The Perioperative Period Is Critical for Postsurgical Cancer Outcomes
Surgery is the primary and often the most effective treatment for many solid tumors. However, even otherwise successful surgeries may disrupt and disseminate tumor cells. Disseminated tumor cells increase the risk of recurrence and metastasis. Indeed, the number of postsurgical circulating cancer cells is a negative prognostic indicator of disease-free survival.
A number of prometastatic events occur during the perioperative period as a direct consequence of surgery. For instance, direct injury to tissue causes local inflammation and a wound-healing response characterized by increased proliferation and enhanced release of angiogenic factors. These events promote the viability of residual or disseminated cancer cells. Inflammation also leads to edema, which increases the pressure of local drainage (via the lymphatic system) and enhances the propulsion of disseminated tumor cells away from the surgical site. Surgical trauma also induces a more general surgical stress response, characterized by systemic increases in catecholamines and inflammatory mediators. This can lead to immunosuppression and a reduction in the cytotoxic activity of natural killer (NK) cells. These events may tip the balance enabling micrometastases, previously suppressed by the immune system, to thrive. ,
Anesthetics and analgesics administered during the perioperative period have the potential to exacerbate prometastatic events, and there is considerable interest in the possibility that the type of anesthetic and/or analgesic agent may influence cancer recurrence. Indeed, a number of retrospective clinical studies suggest that regional anesthesia by local anesthetics increases disease-free survival following breast and prostate cancer surgery. Here, we explore the potential benefits of using local anesthetics during the perioperative period of surgical tumor excision with regard to subsequent disease progression and/or recurrence and the mechanisms, which may underpin it.
Potential Detrimental Effects of Anesthetics and Opioid Analgesics
Opioid analgesics are effective for the management of severe pain but can cause opioid-induced respiratory depression, constipation, hyperalgesia, tolerance, and dependence. Of particular concern in the context of the perioperative period is the possibility that some opioids may cause immunosuppression. For instance, fentanyl and morphine may activate mu-opioid receptors on NK cells, attenuating their cytotoxic activity. , Furthermore, fentanyl and morphine may also stimulate the hypothalamic-pituitary axis to raise glucocorticoid levels, causing indirect immunosuppression. The presence of mu-opioid receptors on some tumor cells (particularly non–small cell lung cancer) suggests that opioids may directly influence cancer cell function. The impact of opioids on cancer is discussed in detail in Chapter 12 .
Volatile general anesthetics such as sevoflurane may also suppress the immune response. In mice, sevoflurane treatment reduced total leukocyte and lymphocyte cell counts in blood. There is also less infiltration of NK and T-helper cells in breast cancer tissue biopsies from patients receiving sevoflurane. Furthermore, serum from breast cancer patients receiving sevoflurane reduces the cytotoxicity of NK cells in vitro. Volatile general anesthetics may also have direct procancer effects. For instance, serum taken from breast or colon cancer patients receiving sevoflurane impairs apoptosis of breast or colon cancer cells, respectively, in vitro. , Sevoflurane may also enhance migration and invasion in ovarian cancers and glioblastomas. The impact of volatile anesthetics on cancer is discussed further in Chapter 11 .
Indirect Benefits of Perioperative Local Anesthetics
Local anesthetics are often used to provide analgesia during the perioperative period as part of a balanced multimodal anesthesia. This diminishes the need for volatile anesthetics and opioid analgesics and may be beneficial for subsequent cancer outcomes. , Furthermore, local anesthetics may attenuate the stress response itself and mitigate the extent of perioperative immunosuppression. ,
However, a recent large prospective multicenter trial comparing outcomes after breast cancer surgery with inhalational anesthesia with or without paravertebral analgesia by ropivacaine or levobupivacaine revealed no difference in disease-free survival. The findings suggest that opioid and volatile anesthetic sparing effects of paravertebral analgesia do not account for any potential beneficial effects of local anesthetics on cancer outcomes in patients undergoing surgery for breast cancer. There are several other ongoing clinical trials exploring the impact of anesthetic technique on cancer outcomes; most predicated on the idea that the potential benefit of local anesthetics is conferred indirectly through their anesthetic sparing effects ( Table 10.1 ).
ClinicalTrials.gov ID | Study Start Date | Resection Site | LA Delivery | GA Alone Versus GA + LA | Direct LA Benefit |
---|---|---|---|---|---|
NCT00418457 | Jan-07 | Breast | NB | ✓ | |
NCT00938171 | Jun-08 | Breast | NB | ✓ | |
NCT01204242 | Aug-09 | Breast | IV | ✓ | |
NCT01916317 | Dec-11 | Breast | IT | ✓ | |
NCT02089178 | Feb-14 | Breast | IV | ✓ | |
NCT02786329 | Jun-16 | Colorectal | IV | ✓ | ✓ |
NCT02839668 | Aug-16 | Breast | IV | ✓ | |
NCT04065009 | Jan-20 | Ovary | IP | ✓ | |
However, there is mounting evidence that local anesthetics may have direct beneficial effects during the perioperative period. They may be antiinflammatory. This may be significant because there is a possibility that antiinflammatory drugs administered perioperatively increase disease-free survival after breast cancer excision. , Furthermore, some cancers express membrane protein targets of local anesthetics, including voltage-activated sodium ion channels (VASCs), not seen in their nonmalignant cellular counterparts. It is therefore possible that local anesthetics such as lidocaine, ropivacaine, and levobupivacaine might provide a direct beneficial effect by inhibiting the function of these proteins.
Direct Effects of Local Anesthetics on Cancer Cells
VASCs are the canonical target for local anesthetics. However, in vitro evidence also implicates the muscarinic acetylcholine receptor (mAChR) and Src kinase as targets for local anesthetic inhibition. , These proteins may play important roles in cancer cell oncophysiology, and their presence raises the possibility of additional beneficial effects of local anesthetics beyond general anesthetic and opioid analgesic sparing. Furthermore, emerging evidence suggests that lidocaine can stimulate the function of NK cells, inhibit tumor angiogenesis, as well as sensitize resistant cancer cells to the actions of several cytotoxic agents, such as cisplatin, pirarubicin, and 5-fluorouracil.
If this is the case, then a more beneficial approach for the use of local anesthetics might be to administer them directly into the tumor, or systemically (e.g., intravenously or intraperitoneally). Indeed lidocaine, a class 1b antiarrhythmic agent, can be safely administered intravenously and is also used as a circulating analgesic, and may be more beneficial in terms of pain control than epidural anesthesia. A number of ongoing clinical trials will test whether local anesthetics delivered directly onto breast tumors prior to excision, intravenously or intraperitoneally during the perioperative period for breast, colon, and ovarian cancer surgery, will prolong postoperative disease-free survival (NCT01916317, NCT02786329, and NCT04065009; Table 10.1 ).
Voltage-Activated Sodium Channel Oncochannelopathy
VASCs are ion channels most noted for their role in generating the action potential in excitable cells. Diseases involving VASCs in excitable cells are called Na + channelopathies. Notable examples are the congenital long QT3 (Romano-Ward) and Brugada syndromes involving Na V 1.5 (cardiac VASC), febrile seizures (Na V 1.1 and Na V 1.2), and painful inherited neuropathy (Na V 1.7). These classic channelopathies involve VASC dysfunction caused by mutations. In cancer, however, a number of hallmarks characterize oncogenic transformation. The term “oncochannelopathy” is used to describe the aberrant expression or function of a particular ion channel, leading to one or multiple cancer hallmarks. No underlying mutation is implied in oncochannelopathies, although mutations in VASC genes have been observed in breast and colon cancer cells.
VASC oncochannelopathies have been implicated in many different types of cancers. We focus on Na V 1.5, which is one of the most studied in metastatic tumors such as colon cancer , and breast cancer. Na V 1.5 is also found in prostate, lung (small and non–small cell), and ovarian cancers. Na V 1.5 expressed in breast and colon cancer cells is functional, and Na V 1.5-mediated ionic currents can be recorded using the patch-clamp technique from colon and breast cancer cells. , Metastatic SW620 colon cancer cells exhibit greater Na V 1.5-mediated currents compared to their SW480 adenocarcinoma cell counterparts. Inhibition of Na V 1.5 by tetrodotoxin or local anesthetics in colon and breast cancer cells reduces proliferation, migration, and invasive behavior, as does reduction in Na V 1.5 expression. , , , , Conversely, increasing Na + flux via Na V 1.5 in colon cancer cells using veratridine enhances invasive activity. , This highlights the importance of Na V 1.5 function in the signal transduction cascades necessary to initiate invasive behavior in cancer cells. It also raises the possibility of directly targeting Na V 1.5 using local anesthetics during the perioperative period and highlights the importance of the few clinical trials looking at the direct effects of local anesthetics ( Table 10.1 ).
Research into the role played by VASCs in oncophysiology is also beginning to unravel a unique and complex system of intracellular signaling and ionic homeostasis, which could be targeted by local anesthetics ( Fig. 10.1 ). Na V 1.5 activity in colon cancer cells can influence the expression of a number of invasion-related genes in a mitogen-activated protein kinase (MAPK)-dependent manner. This may be mediated via Na V 1.5 activity raising [Na + ] i . The Na + gradient is often utilized by cells to drive the transport of other molecules such as Ca 2+ , a key second messenger. It is possible that some of the changes in gene expression attributed to Na V 1.5 activity are mediated by altered [Ca 2+ ] i . Other targets of local anesthetics (such as mAChRs) may also exert their effects by regulating [Ca 2+ ] i . The presence of Na V 1.5 protein may also have direct effects on the function of other transporters, for example, by directly transactivating the sodium-proton exchanger (NHE1). , NHE1 mediates extracellular acidification which is required for the degradation of the extracellular matrix by matrix metalloproteinases. This puts Na V 1.5 in the center of a complex signaling pathway of cancer oncophysiology contributing to proliferation and invasion. It also highlights the potential of targeting Na V 1.5 function in cancer cells. In the following sections we will discuss recent advances in our understanding of the structure and function of Na V 1.5 VASCs, which provides new insights into the mechanism of their block by local anesthetics in the context of cancer.

Na V 1.5 Structure and Function
Na V 1.5 is encoded by the SCN5A gene located on chromosome 3p21. The α-subunit encoded by SCN5A is sufficient to form functional ion channels. Four auxiliary β-subunits are encoded by additional genes (SCN1B to SCN4B) and augment the function of some VASCs. VASC β-subunits may also play a role in breast cancers in which Na V 1.5 is found.
The pore-forming α-subunit of Na V 1.5 is a large protein (>200 kDa in size) with more than 2000 amino acids and contains binding sites for various drugs (including local anesthetics), β-subunits, calmodulin, and also modulatory sites for phosphorylation and ubiquitinylation. Bacterial and insect VASC structures were the first to be solved providing new insights into the relationship between their form and function. , More recently, the structures of mammalian Na V 1.5 and Na V 1.7 were solved. , The Na V 1.5 α-subunit is arranged in four homologous domains (DI–DIV) with six transmembrane segments (S1–S6) in each domain ( Fig. 10.2A ). Each domain is divided into a voltage-sensing module (VM) and a pore module (PM). Viewed from outside the cell membrane, the ion pore is surrounded by the four PMs. The VMs are arranged on the outer surface of the PMs ( Fig. 10.2B ). The inactivation gate is formed by the IFM motif in the short intracellular loop connecting DIII and DIV ( Fig. 10.2C ). Alternative mRNA splicing of SCN5A generates at least 13 Na V 1.5 variants. Of particular note is the neonatal Na V 1.5e splice variant, which is found in both breast and colon cancer cells. , The neonatal splice variant arises from alternative usage of exon 6, which codes for part of the VM.

The gating of Na V 1.5 and other VASCs involves three key steps: voltage-dependent activation, the rapid opening of an Na + selective ion channel pore, and voltage-dependent inactivation. Structurally, these involve transitions into discrete conformations: closed, active, and inactive. These are illustrated in Fig. 10.3 . Activation is caused by a voltage-dependent movement of S4 along its long axis and outward perpendicular to the membrane. , This movement opens the Na + selective pore lined by the PMs. Na + selectivity is governed by a conserved quartet of amino acids (DEKA) ( Fig. 10.2A ). VASCs, including Na V 1.5, inactivate within 1–2 ms following activation. This is caused by the inactivation gate (also sometimes referred to as the h-gate) containing the IFM motif folding into the intracellular opening of the pore blocking ion permeation. ,


Full access? Get Clinical Tree
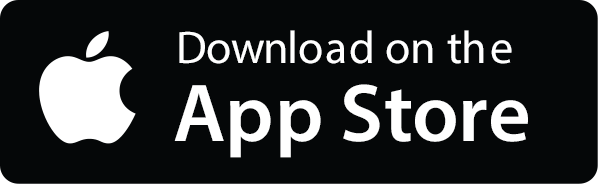
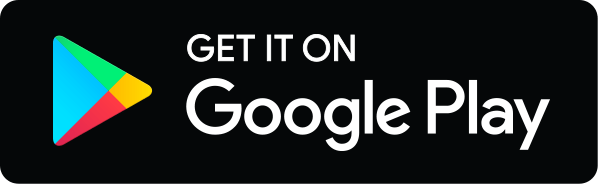
