the scanning electron microscope. The mechanical disruption or stress failure of the integrity of the capillary basement membrane can then result in fluid shifts from the vascular space into the alveolar space leading to interstitial and pulmonary edema.11 There is a relationship between the inflation volume, alveolar distention, and the size of the gap in tight junctions that subsequently results in the previously discussed leaks.12
The alveoli are normally very distensible, but their compliance can be altered by various disease processes. When the alveoli are caused to overdistend to a critical point, they may rupture and inhaled gas/air can then dissect back to the mediastinum via the bronchovascular bundle, or if the ruptured alveolus is near the pleural surface, the gas can enter into the pleural space.10 The presence of air in the mediastinum or the pleural space are both manifestations of barotrauma.10 The air in the mediastinum can then enter the subcutaneous space as it dissects up the fascial planes or possibly enter into the pericardial or peritoneal space.
Clinicians can contribute to the development of VILI by using too large a tidal volume, which results in increased microvascular permeability as a consequence of the stress failure of the tight gap junctions of the capillary endothelial cells and basement membrane. This failure leads to pulmonary edema, alveolar flooding, and a reduction in lung-distensible volume, leading to lung damage. In addition, if too low a tidal volume and PEEP are used in the setting of lung injury, there may be stress forces from the repetitive opening and closing of the lung units, which can lead to inflammation and mechanotransduction, resulting in distal lung damage. Figure 2 summarizes the potential pathophysiology of VILI.
Methods to Reduce Ventilator-Induced Lung Injury
One potential therapy to reduce VILI is PEEP, which may stabilize the lung unit so that the alveoli are not repetitively opening and closing. Using an experimental isolated rat lung model of lung injury, the addition of PEEP protected against the generation of various inflammatory cytokines, whereas high-volume ventilation without PEEP resulted in the largest production of inflammatory molecules.9 Similar results were observed in patients with ARDS who were ventilated with high VT versus using a lung-protective ventilation-support strategy. Patients ventilated with the high VT were found to have higher levels of various pro-inflammatory cytokines, such as tumor necrosis factor, interleukin (IL)-6, and IL-8 in their plasma, as well as, their bronchoalveolar (BAL) fluid, compared to patients ventilated with the lung-protective ventilation-support strategy (6 mL/kg of predicted body weight [PBW]).13
Lung protective ventilation involves using a small tidal volume (6 ml/kg ideal body weight) coupled with enough PEEP to prevent atelectotrauma and avoiding alveolar overdistention by keeping the end-inspiratory plateau pressure, a reflection of the alveolar pressure and possibly alveolar volume <30 cm H2O.14 When the end-inspiratory plateau pressure is kept below 30 cm H2O, alveolar overdistention should not occur. This form of ventilatory support contrasts with more traditional or conventional ventilation-support strategies of the past, which typically used larger VT (10-12 ml/kg measured body weight) with variable amounts of PEEP.14 While there was support for the lung protective ventilatory support strategy from early studies,14a,14b there was also evidence that this type of support did not significantly improve mortality.14c, 14d, 14e In 2000 investigators from the ARDS Network (ARDSNet) reported that using a lung-protective ventilation-support strategy with a tidal volume of 6 mL/kg of PBW versus 12 mL/kg of PBW led to a significant reduction in mortality—from 40%, down to 31%—for patients with ALI and ARDS.15 The ARDSNet study ventilatory support protocol used a PEEP nomogram to govern the amount of PEEP used based on the FIO2 and the goal of maintaining a PaO2 between 55 and 80 mm Hg.15 PEEP obviously has many beneficial effects, including increasing functional residual capacity, improving compliance, increasing oxygenation; recruiting atelectatic units; decreasing pulmonary venous admixture; and allowing a reduction in FIO2, which may decrease the risk for oxygen toxicity.14
Attempting to define the “best or optimum” amount of PEEP to use in the management of patients with acute lung injury and ARDS has been a controversial challenge for decades. The investigators from the ARDSNetork evaluated high PEEP versus their PEEP level used in their lung protective ventilation study in 549 patients with ALI and ARDS who were receiving lung protective ventilation.16 The authors found that there was no difference in the important outcomes of survival or being able to wean from the ventilator.16 Interestingly, they also did not find a significant difference in the development of barotrauma or other complications related to the higher PEEP levels versus the lower PEEP levels. Because there was no significant difference in outcome, the recommendation was to stick with the ARDSNet PEEP formula as per the original nomogram.
The optimum dosing of PEEP continues to be an area of controversy and investigation. A recent randomized controlled trial compared a strategy for keeping the PEEP between 5 cm and 9 cm H2O combined with a low-Vt ventilation-support strategy to a strategy designed to maintain the end-inspiratory plateau pressure between 28 and 30 cm H2O using a specific level of PEEP.17 The primary outcome was 28-day mortality rates, and there was no significant difference between the higher amount of PEEP, which might have been able to produce an increased recruitment of lung units compared to the more minimal distention of 5 to 9 cm
H2O of PEEP. Another randomized controlled trial compared a low-Vt ventilation-support strategy with a higher PEEP, recruitment maneuvers, and a plateau pressure of <40 cm H2O in 983 patients with ALI/ARDS.18 Allowing an end-inspiratory plateau pressure up to 40 cm H2O was an attempt to get more recruitment. The all-cause mortality, which was the primary endpoint, was essentially the same, as was the ability to wean patients from the ventilator. Both of these trials failed to improve on the 31% mortality rate for ALI and ARDS that was found in the ARDSNet trial. For now the ARDSNet protocol with PEEP titration nomogram continues to be the standard of care for managing these critically ill patients.
The use of lung-protective ventilation-support strategy with a tidal volume of 6 ml/kg predicted body weight and nomogram dosed PEEP has now become the standard of care in most centers. Additional support for this ventilatory support strategy comes from the Acute Respiratory Insufficiency España Study19—or ARIES—which compared lung-protective ventilation with conventional ventilation. In this trial the use of lung protective ventilation was also associated with a significant improvement in survival.
In a prospective observational study of 86 brain-injured patients, a greater proportion of those who were given higher Vts (eg, >11 mL/kg per PBW) than those given lower Vts developed ALI/ARDS.20 This may be evidence that using a lower Vt is protective even in patients who do not have lung injury. Patients with brain injuries are susceptible to neurogenic pulmonary edema and perhaps the lower tidal volume and PEEP strategy of the ARDSNetwork protocol is particularly beneficial in patients who are prone to the development of ALI and ARDS.
Although the use of neuromuscular blockers has declined in recent years because of the problems associated with them, an interesting study reported in 2006 looked at proinflammatory cytokines in either bronchoalveolar fluid or the blood of 36 patients with ALI who were treated with these drugs. They found that the amount of these proinflammatory substances was reduced when the patients were taking neuromuscular blockers.21 It is speculated that this observation may be the result of changes in the compliance of the chest wall and how much alveolar distention is occurring, even when patients are receiving standard Vts.
Beneficial Effects of Hypercapnia
Clinicians must recognize that when they provide lung-protective ventilation support, it will potentially result in reduced carbon dioxide elimination. This has been termed controlled hypoventilation or permissive hypercapnia.14 There are consequences of the resultant carbon dioxide (CO2) retention. For example, it can lead to respiratory acidosis and may worsen hypoxemia. It can lead to pulmonary vasoconstriction and pulmonary hypertension. It often leads to a sympathetic discharge, which results in an increased heart rate and hypertension and the potential for increased arrhythmias. The patient may need to have increased sedation because most patients do not like having CO2 retention. Some may even have problems with increased intracranial pressure. Consequently, patients with severe heart disease or increased intracranial pressure are not good candidates for this form of ventilatory support.
In addition, the hypercapnia that may develop from lung-protective ventilation support may have beneficial effects on VILI or the initial lung injury. An animal study in rabbits with ischemia reperfusion lung injury used wet-to-dry ratios as a surrogate for the degree of lung injury. The rabbits with hypercapnic acidosis had a lower wet to dry ratio (less lung water) than those animals that served as controls and did not have hypercapnic acidosis or that were made to develop metabolic acidosis.22 Those who would suggest that hypercapnic acidosis could be resolved by giving bicarbonate replacement should note that there was actually more lung water in the rabbits with buffered hypercapnia than in those that had hypercapnic acidosis.22
The potential benefits of hypercapnic acidosis include decreased tumor necrosis factor-alpha (TNFα) release by alveolar macrophages, decreased adherence between polymorphonuclear leukocytes and endothelial cells, decreased xanthine oxidase activity, reduced nuclear factor-kappa B (eg, the signal for the transcription of all these different inflammatory molecules), decreased nitric oxide synthase activity, and decreased production of IL-8 and toxic O2 radicals from polymorphonuclear leukocytes.23 To test the hypothesis that hypercapnic acidosis is associated with reduced mortality rates in patients with ALI, the 861 patients in the ARDSNet trial were divided into 2 groups: patients who received a Vt of 12 mL/kg of PBW and patient who received a Vt of 6 mL/kg of PBW (Table 2).23 The mortality rate for the standard-treatment patients who received a Vt of 12 mL/kg of PBW was 41% versus 31% for the standard-treatment patients who received a Vt of 6 mL/ kg of PBW. The small group of 11 patients, who even with a Vt of 12 mL/kg of PBW actually had respiratory acidosis with a PaCO2 between 45 and 65 mm Hg had a mortality rate of 18%. Hypercapnic acidosis in this patient population was associated with the best survival rate of all the groups. Although this is an interesting observation, it adds further support to the claim that hypercapnic acidosis in the setting of ALI and ARDS is quite beneficial.
Table 2. Hypercapnic Acidosis and Mortality in ALI
APACHE, Acute Physiology and Chronic Health Evaluation; ALI, acute lung injury; ARDS, acute respiratory distress syndrome; Pplat, plateau pressure; PEEP, positive end-expiratory pressure; HA3 is defined as 7.15 < pH < 7.35 and 45 mm Hg < Paco2 > 65 mm Hg.
ap < .05 compared with patients without HA3.
From Kregenow DA, Rubenfeld GD, Hudson LD, et al. Hypercapnic acidosis and mortality in acute lung injury. Crit Care Med. 2006;34:1-7, with permission
Using Evidence-Based Medicine
Although all clinicians working in the ICU believe that they are using the most current evidence-based medicine to treat their patients, a retrospective cohort was designed to see if clinical practice changed based on the availability of new evidence from the ARDSNet trial.24 The study evaluated the tidal volumes used to ventilate patients with ALI and ARDS in 3 large, New England, university hospitals prior to the ARDSNet trial and after it’s publication. One of the criticisms of the ARDSNet trial was that clinicians were no longer using large tidal volumes of 12 mL/kg. However, this study found that in the years prior to the publication of the ARDSNet results, New England clinicians were using a tidal volume of 12.3 mL/kg of PBW to ventilate patients with ALI and ARDS. Although they did reduce the tidal volume to an average of 10.6 mL/kg of PBW after the ARDSNet trial, the study concluded that the proportion of patients receiving Vts within recommended limits was modest.
Another study evaluated the tidal volumes used to ventilate patients with ALI and ARDS and looked at the size of the tidal volume as a function of the day of mechanical ventilatory support.25 They analyzed the patients based on the size of the tidal volume used, Vts of <6.5 mL/kg of PBW, Vts <7.5 mL/kg of PBW, or Vts <8.5 mL/kg of PBW. Of 88 patients studied, 39% had ventilation with a Vt of ≤7.5 mL/kg per PBW on day 2, 49% on day 4, and 56% on day 7.25 Both of these studies demonstrate that clinicians are somewhat slow to adopt this ventilatory-support technique, which was clearly demonstrated to improve survival of patients with ALI and ARDS by approximately 25%.15 The slow rate of adoption would suggest that this technique is still considered new, despite the fact that it has been around since 2000.
Strategies to Improve Oxygenation
When using low Vts and lung-protective ventilation strategies, clinicians may run into difficulties with worsening oxygenation. In that setting, some of the methods which can be employed to improve oxygenation include:
- Recruitment maneuver (eg, open-lung protective ventilation)
- Prone positioning
- Sighs
- High-frequency oscillation
- Inhaled nitric oxide
- Surfactant-replacement therapy
- Enhanced edema clearance
- Corticosteroids
Another strategy, first studied in the 1970s, is extracorporeal membrane oxygenation (ECMO) in an attempt to rest the injured lungs and allow them to heal.26a The ECMO trial sponsored by the National Institutes of Health assigned severely hypoxemic adult patients with ARDS to receive either ECMO or conventional therapy. Both groups had a 90% mortality rate.26a More recently, a study conducted in England called the Conventional Ventilatory Support vs Extracorporeal Membrane Oxygenation for Severe ARDS—or CESAR—Trial was reported in abstract form only at the January 2008 meeting of the Society of Critical Care Medicine.26 Patients from all over England were transferred to one center with the intention to be treated with an application of ECMO. Approximately one-quarter of the patients, by the time they arrived at the center, did not need to be treated with ECMO. In those who were randomized to the ECMO treatment, there was a 31% decrease in death or severe disability. The survival time until death was 3 times longer for patients who were treated with ECMO compared to conventional management. Unfortunately, the control group in this study was supposed to be managed using a low-volume lung-protective ventilation strategy, but only 63% of that group actually received lung-protective ventilation support versus 86% in the ECMO group. Another interesting report details the use of an artificial, pumpless, extracorporeal membrane ventilator to assist ventilation by providing passive complete carbon dioxide removal.26b Further study is still needed to determine if there is a role for ECMO and artificial lungs in managing patients with severe ARDS.
Outcome and Prognosis
Fortunately, the mortality rate associated with the use of lung-protective ventilatory-support strategies coupled with adequate amounts of PEEP is now approximately 30%, significantly better than the 60% to 90% mortality reported in the studies of the late 1960s and early 1970s.27,27a The cause of death is typically related to recurrent sepsis or from multiorgan dysfunction syndrome (MODS) or multiple organ failure.27b28 Survivors typically have mild to moderate pulmonary function abnormalities, including a restrictive defect and diffusion abnormality. Most pulmonary function improvement occurs during the 3 months post-extubation. Patients with ARDS tend to have a decreased health-related quality of life and an increased level of respiratory symptoms (as judged by the Medical Outcomes Study 36-Short Form General Health Survey – SF 36).28a In addition, ARDS survivors have an increased incidence of post-traumatic stress disorder, anxiety, depression, and insomnia.28b A meta-analysis of 72 studies conducted over a 12-year period showed that the mortality rate has decreased approximately 1.1% per year during the study period.28 Older people are more likely than younger patients to die from ALI or ARDS. Specifically, patients who are 70 years of age or older were twice as likely to die of ALI than their younger counterparts.29
Summary
VILI can develop through various mechanisms. Accordingly, it is important for clinicians to avoid alveolar overdistention. To that end, they should monitor the end-inspiratory plateau pressure and keep it at ≤30 cm of H2O. They should also use lung-protective ventilatory-support strategies with adequate amounts of PEEP when managing a patient with ALI or ARDS. The goal of ventilatory support is maintenance of adequate oxygenation and an adequate tissue oxygen delivery. The use of adequate amounts of PEEP may also be important to the healing process and to prevent further VILI. Clinicians should realize that hypercapnia may have a beneficial effect on patients. After recovering from such a grave injury, survivors will typically have mild to moderate pulmonary limitation and be deconditioned.
References
1. Fan E, Needham DM, Stewart TE. Ventilatory management of acute lung injury and acute respiratory distress syndrome. JAMA. 2005;294:2889–2896.
1a. Ashbaugh DG, Bigelow DB, Petty TL, et al. Acute respiratory distress in adults. Lancet. 1967;2:319-323.
1b. Ware LB, Matthay MA. The acute respiratory distress syndrome. N Engl J Med. 2000;342:1334-1349.
1c. Murray JF, Matthay MA, Luce JM, Flick MR. An expanded definition of the adult respiratory distress syndrome. Am Rev Respir Dis. 1988;138:720-723.
1d. Goss CH, Brower RG, Hudson LD, et al. Incidence of acute lung injury in the United States. Crit Care Med. 2003;31:1607-1611.
2. Bernard GR, Artigas A, Brigham KL, et al. The American-European Consensus Conference on ARDS. Definitions, mechanisms, relevant outcomes, and clinical trial coordination. Am J Respir Crit Care Med. 1994;149:818–824.
3. Bersten AD, Edibam C, Hunt T, et al; Australian and New Zealand Intensive Care Society Clinical Trials Group. Incidence and mortality of acute lung injury and the acute respiratory distress syndrome in three Australian states. Am J Respir Crit Care Med. 2002;165:443–448.
4. Rubenfeld GD, Neff MJ. Epidemiology of acute lung injury: a public health perspective. In: Matthay MA, editor. Acute Respiratory Distress Syndrome. New York: Marcell Dekker; 2003;40.
5. Rubenfeld GD, Caldwell E, Peabody E, et al. Incidence and outcomes of acute lung injury. N Engl J Med. 2005;353:1685–1693.
6. Rubenfeld GD, Caldwell E, Granton J, et al. Interobserver variability in applying a radiographic definition for ARDS. Chest. 1999;116:1347–1353.
7. Esteban A, Fernández-Segoviano P, Frutos-Vivar F, et al. Comparison of clinical criteria for the acute respiratory distress syndrome with autopsy findings. Ann Intern Med. 2004;141:440–445.
8. Ferguson ND, Frutos-Vivar F, Esteban A, et al. Acute respiratory distress syndrome: under-recognition by clinicians and diagnostic accuracy of three clinical definitions. Crit Care Med.2005;33:2228–2234.
9. Dreyfuss D, Saumon G. Ventilator-induced lung injury. Am J Respir Crit Care Med.1998;157:294–323.
10. Pinhu L, Whitehead T, Evans T, et al. Ventilator-associated lung injury. Lancet. 2003;361:332–340.
10a. Webb HH, Tierney DF. Experimental pulmonary edema due to intermittent positive pressure ventilation with high inflation pressures: Protection by positive end-expiratory pressure. Am Rev Respir Dis. 1974;110:556-565.
10b. Kolobow T, Moretti MP, Fumagalli R, et al. Severe impairment in lung function induced by high peak airway pressure during mechanical ventilation: An experimental study. Am Rev Respir Dis. 1987;135:312-315.
10c. Dreyfus D, Soler P, Sammon G. Mechanical ventilation induced pulmnary edema: Interaction with previous lung alterations. Am J Respir Crit Care Med. 1995;151:1568-1575.
11. Vlahakis NE, Hubmayr RD. Cellular stress failure in ventilator-injured lungs. Am J Respir Crit Care Med. 2005;171:1328–1342.
11a. Bone RC, Balk RA, Cerra FB, et al. Definitions for sepsis and organ failure and guidelines for the use of innovative therapies in sepsis. Chest. 1992;101:1644-1655.
12. Parker JC, Hernandez L, Peevy KJ. Mechanisms of ventilator-induced lung injury. Crit Care Med. 1993;21:131–143.
13. Ranieri VM, Suter PM, Cosimo T, et al. Effects of mechanical ventilation on inflammatory mediators in patients with acute respiratory distress syndrome. JAMA. 1999;282:54–61.
14. Malhotra A. Low-tidal-volume ventilation in the acute respiratory distress syndrome. N Engl J Med. 2007;357:1113–1120.
14a. Hickling KG, Walsh J, Henderson S, et al. Low mortality rate in adult respiratory distress syndrome using low-volume, pressure-limited ventilation with permissive hypercapnia: A prospective study. Crit Care Med. 1994;22:1568-1578.
14b. Amato MBP, Barbas GSV, Medeiros DM, et al. Effect of a protective-ventilation strategy on mortality in the acute respiratory distress syndrome. N. Engl J Med. 1998;338:347-54.
14c. Stewart TE, Meade MO, Cook DJ, et al. Evaluation of a ventilation strategy to prevent barotrauma in patients at high risk for acute respiratory distress syndrome. N Engl J Med. 1998;338:355-361.
14d. Brochard L, Roudot-Thoraval F, Roupie E, et al. Tidal volume reduction for prevention of ventilator-induced lung injury in acute respiratory distress syndrome. The multicenter trial group on tidal volume reduction in ARDS. Am J Respir Crit Care Med. 1998;158:1831-1838.
14e. Brower RG, Shanholtz CB, Fessler HE, et al. Prospective, randomized, controlled clinical trial comparing traditional versus reduced tidal volume ventilation in acute respiratory distress syndrome. Crit Care Med. 1999;27:1492-1498.
15. ARDSNet. Ventilation with lower tidal volumes as compared with traditional tidal volumes for acute lung injury and the acute respiratory distress syndrome. N Engl J Med 2000;342:1301–1308.
16. National Heart, Lung, and Blood Institute ARDS Clinical Trials Network. Higher versus lower positive end-expiratory pressures in patients with the acute respiratory distress syndrome. N Engl J Med. 2004;351:327–336.
17. Mercat A, Richard JC, Vielle B, et al. Positive end-expiratory pressure setting in adults with acute lung injury and acute respiratory distress syndrome: a randomized controlled trial. JAMA. 2008;299:646–655.
18. Meade MO, Cook DJ, Guyatt GH, et al. Ventilation strategy using low tidal volumes, recruitment maneuvers, and high positive end-expiratory pressure for acute lung injury and acute respiratory distress syndrome: a randomized controlled trial. JAMA. 2008;299:637– 645.
19. Villar J, Kacmarek RM, Pérez-Méndez L. A high positive end-expiratory pressure, low tidal volume ventilatory strategy improves outcome in persistent acute respiratory distress syndrome: a randomized, controlled trial. Crit Care Med. 2006;34:1311–1318.
20. Mascia L, Zavala E, Bosma K, et al. High tidal volume is associated with the development of acute lung injury after severe brain injury: an international observational study. Crit Care Med. 2007;35:1815–1820.
21. Forel JM, Roch A, Marin V, et al. Neuromuscular blocking agents decreases inflammatory response in patients presenting with acute respiratory distress syndrome. Crit Care Med. 2006;34:2749–2757.
22. Laffey JG, Engelberts D, Kavanagh BP. Buffering hypercapnic acidosis worsens acute lung injury. Am J Respir Crit Care Med. 2000;161:141–146.
23. Kregenow DA, Rubenfeld GD, Hudson LD, et al. Hypercapnic acidosis and mortality in acute lung injury. Crit Care Med. 2006;34:1–7.
24. Young MP, Manning HL, Wilson DL, et al. Ventilation of patients with acute lung injury and acute respiratory distress syndrome: has new evidence changed clinical practice? Crit Care Med. 2004;32:1260–1265.
25. Kalhan R, Mikkelsen M, Dedhiya P, et al. Underuse of lung protective ventilation: analysis of potential factors to explain physician behavior. Crit Care Med. 2006;34:300–306.
25a. Zapol WM, Snider MT, Hill JD, et al. Extracorporeal membrane oxygenation in severe acute respiratory failure. A randomized prospective study. JAMA. 1979;242:2193-2196.
26. Dalton HJ, Peek G, Riker RR, et al. The CESAR Trial: Conventional Ventilatory Support Versus Extracorporeal Membrane Oxygenation for Severe Adult Respiratory Failure. Presented at the 37th Critical Care Congress, Society of Critical Care Medicine, Honolulu, HI: February 2–6, 2008.
26b. Iglesias M, Martinez E, Badia JR, Macchiarini P. Extrapulmonary ventilation for unresponsive severe acute respiratory distress syndrome after pulmonary resection. Ann Thorac Surg. 2008;85:237-244.
27. Angus DC, Musthafa AA, Clermont G, et al. Quality-adjusted survival in the first year after the acute respiratory distress syndrome. Am J Respir Crit Care Med. 2001;163:1389–1394.
27a. Milberg JA, Davis DR, Steinberg KP, et al. Improved survival of patients with acute respiratory distress syndrome (ARDS): 1983-1993. JAMA. 1995;273:306-309.
27b. Montgomery BA, Stager MA, Carrico J, et al. Causes of mortality in patients with the adult respiratory distress syndrome. Am Rev Respir Dis. 1985;132:485-491.
28. Zambon M, Vincent JL. Mortality rates for patients with acute lung injury/ARDS have decreased over time. Chest. 2008;133:1120–1127.
28a. Herridge MS, Cheung AM, Tansey CM, et al. One-year outcomes in survivors of the acute respiratory distress syndrome. N Engl J Med. 2003;348:683-693.
28b. Davidson TA, Caldwell ES, Curtis JR, et al. Reduced quality of life in survivors of acute respiratory distress syndrome compared with critically ill control patients. JAMA. 1999;281:354-360.
29. Ely EW, Wheeler AP, Thompson BT, et al, for the Acute Respiratory Distress Syndrome Network. Recovery rate and prognosis in older persons who develop acute lung injury and the acute respiratory distress syndrome. Ann Intern Med. 2002;136:25–36.
Self-Assessment
- To decrease the chances of ventilator-induced lung injury (VILI), clinicians should focus on which of the following strategies?
(a) A lung-protective ventilation-support strategy using no positive end-expiratory pressure (PEEP)
(b) A lung-protective ventilation-support strategy using low PEEP
(c) A lung-protective ventilation-support strategy using high PEEP
(d) None of the above
- True or false: The hypercapnia that may develop from lung-protective ventilation support may have beneficial effects on VILI.

Full access? Get Clinical Tree
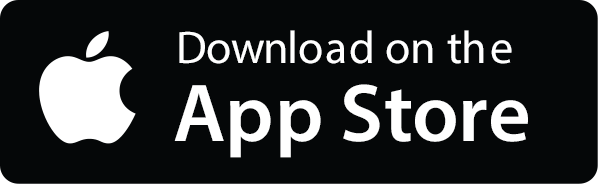
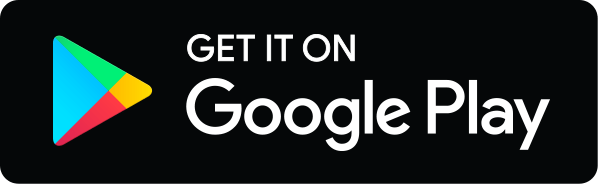