FIGURE 71.1 Volume of lung transplants in the United States. There has been significant increase in the volume of lung transplantation in the past decade, mostly due to increase in the number of bilateral lung transplant operations. (From U.S. Organ Procurement and Transplantation Network and the Scientific Registry of Transplant Recipients.)
Selection Criteria for Suitable Candidates
Although transplantation has been successful in most lung diseases, not all patients with advanced lung diseases are suitable candidates. The patient’s medical records are screened carefully prior to the initial clinic visit. Patients are often declined at the initial clinic visit if there is any history of cigarette smoking in the last 6 months or if there is evidence of irreversible extrapulmonary end-organ damage. Patients are also screened for their insurance coverage, as lung transplantation is an expensive endeavor. Medicare and most state Medicaid and private insurers provide coverage (Table 71.3). After an initial screening of the patient’s records, the potential lung recipients are seen in the lung transplant clinic. A detailed discussion of lung transplantation, its outcome, and potential complications occurs with the patient if there are no obvious contraindications identified during the clinic visit. If the patient consents to the transplant procedure, he or she is then referred for a more detailed transplant evaluation and testing. Lung transplant evaluation and the guidelines for the selection of recipients are listed in Tables 71.3, 71.4, and 71.5 respectively (12). A body mass index (BMI) greater than 30 has been identified as an independent predictor of mortality in the first 12 months post transplantation (13,14). However, BMI greater than 30 is a relative contraindication and must be considered in the context of muscle strength, as more recent data shows that BMI up to 34.9 has no effect on survival (15). Chronic obstructive pulmonary disease (COPD) patients are likely to be scrutinized the closest for BMI and muscle strength, as improvement in quality of life is the indication in the majority of patients in this disease category. Patients who are suitable for lung transplantation are listed with the United Network for Organ Sharing (UNOS).
TABLE 71.1 Criteria for “Ideal” Lung Donors |
![]() |

FIGURE 71.2 Worldwide adult lung transplants major indications by year. There has been a significant increase in the volume of lung transplant in the United States and worldwide. The number and proportion of patients with ILD has also increased since the implementation of the LAS score in 2005. (From the International Society of Heart Lung Transplantation, with permission.)
TABLE 71.2 Disease-Specific Guidelines for Referral |
![]() |
TABLE 71.3 Primary Payers of Adult Lung Transplant Recipients in the United States in 2012 | |
![]() |
TABLE 71.4 Transplant Evaluation Testing |
![]() |
TABLE 71.5 General Guidelines for Selection of Recipients |
![]() |
SURGICAL TECHNIQUES FOR LUNG TRANSPLANTATION
Isolated Lung Transplant
Dr. James Hardy from University of Mississippi reported the first lung transplantation in a human in 1963. Many failed attempts thereafter were mainly due to infections and anastomotic dehiscence from the high doses of steroids used for immunosuppression. The introduction of cyclosporine, in the early 1980s, allowed for reduction of steroid doses. Dr. Joel Cooper from University of Toronto reported successful lung transplantation in 1983. Points to remember include lung recruitment maneuvers upon visualization, as well as adequate heparinization, steroid, and prostaglandin administration. After discussions with the heart procurement team, it is important to retrieve pulmonary artery and vein of appropriate length.
Recipients are usually listed with a height range of ±20%, as height and gender are the primary determinant for lung volumes. Lung transplant centers usually prefer to implant lungs from taller donors into recipients with obstructive lung diseases (COPD, cystic fibrosis [CF]), and lungs from shorter donors into recipients with restrictive lung diseases (idiopathic pulmonary fibrosis [IPF]). Bilateral lung transplantation (BLT) is performed in approximately 75% of COPD patients, 60% of ILD patients, 99% of CF patients, and in 95% of idiopathic pulmonary artery hypertension (iPAH) patients.
There are four lung transplant surgical procedures: single-lung transplantation (SLT), bilateral sequential lung transplantation (BLT), combined heart–lung transplantation (HLT), and bilateral lobar transplantation from living-related donors. Higher rates of intrathoracic bleeding, reexploration, and renal dysfunction are to be expected in patients with previous chest procedures, mainly due to longer time in explanting the native lung. These conditions may be exacerbated if patients require cardiopulmonary bypass (CPB). However, a history of previous chest surgeries is not felt to be an absolute contraindication to lung transplantation. Historically, SLT used to be the most applied technique, but lately BLT has become more prevalent. The advantages of SLT include a shorter waiting time for the recipient, technical ease, and the fact that lungs from one donor can be potentially used for two recipients. With the exception of suppurative lung diseases, SLT has been applied to all lung diseases.
There are different approaches for transplantation depending upon whether single or double lung transplant is considered: posterolateral thoracotomy, anterolateral thoracotomy, sternotomy, or clamshell incision. Procedures can be performed on CPB or extracorporeal membrane oxygenation (ECMO), depending on perfusion mismatch and pulmonary artery pressures. Patients with moderate to severe pulmonary hypertension are more likely to need bypass. Pulmonary artery pressure always increases when the pulmonary artery is clamped. Nitric oxide or inhaled epoprostenol can be used to assist in lowering pulmonary pressures.
Once donor lungs are visualized and determined to be suitable for transplantation, recipient surgery is begun. To avoid hypoxemia due to shunting through the deflated lung during dissection, the pulmonary artery may be clamped first. The recipient pneumonectomy is performed, and the hilum is prepared for anastomosis, providing enough length to clamp pulmonary artery and vein, and still be able to perform anastomosis. This anastomosis can be done without clamping if CPB is used. The main stem bronchus is transected just proximal to upper lobe takeoff, taking care to achieve hemostasis with not only dissected lymph nodes, but bronchial arteries. The phrenic, vagus, and recurrent laryngeal nerves must be spared.
The donor lung hilum is fashioned for transplant on the back table and the pulmonary vasculature is flushed retrograde to remove any emboli. The bronchus staple line is removed and airways are suctioned free. The donor lung is then brought up to be implanted. The three anastomoses are hand sewn in their posterior-to-anterior anatomic sequence: bronchus, pulmonary artery, and pulmonary veins—left atrium. The bronchial blood supply to the donor lung is disrupted during transplantation, so the donor bronchus is dependent upon retrograde bronchial blood flow through the pulmonary circulation, unless direct bronchial revascularization is performed. Most centers do not perform bronchial revascularization due to technical difficulty in anastomosing the bronchial arteries.
Mannitol and high-dose methylprednisolone are given once all anastomoses are completed. Prior to clamps being removed, de-airing maneuvers are performed via steep Trendelenburg positioning, CO2 flooded on the field, and visualization via echocardiogram. The implanted lung is then ventilated as it is being perfused.
Function is confirmed via blood gas, hemostasis is achieved, and chest tubes are placed, followed by closure. Intraoperative bronchoscopy is performed at the end to visualize not only anastomosis, but to clear secretions, evaluate for torsion, and assess for development of primary graft dysfunction (PGD) manifested with severe pulmonary edema. Postoperative pulmonary vein thrombosis, a rather uncommon complication, may manifest as persistent pulmonary edema (in spite of adequate diuresis).
Heart–Lung Transplant
In 1981, the first HLT was performed in a patient with pulmonary arterial hypertension. The only absolute indication for this procedure is Eisenmenger syndrome associated with complex congenital heart disease. Other indications are patients with concurrent significant LV dysfunction and advanced lung disease, and idiopathic pulmonary hypertension with severe right ventricular failure. The latter is an uncommon indication for this type of surgery as the majority of RV dysfunction recovers after isolated BLT (Fig. 71.3). The implantation itself is en bloc on CPB, either via sternotomy or clamshell incision. Tracheal anastomosis is performed first, followed by bicaval anastomosis, then aorta. Techniques can be inferred from individual organ implantation.

FIGURE 71.3 Intraoperative transesophageal echocardiogram (TEE) in a patient with iPAH undergoing BLT. Note the reduction of the size of the right atrium (RA) and right ventricle (RV) at end systole about 1 hour after reperfusion of the lungs.

FIGURE 71.4 Induction immunosuppression. Use of induction immunosuppression (in addition to intraop pulse methylprednisolone) in adult lung transplant recipients. Data from January 2002 to June 2013. (From the International Society of Heart Lung Transplantation, with permission.)
Living-Related Lobar Transplant
About 130 of these transplantations have been performed in the United States since 1991. This technique is generally reserved for patients who meet all the criteria for lung transplantation, but have a minimal chance of surviving the waiting time on the lung transplant waiting list. Donors are typically family members who undergo full evaluation for thoracotomy. Because this procedure presents risks to two individuals, appropriate recipient and donor selection, and timing of transplantation, are critical to minimize the morbidity to the donor and maximize the chance of a successful outcome in the recipient.
IMMUNOSUPPRESSION
Induction therapy is intensive immunosuppressant therapy given at the time of transplant to reduce the risk of acute rejection and to delay initiation of maintenance immunosuppression. An intravenous bolus of 500 to 1,000 mg of methylprednisolone is given in the operating room. Most centers use another agent for induction, with the interleukin-2 receptor antagonists (basiliximab) being most common, followed by antilymphocyte antibody (thymoglobulin, alemtuzumab) (Fig. 71.4). Some studies have shown a survival benefit with induction therapy (16) as well as significant decrease in acute rejection early after transplant without significant increase in infection rates (17,18) (Fig. 71.5).
Maintenance immunosuppression is lifelong therapy given to prevent acute and chronic rejection. Doses and medications are changed and adjusted to minimize their side effects. Table 71.6 details the numerous side effects, interactions, and dosing of the immunosuppressive medications. Triple immunosuppression with calcineurin inhibitor, antiproliferative agent, and corticosteroids is the rule for maintenance immunosuppression. The most commonly used agents in the last 5 years are: tacrolimus (Tac), mycophenolate mofetil (MMF), and prednisone (Fig. 71.6). Tac has been shown to reduce the incidence of bronchiolitis obliterans syndrome (BOS) when compared to cyclosporine, but without any differences in acute rejection incidence, or survival, after 3 years (19). However, another study compared a triple immunosuppression maintenance therapy containing CyA to tacrolimus and found that the number of episodes of acute rejection per 100 patient days was lower in the tacrolimus group (20). In two prospective trials comparing azathioprine and MMF, Palmer et al. showed no difference in acute rejection rates in the first 6 months while McNeil et al. showed no difference in acute or chronic rejection at 3 years (21,22).

FIGURE 71.5 Survival was calculated using the Kaplan–Meier method, which incorporates information from all transplants for whom any follow-up has been provided. Survival rates were compared using the log-rank test statistic. (From the International Society of Heart Lung Transplantation, with permission.)
TABLE 71.6 Commonly Used Immunosuppressive Medications | |||
![]() |

FIGURE 71.6 Maintenance immunosuppression in adult lung transplant recipients in the United States. Note in the last decade the shift of calcineurin inhibitors and antimetabolites to tacrolimus and mycophenolate mofetil, respectively. Also note the increased use of induction agents at the time of transplantation. (From U.S. Organ Procurement and Transplantation Network and Scientific Registry of Transplant Recipients.)

FIGURE 71.7 Adult lung transplants. Procedure type within indication, by year, from 1998 to 2012. Blue indicated bilateral double lung transplant; red indicates single lung transplant. (From the International Society of Heart Lung Transplantation, with permission.)
The calcineurin inhibitor cyclosporine A (CyA) is sometimes substituted for Tac or azathioprine for MMF in patients intolerant of their side effects (especially GI symptoms). Sirolimus is substituted for calcineurin inhibitors in some patients with kidney injury with amelioration of kidney injury and seemingly preserved lung function (23).
OUTCOMES FOLLOWING LUNG TRANSPLANTATION
Survival
The median survival after lung transplantation is 5.6 years. The 1-, 3-, 5-, and 10-year actuarial survivals after lung transplantation are 80%, 69%, 53%, and 30%, respectively (1). BLT is being applied much more frequently than SLT in the last decade (Fig. 71.7). BLT has significantly higher survival compared to SLT (Fig. 71.8). The survival is also significantly better in lung transplant programs doing higher volumes (Fig. 71.9) (13,24). However, these survival rates lag considerably behind those of liver or kidney transplant recipients, for whom the 5-year actuarial survival approximates 80%. In the absence of prospective randomized trials, it is difficult to ascertain whether lung transplantation truly increases survival over the natural history of the lung disease. Hosenpud et al. (25) made a disease-specific comparison of survival after transplantation and found that patients with CF and IPF benefit from transplantation. No such advantage has yet been demonstrated for patients with COPD, a disease that typically follows a protracted course with a 5-year survival of 40% to 50% for patients with forced expiratory volume in 1 second (FEV1) less than 20% of predicted (26). Thus, the main indication for lung transplantation in patients with COPD is to improve quality of life in patients with FEV1 in the 15% to 25% range, rather than prolonging survival. BODE index (body mass index [B], airflow obstruction [O], dyspnea [D], and exercise capacity [E]) has been identified as a predictor of survival and rehospitalization in COPD patients (27).
There have been a few risk factors identified by multivariate analysis that are associated with risk of death 1 year after transplantation: diagnosis of iPAH or IPF, increased severity of recipient illness at the time of transplantation (i.e., ventilator dependence, ICU care), transplant volume less than 30 per year, older recipient age at the time of transplant (especially above 60 years of age), higher pretransplant supplemental oxygen, lower cardiac index, and retransplantation (1). However, it is important to remember that those statistics are aggregate data, reported by multiple centers to the International Society for Heart and Lung Transplantation (ISHLT). Since different centers have different expertise and experience, those risk factors may not be uniform among centers. For example, in carefully selected ventilator-dependent patients who are not critically ill, mechanical ventilation did not influence the 1-year outcome after transplantation (28,29). The leading causes of death in the first 30 days after transplantation are primary allograft failure and bacterial pneumonia, with infection being the leading cause of death in the first year (Fig. 71.10) (1). Chronic lung rejection is the most common cause of death in patients surviving past the first year after transplantation and occurs in 50% of recipients surviving 5 years after transplantation (Fig. 71.11).
Pulmonary Function and Gas Exchange
Both SLT and BLT patients result in significant improvement in pulmonary function tests (PFTs) and in gas exchange post transplantation. The peak improvement in PFTs is achieved at 1 to 3 months after SLT and in 4 to 6 months after BLT. The factors associated with the delay in achieving peak values are reperfusion injury, postoperative pain, altered chest wall mechanics, and respiratory muscle dysfunction after transplant surgery. After SLT in patients with COPD, the FEV1 significantly improves to 45% to 60% of the predicted value and lung volumes approach the normal predicted values, with associated midline shift on chest radiograph (Figs. 71.12 and 71.13) (30). After SLT in patients with IPF, the vital capacity improves to about 70% to 80% of the predicted value, with associated midline shift of the allograft into native chest cavity (Figs. 71.14 and 71.15) (30). After BLT, the spirometry values approximate predicted values for the recipient’s chest cavity size and the chest radiograph will show normal lung fields (Figs. 71.16 and 71.17).

FIGURE 71.8 Adult lung transplants (January 1994 to June 2012). Survival was calculated using the Kaplan–Meier method. Survival rates were compared using the log-rank test statistic. The conditional median survival is the estimated time point at which 50% of the recipients who survive to at least 1 year have died. (From the International Society of Heart Lung Transplantation, with permission.)

FIGURE 71.9 Adult lung transplant center volume and hazard ratio for mortality within the first posttransplant year (January 2000 to June 2012). Dashed lines are 95% confidence intervals. (From the International Society of Heart Lung Transplantation, with permission.)

FIGURE 71.10 Infection is the leading cause of death in the first year, while chronic lung rejection is the leading cause of death in patients who live past the first year, data collected 1992–2013. Most of Graft failure is due to PGD in the first year, and due to chronic lung rejection beyond the first year. Bronchiolitis is chronic lung rejection. (From the International Society of Heart Lung Transplantation, with permission.)

FIGURE 71.11 Freedom from bronchiolitis obliterans syndrome (BOS) rates were computed using the Kaplan–Meier method. Data from 1994 to 2012, conditional on patients surviving 14 days. (From the International Society of Heart Lung Transplantation, with permission.)

FIGURE 71.12 FEV1, FVC, and TLC in six COPD patients before and after single lung transplantation. Note the significant improvement in FEV1 and in the decline in TLC.
After lung transplantation, in allografts with minimal or no injury immediately post transplant, arterial oxygenation and hypercapnia return to normal within a few days. Supplemental oxygen is unnecessary for most patients at the time of hospital discharge.
Hemodynamics
In patients with significant pulmonary hypertension (iPAH, or pulmonary hypertension associated with ILD), BLT has been adopted as the procedure of choice in most centers. Between 1995 and 2008, 714 patients have undergone BLT, and 94 patients SLT, according to the ISHLT registry report. BLT results in immediate and sustained normalization of pulmonary artery pressures in this population, barring significant reperfusion injury. This is associated with total or near-total resolution of the associated tricuspid valve insufficiency and is accompanied by an immediate increase of the cardiac output with a gradual remodeling of the right ventricle (see Fig. 71.3). The right ventricle decreases in size and regains normal function over the next few days to weeks. This hemodynamic improvement is sustained after successful transplantation. The advantage of BLT over SLT for patients with pulmonary vascular disease is that airway injury is better tolerated in BLT. Due to the resultant severe ventilation/perfusion mismatch, airway injury after SLT (caused by pneumonia, or acute or chronic rejection) may result in profound hypoxemia, especially in patients with severe pulmonary hypertension (31).

FIGURE 71.13 Chest radiograph after right single lung transplant in a patient with COPD. Note the more compliant hyperinflated native COPD lung with flat diaphragm and (clinically asymptomatic) shift across the midline into the right allograft side.
Quality of Life
Several studies show a dramatic, global improvement in all measures of quality of life (physical, social, and psychological) in most recipients as early as 3 months after transplantation. These improvements are maintained or continue to improve over the next several years in patients who maintain lung function (Fig. 71.18) (32–35). However, because bronchiolitis obliterans (BO) causes progressive deterioration of lung function, any newly acquired improvement in the quality of life deteriorates if BO occurs after transplantation (36). Only approximately one-third of recipients return to work after transplantation. Factors that may contribute to this relatively low reemployment rate include potential reluctance to hire employees with complex medical conditions, potential loss of income or medical benefits as a result of returning to work, and the change of priorities and goals of recipients after recovering from their previous incapacitating condition (Fig. 71.19) (37).

FIGURE 71.14 Vital capacity in patients with IPF undergoing SLT, resulting in a significant improvement in lung volumes.

FIGURE 71.15 Chest radiograph in a patient with IPF who underwent single left lung transplantation. Note the mild midline shift (clinically asymptomatic) of the more compliant left allograft into the right chest cavity containing the native lung.
Exercise Capacity
Peak exercise performance, as measured by cycle ergometry, is characteristically reduced in both SLT and BLT recipients as late as 1 or 2 years after the surgery. These patients have subnormal peak work rate, peak oxygen consumption, and early lactate threshold on incremental exercise testing. Maximal oxygen consumption is significantly increased after lung transplantation, but it typically is 40% to 60% of the maximal predicted value. Interestingly, the limitation to exercise is not cardiac or ventilatory in nature, and, surprisingly, the maximal oxygen consumption attained during exercise testing is similar whether the recipients are of SLT or BLT (38). Reduced mitochondrial oxidative capacity and peripheral oxygen use in the skeletal muscles of lung transplant recipients (39–41) may be secondary to the deconditioning associated with chronic lung diseases or to impairment in skeletal muscle mitochondrial respiration associated with calcineurin inhibitors (42–44).

FIGURE 71.16 FEV-1 and FVC in five patients with cystic fibrosis before and after transplant. Values are percentage of predicted.

FIGURE 71.17 A: Pretransplant chest radiograph in a patient with CF showing bilateral bronchiectasis, severe left lower lobe necrosis and volume loss with shift of the heart into the left hemithorax. B: Three months after bilateral lung transplant.

FIGURE 71.18 Functional status is collected using Karnofsky score for adult recipients and Lansky score for pediatric recipients. This figure shows the functional status reported on the 1-year, 2-year, and 3-year annual follow-ups. Because all follow-ups between March 2005 and June 2013 were included, the bars do not include the same patients. (From the International Society of Heart Lung Transplantation, with permission.)

FIGURE 71.19 Employment status reported on the 1-year, 3-year, and 5-year annual follow-ups. Because all follow-ups between April 1994 and June 2013 were included, the bars do not include the same patients. (From the International Society of Heart Lung Transplantation, with permission.)
COMMON COMPLICATIONS AFTER LUNG TRANSPLANTATION
Primary Graft Dysfunction
PGD is a form of acute lung injury occurring in the first few days after lung and is due to a spectrum of diseases (45). PGD represents a spectrum of diseases characterized to variable degree by fever, hypoxemia, pulmonary hypertension, hypotension, pulmonary edema, and acute kidney injury. The incidence of severe PGD is about 15% and is a common cause of mortality within the first posttransplant year. Severe PGD leads to increased duration of mechanical ventilation and intensive care unit length of stay, poor functional outcomes, and increased risk of BOS. The differential diagnosis includes ischemia–reperfusion injury, hyperacute rejection, donor-related bacterial pneumonia, pulmonary vein thrombosis, or edema from acute kidney injury. The chest radiograph and clinical scenario are almost identical in all these etiologic factors, that is, all have widespread interstitial and alveolar infiltrates in the allograft(s) and hypoxemia. Severity of PGD is graded by PaO2/FiO2 ratio and chest radiographic abnormalities at 24 and 72 hours. The diagnosis of reperfusion injury is established by excluding other causes of graft dysfunction. An algorithm for the workup of reperfusion injury is summarized in the article by Zander et al. (46). Inhaled nitric oxide, independent lung ventilation, and extracorporeal membrane oxygenation have been attempted with variable success for patients with severe PGD. The clinical course in survivors of severe PGD is protracted, but some of the survivors are able to ultimately attain normal allograft function. Variables associated with severe PGD are related to donor characteristics (such as smoking history), recipient characteristics (such as presence of pulmonary hypertension), and in patients requiring prolonged CPB runs (47). As PGD is often characterized by leaky capillaries and decreased cardiac output, medical management should be aimed at preserving and optimizing kidney function in the first 72 hours; this is best achieved by avoiding nephrotoxic agents and avoiding hypotension. The latter is perhaps best achieved by using low-dose inotropic agents as first-line therapy (i.e., low-dose epinephrine) especially when the cardiac output is low. If hypotension persists, then measuring systemic vascular resistance (SVR) will guide the next step: low-dose vasopressin if SVR is low, or judicious use of intravenous fluids or blood products if SVR is high. This strategy aims to minimize fluid overload and maximize kidney perfusion without using high dose of vasoconstrictors or large volumes of intravenous fluids. Retransplantation has been attempted for patients with severe PGD with variable success.
Airway Complications
Airway complications requiring intervention occur in 7% to 15% of bronchial anastomosis (48). This reflects both improved surgical techniques that occurred with the advent of bronchial telescoping and relatively lower steroid doses compared to those used with transplantation in the 1970s and early 1980s. Complete dehiscence of the bronchial anastomosis, rare at present, is an emergency situation that requires immediate surgical intervention. Anastomotic stenosis is the most common airway complication, followed by bronchomalacia. Most airway complications manifest in the first 100 days after transplantation. They may present as wheezing, exertional dyspnea, or decline in FEV1. As the bronchial arteries are not reconnected at the time of transplantation, the bronchial mucosa distal to the anastomosis could be affected by bronchial artery reperfusion injury. This manifests as bronchial mucosal injury with ischemic mucosal surface. The mucosal injury may heal with minimal sequelae, thereby resulting in airway stricture, or, if the bronchial artery ischemia injures the underlying cartilaginous support of the bronchus, it may cause bronchomalacia. Alternatively, bronchial stenosis may be caused by overgrowth of the scar tissue at the site of the bronchial anastomosis. Thus, airway complications may be at the anastomotic site or a few centimeters distally. Bronchial mucosal injury has the bronchoscopic appearance of a pseudomembrane, which is sometimes superinfected with fungal organisms (Fig. 71.20). Most airway complications are amenable to correction with stent placement, balloon dilation, or cautery (Fig. 71.21) (49,50). Retransplantation has been attempted for this complication with variable success.
Infections
The rate of infection among lung transplant recipients is two to three times higher than that for recipients of other solid organs (51). This difference is most likely related to the exposure of the allograft to the external environment, and impaired local lung defenses—impaired mucociliary clearance, impaired lymphatic drainage, and poor cough reflex due to denervation. Infection is the most common cause of death in the first 12 months after lung transplantation and also a common cause of death after the first year. In a retrospective review at a lung transplant center, the rate of freedom from at least one episode of infection is 32% in patients surviving 12 months (Fig. 71.22). Bacterial infections are the most common with respiratory tract pathogens being the most common source of bacterial infections. Microbes from donor lungs cause a significant number of early episodes of bacterial pneumonia, but the incidence has decreased because antibacterial prophylaxis is used at the time of transplantation. A prospective, multicenter trial from Spain noted an incidence of 72 pneumonic episodes per 100 lung transplant recipients per year, with Pseudomonas aeruginosa being the most common, followed by Staphylococcus aureus (52). Viral infections are the second most common cause of infections; cytomegalovirus (CMV) infection is the most common among the viral etiologies. Patients at highest risk of infection and morbidity are CMV-seronegative recipients from a seropositive lung donor. The case fatality rate of CMV infection is reported to be about 5%. Extended CMV prophylaxis has resulted in significant decline of risk of CMV reactivation (53). Herpes simplex (HSV) and varicella zoster (VZ) infections are also quite common, and are mostly caused by reactivation of a latent viral infection. Acquired respiratory viral infections are mostly secondary to respiratory syncytial virus (RSV), influenza, and parainfluenza viruses. These viral infections may cause cough, wheezing or, in extreme cases, acute respiratory distress syndrome (ARDS). Parvovirus has been reported to cause refractory anemia in recipients of solid organ transplants. The most common cause of fungal infection is Candida albicans, likely due to central lines. Aspergillus also commonly infects the lung; the spectrum of disease ranges from necrotic bronchitis to invasive fungal pneumonia (54). The incidence of Pneumocystis jirovecii is probably 5% to 10% since the institution of long-term prophylaxis.

FIGURE 71.20 Bronchial mucosal reperfusion injury and Aspergillus infection of RUL orifice and bronchus intermedius before (A) and after debridement and right bronchus intermedius stent placement (B).

FIGURE 71.21 Bronchus intermedius showing pseudomembrane formation and severe bronchostenosis (at medial edge, A) prior to stent placement; and after 10- × 20-mm Ultraflex stent placement (B).

FIGURE 71.22 The 12-month freedom from any infection is 32% after lung transplantation in patients surviving 12 months (N = 110). (Data from University of Florida database.)
Acute Rejection
The lung is characterized by the highest rates of rejection among the commonly transplanted solid organs, with 20% to 25% freedom from acute rejection in the first year (55,56). Acute rejection denotes the infiltration of the allograft by lymphocytes (Fig. 71.23); severity of the rejection is classified by pathologic criteria (57). The diagnosis of acute cellular rejection (ACR) relies on the identification of lymphocytic perivascular or peribronchiolar infiltrates in lung tissue obtained from transbronchial biopsies. Many episodes of acute rejection are diagnosed in asymptomatic patients undergoing surveillance biopsies. When symptomatic, acute rejection can present with fever, hypoxemia, dyspnea, cough, or sputum production. Higher-grade rejection can lead to acute respiratory distress. Therapy of acute rejection is daily pulse methylprednisolone for 3 days. In steroid-resistant cases, antilymphocyte antibody therapy is given (thymoglobulin or alemtuzumab). The maintenance immunosuppression regimen is always reviewed and optimized to prevent recurrence.

FIGURE 71.23 Perivascular cuffing of a blood vessel is diagnostic of acute rejection. Transbronchial biopsy specimen.
Chronic Lung Allograft Dysfunction
Chronic lung allograft dysfunction (CLAD) is a term that has been used since about 2010 to describe the persistent decline in allograft function compared with the best FEV1 attained post transplant. Every effort should be made to identify the specific cause of decline in allograft function in the hope that appropriate and successful therapeutic interventions can be undertaken to restore and optimize graft function. However, patients who have persistent allograft dysfunction without an identifiable cause are labeled as having CLAD (Fig. 71.24) (58). Most insults to the allograft bronchial epithelium have been identified as potential risk factors for CLAD: severe PGD, recurrent ACR, antibody-mediated rejection (AMR), gastroesophageal (GE) reflux, and possibly respiratory viral infection (59–63). Approximately 66% of cases of CLAD are due to obstructive lung disease, synonymous with BOS; and 34% is due to restrictive lung disease, synonymous with restrictive allograft syndrome (RAS). BOS and RAS are two distinct phenotypes that are characterized by different chest radiographs and prognoses (64). Moreover, BOS is divided into distinct stages based on spirometry, with each lower stage carrying a poorer prognosis (65,66). In patients with decline in lung function, the CLAD diagnosis is made once reversible causes have been excluded. Bronchoscopy and transbronchial biopsy is performed to exclude the potentially reversible causes such as anastomotic complications, infections, acute rejection, or disease recurrence in the allograft. Unlike acute lung rejection, pathologic diagnosis of CLAD by transbronchial biopsy is difficult related to the small size of the lung sample obtained. Patients are also carefully assessed for GE reflux as treatment may stop the decline in spirometry.

FIGURE 71.24 Chronic lung allograft dysfunction could be of obstructive or restrictive physiology, with different prognosis in each category. FEV1, forced expiratory pressure in 1 second; FVC, forced vital capacity.

FIGURE 71.25 Open lung biopsy sample showing obliteration of a bronchiolar lumen with granulation tissue, diagnostic of obliterative bronchiolitis.
BO is the histologic equivalent of chronic lung rejection. BO is characterized by initial lymphocytic-mediated cytotoxicity directed at the epithelial cells lining the respiratory bronchioles. The lymphocytes initially infiltrate the submucosa of the airways and migrate through the basement membrane into the epithelial layer. At this site, epithelial cell necrosis occurs, resulting in a secondary cascade of inflammatory mediators and cytokines attracting neutrophils, fibroblasts, and myofibroblasts. This results in formation of granulation tissue obliterating the bronchiolar lumen, airway obstruction, and proximal bronchiectasis (Fig. 71.25). BOS occurs in approximately 50% of recipients 5 years after transplantation (see Fig. 71.11). It is the most common cause of death in patients surviving more than 1 year; the median survival after the diagnosis of BOS approximates 2 years. In most patients, BOS is characterized by a relentless decline in airflows (Table 71.7), albeit at variable rates in different patients, and ultimately results in respiratory failure. In BOS, the chest radiograph is initially clear, but in the advanced stages will show bronchiectasis and interstitial infiltrates. The chest radiograph in RAS shows interstitial infiltrates with restrictive spirometric changes. Retransplantation has been attempted for this complication but with variable success.
Cancers
Solid organ transplant recipients have elevated risk of cancers (67). Skin cancers are the most common form of malignancies, affecting more than half of solid organ transplant recipients during their long-term course. Several studies have shown that after a first cutaneous squamous cell carcinoma, the majority will develop subsequent skin cancers within 3 years. A decrease in cutaneous carcinogenesis after the reduction of immunosuppression has been reported. Moreover, sirolimus has been shown to reduce the rate of secondary recurrence of squamous cell carcinoma (68). Bronchogenic carcinomas have been reported in the native lung months-years after SLT. A transplant-related malignancy posttransplant lymphoproliferative disorder (PTLD), has also been reported in 1% to 5% of recipients in the first 5 years. The presentation and pattern of organ involvement is related to the time of onset, with intrathoracic predominance in the first year and extrathoracic predominance after the first year. The incidence of PTLD increases in proportion to the intensity of immunosuppression, especially when antilymphocyte antibodies are used. Most PTLD falls under the spectrum of non-Hodgkin lymphoma, and it is predominantly B-cell lymphocyte in lineage. Epstein–Barr virus (EBV) reactivation or infection is a major risk factor, with the majority showing EBV infection in lymphoma tissue. Decreasing the intensity of immunosuppression is the mainstay of therapy, but carries the risk of allograft rejection. Antiviral therapy, anti-CD20 monoclonal antibody therapy, combination chemotherapy, and radiation therapy have all been used, with variable success; an optimal treatment protocol has yet to be determined. Many patients die of the side effects of treatment rather than from disease progression (69).
TABLE 71.7 Staging of Bronchiolitis Obliterans Syndrome | |
![]() |
Recurrence of Native Lung Diseases
Sarcoidoses, giant cell pneumonitis, lymphangiomyomatosis, diffuse panbronchiolitis and, most recently, polymyositis-associated ILD have each been documented to recur in the allograft (70–74). Due to the small number of recipients with these diagnoses, and the relatively short follow-up time, the recurrence rate and its impact on the allograft function is not known with certainty. Additionally, the transplant community continues to transplant these diseases.
CONCLUSION
Lung transplantation has become an acceptable therapeutic option for patients with end-stage lung diseases who have failed all possible medical and surgical interventions. Patients should be referred for transplantation when projected 2-year survival is about 50% and transplantation is expected to increase the probability of survival. The patient’s perception of an unacceptable quality of life associated with the end-stage lung disease is an important additional consideration, but the life expectancy must be the overriding impetus for referral. In addition, since the waiting time may be up to 12 months, the patient’s health must remain stable during this time so that the patient will be able to endure transplantation, as well as the pulmonary rehabilitation that follows. The use of relatively stringent criteria is important in identifying candidates for whom transplantation is likely to be successful; it is important to avoid selecting poor candidates simply because of the desperate nature of their situation. Patients should be functionally disabled but ambulatory. They should also be free of clinically significant cardiac, renal, or hepatic impairment. Lung transplantation has been proven to prolong the 2- and 4-year survival in advanced stage IPF and CF patients, but not in patients with COPD. In COPD patients, we generally wait until the patient has a projected 2-year survival—with or without transplantation—before performing transplantation, with improvement in the quality of life being the most important factor to consider as the justification.
HEART TRANSPLANTATION
Heart failure affects 20 million people worldwide, 5.8 million are in the United States, where 550,000 cases are diagnosed yearly (75). With the exception of that caused by reversible etiologies, heart failure continues to progress over time, resulting in markedly decreased survival and quality of life (76). End-stage heart failure carries a dramatic 1-year mortality of 50% to 80% despite optimal medical therapy (77). Soon after its inception, orthotopic heart transplantation was shown to dramatically improve both survival and quality of life (78). However, despite the increasing number of patients in need of this life-changing therapy, the number of heart transplants has remained relatively steady in the last 20 years (79). Therefore, ISHLT has been diligent in developing registries and guidelines to optimize the application and management of this life-changing therapy (79–85).
OVERVIEW
As of June 30th, 2013, 104,027 adult heart transplants have been performed worldwide, and reported to the ISHLT; approximately 3,300 of these have occurred in last year (79). Advanced heart failure with reduced ejection fraction is the most common condition leading to cardiac transplantation; both nonischemic cardiomyopathy and ischemic cardiomyopathy account for 49% and 43% of all heart transplants, respectively. Other diagnoses leading to cardiac transplantation include valvular heart disease, congenital heart disease, retransplantation, and intractable ventricular arrhythmias. Multiorgan transplantation, generally resulting from end-stage heart failure leading to severe end-organ dysfunction, continues to progressively increase in the form of heart–kidney, heart–liver, and heart–lung transplants (79).
ORGAN ALLOCATION
The rate of heart donation has remained stable over the past decade; only 3.5 hearts per 1,000 patient deaths are recovered and transplanted in the United States (86). In order to ensure equitable distribution of organs, the UNOS, the entity responsible for the procurement and distribution of cadaveric organs in the United States, has developed an organ allocation system, the OPTN (Organ Procurement and Transplantation Network), based on blood type compatibility, geographical distance, severity of illness, and time accrued on the wait list. It operates through 11 geographic regions of the country, with each region further divided into many OPOs. The OPOs’ main responsibility is the identification and screening of potential donors, and coordination of the transplant process. In general, the allocation of hearts is first done within the OPO, and then outside it, in 500 nautical mile concentric zones from the donor hospital.
DONOR SELECTION
After consent for organ donation is obtained, the initial donor screening consists of confirmation of brain death, age, gender, height, weight, and ABO blood type. The OPO also investigates the circumstances leading to death, presence of active malignancies, time of severe hypotension and cardiopulmonary resuscitation, and other cardiovascular risk factors. Routine laboratory and serologic tests are performed (human immunodeficiency virus [HIV], hepatitis B [HBV], and C viruses [HCV], CMV, EBV, syphilis testing [RPR/VDRL], and human T-lymphotropic viruses [HTLV I/II]), as well as a succinct cardiovascular workup (electrocardiogram, chest x-ray, and a transthoracic echocardiogram; coronary angiography is usually done for donors older than 40 to 50 years old, and occasionally a right heart catheterization is justified). Class I and II HLA genotyping is also performed for prospective virtual cross-matching. Once the evaluation is complete, the donor is entered into the UNOS database and OPTN rank list for organ allocation. The vitality of the donor organs is maintained by the OPO, ensuring appropriate ventilation and organ perfusion; intravenous vasopressin, levothyroxine, and glucocorticoids are used to preserve the allograft function after brain death (87). The final evaluation is done in situ by the cardiovascular surgeon at the time of recovery.
Suitable donor characteristics include: Age less than 55 years, projected ischemic time less than 4 hours, left ventricular wall thickness less than 12 to 14 mm, LVEF more than 40% to 45%, no CAD (or single vessel CAD amenable to surgical revascularization), none or low-dose active inotropic agents, donor body weight no greater than 30% below that of the recipient, among others. Height matching is also of paramount importance; undersized hearts will be eventually insufficient to maintain the needs of the body, and oversized hearts will develop constrictive-like physiology and congestive heart failure symptoms (82).
The median donor age is currently 34 years, and it has been progressively rising in recent years; in the United States, only about 8% of donors are older than 50 years of age (79). Additionally, donors continue to be mostly males (about 69%), with an average BMI of 25. Comorbidities have become progressively more prevalent in the most recent era: 25% of donors have diabetes mellitus, 44% have hypertension, and 6% have had a previous malignancy (84).
RECIPIENT SELECTION
Due to the limited availability of donor hearts, and the increasing prevalence of patients in need of cardiac transplantation, the process of recipient selection and organ allocation continues to grow in importance. The expected survival of patients with end-stage heart failure is markedly reduced compared with healthy individuals of similar age; heart transplantation results in a remarkable improvement in survival and quality of life in appropriately selected patients (81).
The most common indications for cardiac transplantation are cardiogenic shock, end-stage heart failure despite optimal medical therapy, life-threatening refractory ventricular arrhythmias, and intractable angina (81). Ambulatory end-stage heart failure patients amenable to cardiac transplantation, are preferably evaluated with a cardiopulmonary exercise stress test (CPET) to assess their exercise performance; a peak VO2 of less than 12 mL/kg/min (while on optimal beta-blocker therapy), or less than 50% of predicted VO2, or a VE/VCO2 slope greater than 35, are all markers of high short-term mortality on standard medical therapy alone, and eligible candidates for cardiac transplantation. Additionally, combining the Heart Failure Survival Score (HFSS) (88) and the Seattle Heart Failure Model (SHFM) (89) improves the predictive ability for HF risk stratification in patients referred for transplantation (90).
TABLE 71.8 Evaluation Work-up Prior to Heart Transplantation: Anamnesis and Physical Examination |
![]() |

Full access? Get Clinical Tree
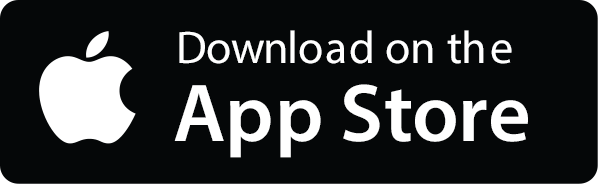
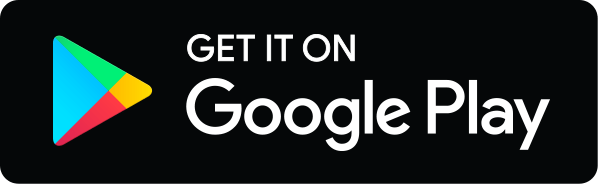