Local anesthetics are synthetic derivatives of cocaine, a plant alkaloid obtained from the leaves of the South American coca plant, and the first local anesthetic to be discovered. Cocaine is a benzoic acid derivative coupled to a tertiary amine compound by an ester linkage. It is a weak base that is poorly soluble in water. Similarly, all local anesthetics contain a lipophilic benzoic acid derivative linked to a hydrophilic tertiary amine by an ester or amide chain and exist in ionized (cationic) and unionized forms (weak base). The lipophilic and hydrophilic components of local anesthetics enable their penetration into both lipid and aqueous membranes. It is this property that permits local anesthetics to traverse perineurium and axonal walls and block neural transmission without affecting cellular function or metabolism. Sodium conductance (i.e., depolarization) is prevented when an adequate concentration and volume of local anesthetic surround the nerve. The external opening of the Na+ channel is not the site of action of local anesthetics. Rather, the lipophilic, uncharged base form of the local anesthetic penetrates the neuronal cell wall and reaches the axoplasm where it exists in equilibrium as both a charged ionized salt and an uncharged base. The relative concentration of these forms depends on the tissue pH and the pKa of the compound. Once in the axoplasm, the charged base or cationic form enters the internal opening of the sodium channel and blocks Na+ conductance.
Local anesthetic metabolism is determined by the chemical linkage ( Fig. 34.1 ). Those with an ester linkage are metabolized by plasma esterases and those with an amide linkage are metabolized in the liver. Compounds with ester linkages are more likely to cause allergic reactions. The degree of lipid solubility of a local anesthetic determines its potency because the neuronal cell wall is a lipid structure. However, the relationship between lipid solubility and potency is not linear. The onset of action of a local anesthetic is correlated with its specific dissociation constant, or pKa, the pH at which 50% of the drug is present as the uncharged base and 50% as the cationic form. Only the unionized base can penetrate the cell wall. All local anesthetics have a pKa greater than 7.4. The lower the pKa of a local anesthetic, the greater the number of uncharged molecules available to traverse the lipid cell membrane, and the more rapid its onset of action.

The duration of action of a local anesthetic is determined by its protein binding capacity. After traversing lipid membranes, the local anesthetic enters the Na+ channel, a protein structure, to exert its pharmacologic effect. Therefore duration of action is directly correlated with degree of protein binding.
Frequency-dependent blockade is another important characteristic of local anesthetics. Most local anesthetics enter the Na+ channel only when it is open during depolarization. Thus neurons with high-frequency depolarization (i.e., sensory and pain fibers) are more readily blocked than fibers that have low-frequency depolarization (i.e., motor nerves).
Local anesthetic systemic toxicity (LAST) is the most feared complication of using regional anesthesia as it may result in side effects ranging from mild, transient symptoms to permanent disability or even death. Other reactions to local anesthetics include allergies and neurotoxicity. The best treatment of these problems is through prevention. However, this might not always be possible, so it is important to know the consensus statements published by the American Society of Regional Anesthesia and Pain Medicine.
Rare allergic reactions are mainly associated with ester local anesthetics, which are metabolized by plasma esterases to form para-aminobenzoic acid (PABA), the probable allergenic component. PABA is present in sunscreens and it is recommended that ester local anesthetics not be administered to patients with a history of sunscreen allergy. Skin testing is available to diagnose true local anesthetic allergy. The amide local anesthetics are safe in patients with a true allergy to ester anesthetics as cross-reactivity between ester and amide local anesthetics has not been demonstrated.
Every local anesthetic is capable of producing neurotoxicity. However, this complication is rare. True local anesthetic neurotoxicity is usually mild and resolves completely over time. More common is a neuropathy that follows a nerve injury because of needle trauma or a direct intraneural injection of epinephrine-containing local anesthetics in high concentrations. Lidocaine 5% for spinal anesthesia has been a particular concern because of its association with transient neurologic symptoms (TNS). This syndrome is characterized by the appearance of back pain that radiates to the buttocks within 24 hours of the block, the absence of motor and sensory deficits, and resolution within 3 to 10 days. The lowest effective concentration of local anesthetic should be used when performing any regional block.
Pharmacology of Local Anesthetics
Tetracaine
Tetracaine is an ester local anesthetic with a high pKa of 8.5, slow onset, and long duration of action (60–360 minutes). It is among the most potent local anesthetics in clinical use today. It is also among the most toxic. The maximum dose is 1 mg/kg. Tetracaine is most commonly used for spinal anesthesia (usual dose 0.2–0.6 mg/kg) and topical anesthesia of the eye. For spinal anesthesia in children, it comes in two forms: a lyophilized crystal that is reconstituted or a 1% solution that is diluted with distilled water, cerebrospinal fluid, or dextrose to produce hypobaric, isobaric, or a hyperbaric solution, respectively. The duration of action of tetracaine is prolonged by addition of epinephrine. It is also available as an ophthalmic solution as tetracaine hydrochloride 0.5%. The usual dose is 1 to 2 drops per eye. Long-term use of the drops is not recommended as damage to the surface of the eye may occur.
2-Chloroprocaine
2-Chloroprocaine is gaining popularity for pediatric epidural analgesia because it is rapidly metabolized by plasma esterases, its accumulation in the plasma is unlikely, and systemic toxicity is rare. Although neonates and infants have half the plasma esterase level of adults, there is no clinical difference in metabolism of 2-chloroprocaine. Chloroprocaine has a relatively high pKa of 9 and only about 5% of the drug is present in the unionized form at physiologic pH. Chloroprocaine has a rapid onset of action (5 to 10 minutes) because of its high tissue penetrance. It has a short duration of action (45 minutes) that can be prolonged (to 70–90 minutes) with addition of epinephrine. It has roughly a quarter of the potency of tetracaine or bupivacaine.
Use of epidural chloroprocaine decreased in the 1980s because of neurotoxicity associated with its 0.2% bisulfite preservative. Currently, chloroprocaine is available without preservatives for epidural use. Even with preservative-free chloroprocaine, a syndrome of back pain after large epidural doses has been described in adults. Epidural anesthesia is achieved by administering up to 1 mL/kg of 2-chloroprocaine (2% or 3%) with epinephrine 1:200,000 (maximal dose of chloroprocaine, 20–30 mg/kg). Continuous epidural analgesia has been used in infants aged less than 6 months using 1.5% chloroprocaine at 0.4 to 0.8 mL/kg/h.
Lidocaine
Lidocaine was the first amide local anesthetic introduced and remains a popular local anesthetic still in use today. Lidocaine has a relatively low pKa of 7.9 and 25% of the drug is nonionized at physiologic pH. It has a rapid onset and an intermediate duration of action (60–90 minutes) that is prolonged by adding epinephrine. It has one-eighth the potency of bupivacaine or tetracaine. The maximum dose of 5 mg/kg can be increased to 7 mg/kg by adding epinephrine. In addition to epidural and peripheral nerve blockade, lidocaine can be administered transcutaneously in the form of a skin patch (Lidoderm). It is also available as an oral formulation (mexiletine) for the treatment of neuropathic pain, and it is the only anesthetic recommended for IV regional blockade.
Bupivacaine
Bupivacaine has a relatively high pKa of 8.1 and is only 15% nonionized. Therefore the time to onset is relatively slow. Bupivacaine is highly protein bound and thus has a long duration of action, which is not prolonged by adding epinephrine. However, addition of epinephrine will reduce the rate of systemic absorption, and thus lower the peak plasma concentration, which is an important consideration given the potential toxicity of bupivacaine. Bupivacaine is one of the most potent local anesthetics in use today. Its l-enantiomer, levobupivacaine, may have less cardiac toxicity but it has equal anesthetic potency. The maximum dose of both bupivacaine and levobupivacaine is 3 mg/kg. Severe cardiovascular depression at plasma levels slightly above those considered necessary to produce signs and symptoms of neurotoxicity has been observed with bupivacaine. Bupivacaine has more depressant effects on the cardiac conduction system than lidocaine, but is no more toxic than lidocaine with regard to its effects on blood pressure and cardiac output. Bupivacaine can reduce conduction time and increase the potential for reentrant rhythms. Its duration is long because it is highly protein-bound and thus takes a long time to “wash out” from the sodium channel. Thus resuscitation can be prolonged but is not impossible.
Ropivacaine
Ropivacaine is the newest of the amide local anesthetics and was developed because of the cardiotoxicity associated with bupivacaine. It is chemically similar to bupivacaine, having a propyl side-chain whereas bupivacaine has a butyl side-chain. Its pKa is 8.0 and a slightly greater percentage of the drug is present in the nonionized form at physiologic pH. Ropivacaine is slightly less protein-bound than bupivacaine, so it is not surprising that it has a faster onset but slightly shorter duration of action (150–300 minutes). Like bupivacaine, the duration of blockade is not prolonged by adding epinephrine.
Ropivacaine is produced only as the l-enantiomer. One feature common to all the amide anesthetics is that their l-form possesses less cardiotoxicity. Ropivacaine appears to possess more sensorimotor discrimination than bupivacaine when administered to produce equivalent analgesia. It is estimated that ropivacaine is about three-quarters as potent as bupivacaine. It is available as 0.2% and 0.5% solutions and is dosed for epidural and peripheral nerve block with a maximum dose of 4 mg/kg for single injections and 0.4 mg/kg/h for continuous infusions.
Pharmacology of Adjuvant Analgesics
Clonidine
Clonidine is an α-2-adrenergic agonist that was developed primarily as a nasal decongestant because of its peripheral vasoconstrictor properties. It was soon recognized to have hypotensive effects and was primarily then used as an antihypertensive agent in adults. More recently, its anxiolytic and antinociceptive effects have been useful in the perioperative setting. For sedation and anxiolysis, clonidine, 2 to 4 μg/kg, can be administered orally. It possesses the ability to reduce intraoperative anesthetic requirements, reduce postoperative opioid consumption, and stabilize hemodynamic responses to laryngoscopy and surgery.
Clonidine is used as an analgesic adjuvant in the management of acute and chronic pain in children. In peripheral and epidural nerve blocks, clonidine prolongs both sensory and motor blockade of the local anesthetic. Clonidine alone can produce analgesia when injected in the epidural or intrathecal space, or in the proximity of peripheral nerves. However, because of the large doses required, side effects of hypotension, bradycardia, and sedation limit its utility as a sole analgesic agent. Clonidine has proven useful to control withdrawal symptoms in children with opioid or benzodiazepine dependence.
The mechanism by which clonidine exerts its analgesic effects are uncertain. In the superficial laminae of the dorsal horn of the spinal cord and in certain brain stem nuclei, α-2-receptors are abundant. Central sympatholysis is believed to be responsible for its antihypertensive effect. The prevention of norepinephrine release from presynaptic nerve terminals by clonidine is believed to be partially responsible for producing analgesia. However, there is evidence that clonidine increases acetylcholine release from neurons in the dorsal horn of the spinal cord, thus activating spinal acetylcholine receptors. This may enhance local anesthetic blockade of C and Aδ fibers by augmenting potassium conductance.
Clonidine can be administered by a variety of routes. As an epidural additive, the bolus dose is 1 μg/kg, which may be followed by an infusion of 0.05 to 0.2 μg/kg/h. When given orally, it is almost totally absorbed from the GI tract. Once in the systemic circulation, its high lipid solubility allows it to penetrate the spinal cord and brain. Clonidine comes in an injectable form and in a tablet (0.1, 0.2, and 0.3 mg) and transdermal patch (0.1, 0.2, and 0.3 mg). The patches need to be changed every 7 days. The patches should not be cut, and an alternative delivery method should be employed. The oral starting dose is 2 to 4 μg/kg/day and transdermal dose of clonidine is 4 to 8 μg/kg/day, both with a maximum of 0.3 mg/day. The oral dose is usually administered in two divided doses. Clonidine cannot be abruptly discontinued after prolonged administration, because severe hypertension and other symptoms similar to an abstinence syndrome may occur.
Anticonvulsants
Anticonvulsants are useful for managing a variety of neuropathic pain conditions including complex regional pain syndrome, phantom limb pain, and postherpetic neuralgia. However, few studies in children exist and the use of this class of drugs in children is based on extrapolation from adult literature and from growing anecdotal pediatric experience. Although early adult experience established the efficacy of phenytoin, carbamazepine, and valproic acid as analgesic adjuvants, gabapentin is now the first-line anticonvulsant used for this purpose.
Gabapentin
Gabapentin was developed as a gamma aminobutyric acid (GABA) analog for relief of muscle spasticity but was later recognized to be more effective as an anticonvulsant. The mechanism of action of gabapentin has not been clearly delineated. It does not appear to interact with receptors or sodium channels, and may enhance extracellular GABA concentrations by altering GABA transport. Gabapentin is not metabolized and is entirely dependent on renal excretion for elimination. It is not protein-bound and has a half-life of 5 to 9 hours. The most common adverse effects include abdominal pain, dizziness and ataxia, tremor, nystagmus, somnolence, and mood and behavior disturbances. However, gabapentin is generally well tolerated and the side effects can be largely avoided by slowly increasing the dose of gabapentin on a daily basis until analgesia results or intolerable side effects disappear. In the author’s institution we start with 5 mg/kg (maximum dose 300 mg) at bedtime on the first day, 5 mg/kg twice-daily on the second day, and then 5 mg/kg thrice-daily. We continue to increase the daily dose in this fashion by 5 mg/kg/day. If somnolence is bothersome, we administer half the daily dose at bedtime. The maximum daily dose is 80 mg/kg/day, or the adult dose of 3600 mg.
Carbamazepine
Carbamazepine is an anticonvulsant that is believed to produce analgesia by sodium-channel blockade. Carbamazepine is metabolized by the liver. Therapy is initiated in children aged over 6 years with an oral dose of 10 mg/kg/day divided in two to four doses and increased to the usual maintenance dose of 15 to 30 mg/kg/day. Severe adverse effects including aplastic anemia, agranulocytosis, congestive heart failure, sedation, fatigue, ataxia, slurred speech, and hepatitis have been reported. A complete blood count and liver function studies should be obtained before and during therapy.
Valproic Acid
Valproic acid is an adjuvant treatment for neuropathic pain accompanied by a mood disturbance, and prophylactic therapy for migraine headaches. At least three mechanisms of action have been proposed for valproic acid: (1) increased production and release of GABA in central neurons; (2) reduced neuronal excitation initiated by N-methyl-D-aspartate (NMDA)-type glutamate receptors; and (3) direct membrane stabilizing effects. The usual oral starting dose of 10 to 15 mg/kg/day may be increased to 60 mg/kg/day in two to three divided doses. Anorexia, nausea and vomiting, weight gain or loss, and sedation are common side effects. The most serious side effects include hepatotoxicity, hyperammonemia, platelet dysfunction, and pancreatitis.
Tricyclic Antidepressants
When added to an analgesic regimen, tricyclic antidepressants (TCAs) improve sleep, mood, and daily functioning. Studies of its use for chronic pain in children have not been performed. These drugs are believed to produce analgesia by central serotonin and norepinephrine reuptake inhibition, thus augmenting descending inhibitory pathways. This class of drugs causes anticholinergic side effects: dry mouth, sedation, orthostatic hypotension, constipation, urinary retention, and tachycardia. Sudden death from arrhythmias has been reported in children on tricyclic antidepressants for treatment of depression. Before initiating TCA therapy, we obtain a history for palpitations, syncope, and cardiac conduction disturbances. An electrocardiogram should be obtained to rule out prolonged QT interval. Nortriptyline may produce less daytime somnolence and fewer anticholinergic effects than amitriptyline. However, when insomnia accompanies chronic neuropathic pain, we prefer to use amitriptyline. Therapy with amitriptyline or nortriptyline is initiated at 0.1 to 0.2 mg/kg at bedtime and increased by 0.1 to 0.2 mg/kg every 3 to 4 days to a maximum of 1 mg/kg/day or 50 mg.
Local Anesthetics
Lidocaine and its oral formulation (mexiletine) are referred to as membrane stabilizers because they block sodium channels, prevent membrane depolarization, and block excitatory impulses along neural pathways. They are used to treat neuropathic pain in adults and have been used in children with mixed results. To determine its usefulness, we administer an IV bolus of 1 mg/kg followed by an infusion at 1 mg/kg/h, with gradual increases to achieve a plasma lidocaine concentration of 2 to 5 μg/mL. If this test regimen produces adequate analgesia, oral therapy with mexiletine is begun. Adverse side effects of mexiletine include nausea and vomiting, ataxia, diplopia, and sedation; these frequently limit its use.
References

Full access? Get Clinical Tree
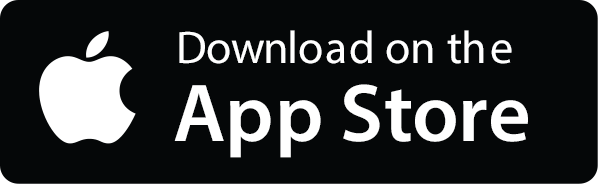
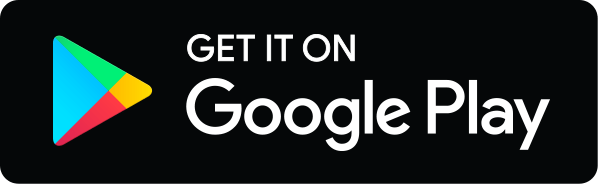
