• Andrea Casati, MD
I. | INTRODUCTION |
II. | NERVE ROOTS ANATOMY & CLASSIFICATION OF NERVE FIBERS |
III. | CLINICAL PROPERTIES OF LOCAL ANESTHETIC SOLUTIONS |
IV. | GENERAL PRINCIPLES |
V. | SELECTING A LOCAL ANESTHETIC FOR SURGICAL ANESTHESIA |
VI. | POSTOPERATIVE MAINTENANCE OF NERVE BLOCK |
VII. | USING ADDITIVES FOR CONTINUOUS PERIPHERAL NERVE BLOCKS Vasoconstrictors Alkalinization Clonidine Peripheral Opioids |
VIII. | COMPLICATIONS RELATED TO THE INJECTION & INFUSION OF LOCAL ANESTHETIC SOLUTIONS |
INTRODUCTION
In the past few years progress has been made in understanding the mechanisms and pathways involved in the modulation of pain, as well as in developing new therapeutic tools to provide satisfactory pain relief after surgery. The relationship between the intensity of acute postoperative pain and the duration of the patient’s recovery and functional outcome has been well established. For these reasons, the prevention and treatment of acute pain had become the focus of great interest for perioperative specialists. Postoperative pain differs from chronic pain by its shorter duration and its requirement for immediate relief, which dictate the development of suitable management protocols. Preemptive and preventive analgesia also represent concepts that only apply to acute postoperative pain. Finally, it is important to recognize the role of acute pain in the development of chronic pain syndrome.
Irrespective of its nature, pain is not an objective but rather a subjective symptom. In the surgical as well as medical environment, intrinsic and extrinsic factors affect individual pain thresholds. Accordingly, the clinician must be always aware that pain treatment must be approached using a multimodal and multipharmacologic approach; no one single technique by itself, including the use of continuous peripheral nerve block, provides adequate pain relief in all patients and in all circumstances. The first description of continuous peripheral nerve block was reported in 1946 by Paul Ansbro,1 who described the placement at the supraclavicular level of a blunt needle secured to the patient’s skin using a cork, through which the needle was inserted before block placement. This cumbersome apparatus allowed the incremental injection of local anesthetic in order to prolong the duration of anesthesia in patients undergoing upper extremity surgery. In their report the authors used a short-onset/intermediateduration local anesthetic, like 1% procaine. After an initial 40-mL bolus the authors injected incremental doses based on the duration of surgery, up to a final volume ranging between 120 mL for 1.5-h surgery and 220 mL for 4-h surgery. During the following 3 decades continuous perineural infusion techniques continued to be developed, and their indications extended; initially they were mainly used for upper extremity blocks, afterward they were also employed for lower limb blocks.
In 1977 Selander2 reported on the injection of 30 to 50 mL of mepivacaine to conduct a continuous axillary block in 137 patients undergoing hand surgery, and in 1979 Manriquez and Pallares3 reported on the repeated injection of 20 mL of 0.25% bupivacaine every 6 h to prolong the sympathetic block and pain control for 4 days.
In 1982 Matsuda and colleagues4 reported on the use of either 30 mL of 1% lidocaine with epinephrine followed by 15 mL intermittently (1.5-2.75 h) or 40 mL of a 0.5% bupivacaine and 1% lidocaine mixture followed by intermittent injection of 20 mL (1.25-4.3 h) in 50 patients undergoing upper extremity reimplantation. Subsequently most of the groups have focused their clinical protocols on the use of low concentrations (0.125-0.25%) of bupivacaine.5–7
More recently, with the introduction of new long-acting aminoamide local anesthetics, such as ropivacaine or levobupivacaine, the attention of clinicians has shifted to the evaluation of these new agents, often with interesting findings. Borgeat and coworkers8 compared 0.2% ropivacaine and 0.15% bupivacaine (to account for the difference in potency between the two agents) in terms of their effects on motor function and demonstrated that they provided similar postoperative pain control, but 0.2% ropivacaine allowed for better preservation of motor function than bupivacaine. On the other hand, levobupivacaine at 0.125% concentration seems to provide the same level of motor preservation as 0.2% ropivacaine.9
The advantages of continuous block techniques have been largely demonstrated for major orthopedic surgery, implementing the rehabilitation of these patients after total arthroplasty allowing early, pain-free mobilization of the operated limb. Finally, the indication of this technique of pain control has also been expanded to outpatient procedures. In fact it is known that over 40% of ambulatory patients experience moderate to severe pain within the first 48 h after ambulatory orthopedic surgery. Although different therapeutic approaches have been advocated, none provide reliable pain control, and pain remains a major concern. Most frequently patients are discharged with oral medication that has limited effects on pain relief. Intraarticular injections of narcotic analgesics or local anesthetics have also been used, but these techniques have been shown to provide only limited and short-lasting pain relief. In recent years several groups have evaluated the use of continuous nerve blocks in outpatients. Most of these investigators used solutions of 0.125% bupivacaine or 0.2% ropivacaine infused through either elastomeric or electronic patient-controlled (PCA) pumps, with relevant benefits not only in terms of quality of postoperative analgesia, but also in improved quality of life during the first postoperative days.10–12
NERVE ROOTS ANATOMY & CLASSIFICATION OF NERVE FIBERS
The nerve roots are divided into three types according to their anatomic and functional properties: A, B, and C fibers. The A fibers are responsible for motor efferent conduction and are divided in Aα, Aβ, and Aγ fibers. They are all myelinated, like the Aδ fibers, which are sensory fibers carrying pressure and distension information. The B fibers are constituted of the autonomic pregangliar fibers, and the C fibers include all the amyelinic fibers of the posterior spinal roots as well as the postgangliar autonomic fibers.
Clinical Pearls
Generally, the bigger the size of the nerve fibers the greater the amount of local anesthetic solution required to block conduction. Thus, fibers of small size are blocked sooner than those of larger diameter.
The B fibers of the autonomic system constitute an exception of this rule: even though they are myelinated fibers a minimum concentration of local anesthetic solution is required to produce an effective blockade.
This explains why the sympathetic blockade is observed before the onset of sensory or motor blockade.
In the myelinated nerve roots, the action potential conduction proceeds from one Ranvier node to the next (jumping, or saltatory, conduction). Because the size of the fibers is proportional to the length between one Ranvier node and the next, the speed of conduction of the action potential increases with the size of the fibers. Generally, the bigger the size of the nerve fibers, the greater the amount of local anesthetic solution required to block the conduction. Thus, the fibers of small size are blocked sooner than those of larger diameter. The B fibers of the autonomic system constitute an exception to this rule: even though they are myelinated fibers, a minimum concentration of local anesthetic solution is required to produce an effective blockade. This property of B fibers explains why the sympathetic blockade is observed before the block of the other fibers (Table 10-1). The difference in sensitivity to neural blockade allows determination of the minimum concentration of local anesthetic that blocks only the small fibers, mainly responsible for nociception, with minimum or no block of the lager fibers. This in turn, allows neural blockade to accomplish analgesia with no or minimum motor block; the basis of the differential sensory-motor blockade.
Onset and Recovery of Nerve Blockade in the Different Types of Nerve Fibers
Nerve Block Onset | Nerve Block Recovery |
| |
B | A-α |
| |
C; A-δ | A-β |
| |
A-γ | A-γ |
| |
A-β | C; A-δ |
| |
A-α | B |
CLINICAL PROPERTIES OF LOCAL ANESTHETIC SOLUTIONS
The choice for a local anesthetic solution for peripheral nerve blocks is based on
1. Onset time
2. Duration of blockade
3. Ability to produce a differential sensory-motor block
4. Potential for toxicity
The onset time of local anesthetics is influenced by the molecule’s pKa (the higher the pKa the slower the onset time of the nerve block in a physiologic environment) and diffusibility.13 On the other hand, the ability to cross the cell membrane depends on the molecular weight and the liposolubility of the molecule. All local anesthetics have nearly the same molecular weight, but the diffusibility of the local anesthetic molecules from the injection site depends on its hydrophilicity. The nonionized form of the molecule is more lipid-soluble than the ionized one, so it and can cross the cell membrane easier but diffuses less easily. The commercial solutions of local anesthetics have an acid pH, and the pKa of the different local anesthetics range from 7.9 to 8.1. Accordingly, the ionized form, which is less lipophilic, is more represented than the nonionized one.
Clinical Pearls
The onset time of local anesthetics is influenced by the molecule’s pKa (the higher the pKa, the slower the onset time of the nerve block in a physiologic environment) and diffusibility.
The ability to cross the cell membrane depends on the molecular weight and the liposolubility of the molecule.
All local anesthetics have nearly the same molecular weight, but the diffusibility of the local anesthetic molecules from the injection site depends on its hydrophilicity.
The nonionized form of the molecule is more lipidsoluble than the ionized one, therefore, it can more readily cross the cell membrane but diffuses less easily.
As onset time decreases, the dose, volume, or concentration of a given local anesthetic can be increased. Other strategies include the modification of the pKa of the anesthetic solution by warming it or adding sodium bicarbonate to increase the number of nonionized local anesthetic molecules, especially with lidocaine and mepivacaine. The alkalinization of ropivacaine, bupivacaine, or levobupivacaine is much more difficult, because of their very high pKa. Another important aspect deserving consideration is the pH of tissues; for example, the local tissue acidosis related to inflammation can increase the ionized form of local anesthetic, thereby reducing its efficacy. The potency of a local anesthetic is usually expressed as the minimum effective concentration (Cm): the minimum anesthetic concentration that reduces the action potential of a nerve fiber bathed in a solution with a 7.27.4 pH and stimulated with a 30-Hz current by 50% within 5 min. The potency of local anesthetics is strictly related to their lipid-solubility; the more lipid-soluble a local anesthetic is, the greater its potency and consequently the lower its Cm.
The Cm of a local anesthetic also changes according to the size of the nerve fiber. Although the total amount of local anesthetic affects the onset, degree, and duration of the nerve block, its concentration primarily influences the intensity of the blockade. The smallest fibers (A-δ, β, and C), with a slower conduction speed, are more sensitive to the blocking activity of the local anesthetic solution than are those with a larger diameter (A-β, and A-α) and fast conduction. In other words, the smallest fibers need a lower Cm than those of larger size. This aspect is related to the number of anesthetic molecules available to block the conduction; when using low concentrations the small number of local anesthetic molecules available will block only the small fibers. A possible explanation is the need for blocking three consecutive Ranvier nodes in order to produce a complete nerve block. Since the distance between consecutive Ranvier nodes increases as the size of the nerve fiber increases, low concentrations of local anesthetics block three consecutive nodes only in the small nerve fibers and not in the large ones. This is the basis for the differential sensorymotor blockade, which is more evident with the lipophilic agents with high pKa, such as bupivacaine, ropivacaine, and levobupivacaine.
Table 10–2.
Chemical and Physical Properties of the Main Local Anesthetic Drugs, Including the Reported Equipotent Concentrations

Full access? Get Clinical Tree
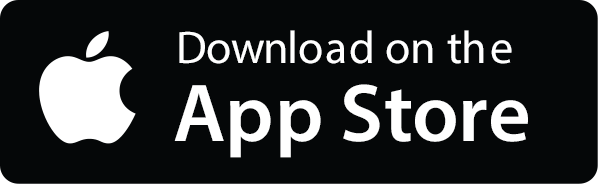
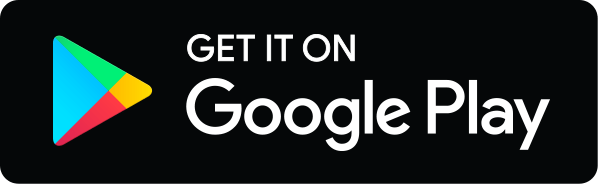