In his seminal 1876 work defining germ theory, Dr. Robert Koch described experiments in which he established the causative role of infecting microorganisms in septic shock. Infection was accepted as the cause of sepsis until 1957, when bacterial endotoxin was discovered and demonstrated to recapitulate the pathophysiology of septic shock in the absence of a live replicating bacterial infection. The link between septic shock and the immune system was established 30 years later when Cerami and colleagues demonstrated that endotoxin activated macrophages to release the inflammatory cytokine tumor necrosis factor α (TNF-α), later found to be a primary endogenous mediator of endotoxic shock. Subsequent studies established elevated levels of inflammatory cytokines in the blood of patients with sepsis. The prevailing theory regarding the pathogenesis of sepsis at that time was that bacterial infection induced an inflammatory cytokine storm that caused septic shock. Subsequently, sepsis researchers sought to treat sepsis by attenuating the inflammatory response. Unfortunately, in numerous clinical trials, anti-inflammatory strategies have failed to improve sepsis survival, and in some cases treatment with anti-inflammatory agents increased sepsis mortality.
The failure of anti-inflammatory strategies alone to improve sepsis survival led to a reevaluation of the role of the immune system in the pathogenesis of sepsis. Mounting evidence suggests that suppression is the primary immune derangement in the septic patient and that the pathophysiology of sepsis results as much or more from an immunocompromised state than from a systemic inflammatory response.
A wealth of evidence supports a role for immune dysfunction in sepsis, with data demonstrating exaggerated and suppressed immune responses. The state of the immune system in sepsis, though, remains incompletely understood. This chapter reviews the available basic and clinical evidence indicating that immunosuppression is the overriding immunologic derangement septic patients. We also highlight potential methods to monitor the immune status of a critically ill patient. We review potential therapies aimed at stimulating the immune system of the septic patient.
Immune System in Sepsis: Systemic Inflammatory Response Syndrome to Compensatory Anti-inflammatory Response Syndrome
The host response to infection is complex and varies depending on the type of infection, bacterial load, and host genetic factors. Cells of the innate immune system, including granulocytes, macrophages, dendritic cells, and innate lymphoid cells such as natural killer cells express germ-line-encoded receptors that recognize pathogen-associated molecular patterns and host-derived molecules associated with tissue damage (damage-associated molecular patterns). These innate immune cells are tasked with the early recognition of microbial infection and the tissue damage associated with infection or injury. Activation of the innate immune system causes release of a diverse range of inflammatory mediators including those canonically associated with sepsis: TNF-α and interleukin 6 (IL-6). The proximal signaling mechanisms of the innate immune system form a positive feedback loop that serves to amplify the response to minor injuries and infections to facilitate early clearance of infecting organisms and damaged tissue. This innate inflammatory response activates the adaptive immune system, inducing proliferation of antigen-specific T cells and B cells that acutely combat infection and provide long-term memory cells, improving future immunity. Under normal physiologic conditions, progression of the immune response leads to activation of negative feedback pathways that downregulate inflammation. Innate immune cells switch from production of inflammatory cytokines to production of anti-inflammatory cytokines such as IL-10 and transforming growth factor β (TGF-β). Innate immune cells, including antigen-presenting cells, undergo programmed cell death through apoptosis, which removes the stimulation to the adaptive immune cells. Without activation, T and B cells become less responsive. A subset of these cells transition to a memory phenotype, and effector B and T cells, no longer required to fight infection, undergo apoptosis.
With the discovery that TNF-α can induce a syndrome similar to endotoxic shock, a theory of sepsis as an overexuberant inflammatory reaction developed. It was hypothesized that in response to severe infections or significant tissue damage, the positive feedback loops of the innate immune response drove activation of the inflammatory response beyond the local environment. This change led to a pathologic organism-wide activation of innate immunity, termed the systemic inflammatory response syndrome (SIRS). Inflammatory cytokines that normally function in the local environment were systemically disseminated, causing fever, leukocytosis, and tachycardia while contributing to capillary leak, vasogenic shock, and remote organ damage. To explain the persistence of SIRS in sepsis, Bone hypothesized that, under normal conditions, inflammation was followed by immune downregulation that limited the inflammatory response. Just as SIRS represented exaggeration of normal, adaptive, proinflammatory pathways, a new syndrome, the compensatory anti-inflammatory response syndrome (CARS), reflected a pathologic downregulation of immune function.
Since the development of the SIRS-CARS model, ongoing research has clarified the relationship among the severe infections that cause sepsis, the state of the immune system, and the clinical course of septic patients. Clinically, septic patients exhibit clear evidence of immune dysfunction. The signs include a loss of delayed hypersensitivity, an inability to clear microorganisms, and a predisposition to secondary infections. Postmortem studies demonstrate that 80% of patients who die with sepsis have a persistent focus of infection. With ongoing sepsis, infectious burden increases, with increased frequency of positive blood cultures and increased infections with opportunistic organisms. Septic patients also demonstrate viral DNAemia; viral DNA from latent herpes family viruses (presumably reactivated during sepsis-induced immunosuppression) are recovered in the blood of 42% of sepsis patients, as compared with 5% of critically ill nonseptic controls. The immune state of the septic patient is characterized by impairment of neutrophil functions, increased lymphocyte and dendritic cell apoptosis, a shift from a T H 1 (T helper cell type 1) to a T H 2 (T helper cell type 2) cytokine profile, an increase in the proportion of T regulatory cells, a release of anti-inflammatory mediators, lymphocyte anergy, and monocyte deactivation ( Table 38-1 ). Most deaths in sepsis occur late in the course of the syndrome, well after resuscitation. Those patients who survive show evidence of immune function recovery.
Lymphocyte (CD4 T cells, B cells) and dendritic cell apoptosis |
Switch to T H 2, or immunosuppressive, cytokine profile and release of anti-inflammatory mediators |
Lymphocyte anergy increased proportion of regulatory T cells |
Monocyte deactivation evidenced by decreased expression of mHLA-DR Impairment of neutrophil functions |
Expansion of immature myeloid suppressor cell populations |
Mechanisms of Immune Dysfunction
In animal and human studies, sepsis induces apoptosis in lymphocytes and gastrointestinal epithelial cells ( Fig. 38-1 ). Examination of the spleen of patients who died from sepsis reveals a profound depletion of B cells, CD4 T cells, and follicular dendritic cells from the innate and adaptive immune systems that is not observed in the spleen of patients who died after trauma. Septic patients also have absolute lymphocyte counts well below normal. This lymphopenia is associated with poor outcome and the degree of lymphocyte apoptosis correlates with the severity of sepsis. Loss of these cells impairs antibody production, macrophage activation, and antigen presentation. Apoptosis also impairs innate immunity by disrupting the crosstalk between the innate and adaptive immune systems. As a result, sepsis is associated with T cell anergy. Macrophages and dendritic cells that take up and eliminate apoptotic cells release anti-inflammatory cytokines such as IL-10 and TGF-β while suppressing proinflammatory cytokines. T cells that come into contact with these macrophages and dendritic cells become anergic or undergo apoptosis. T cells of patients with peritonitis also have decreased T H 1 function even in the absence of T H 2 cytokines. These T cells fail to proliferate. These T cell findings positively correlate with mortality. Studies in a clinically relevant mouse model of sepsis confirm the significance of lymphocyte apoptosis to sepsis pathophysiology. Mice were injected with either apoptotic or necrotic cells before induction of sepsis and survival was recorded. Mice adopting transferred apoptotic cells had greater mortality compared with mice that received necrotic cells. Significantly, mice that received apoptotic as opposed to necrotic cells also exhibited T H 2 cytokine profiles and decreased interferon γ (IFN-γ) production by spleen cells. In several animal studies, apoptosis was reversed. In such investigations, mice that overexpressed BCL-2, an antiapoptotic protein, in lymphocytes had lower mortality rates in pneumonia and cecal ligation and puncture models of sepsis. Similar data were reported in mice that overexpressed BCL-2 (B cell lymphoma 2) in gut epithelia. The cellular mechanisms of apoptosis in sepsis are incompletely understood, but there is evidence that the extrinsic death receptor and the intrinsic, or mitochondrial, pathways are being activated. Death receptors activated by circulating TNF and CD95 (FasL) activate caspase-8, which then sets off an apoptotic cascade. The mitochondrial pathway can be stimulated by several different agents. These include reactive oxygen species, radiation, chemotherapeutic agents, cytochrome c , and cytokine withdrawal. It appears that there is significant crosstalk between the two pathways and that sepsis acts through multiple mechanisms to induce cell apoptosis.

Although controversial, some investigators have reported that T regulatory (CD4 + and CD25 + ) cells play an important role in the immunosuppression that occurs during sepsis. T regulatory cells modulate the immune response to pathogens by acting on other T cells and antigen-presenting cells. T regulatory cells release cytokines such as IL-10, TGF-β, and IL-4 and thereby mediate responses in CD4 and CD8 T cells. One study revealed that the proportion of T regulatory cells was increased in the blood of septic patients immediately after diagnosis and persisted only in nonsurvivors. This increase in T regulatory cells also has been shown in clinically relevant animal models of sepsis. T regulatory cells may be important in the switch from a hyperinflammatory state to immune dysfunction in sepsis. A study demonstrated improved survival in septic mice given an antibody to the glucocorticoid-induced TNF receptor that is highly expressed on T regulatory cells. This antibody restored CD4 + T cell proliferation and increased T H 1 and T H 2 cytokines. This approach reversed the adaptive immune dysfunction seen in sepsis. T regulatory cells may prove to play a crucial role in the development and treatment of immune dysfunction in severe sepsis. Our group performed functional studies on leukocytes isolated from immediate postmortem autopsies of critically ill patients. As compared with noncritically ill control patients, splenocytes from septic patients were profoundly anergic. When stimulated ex vivo, cytokine elaborated by cells from septic patients was generally less than 10% of that produced by cells isolated from noncritically ill controls. These differences included proinflammatory and anti-inflammatory cytokines, demonstrating that these lymphocytes are globally suppressed and not simply skewed to a pro- or anti-inflammatory phenotype. This phenotype has been hypothesized to result from either T cell exhaustion or from a global slow-down in cellular function and is termed hibernation. Phenotypic evaluation of cells from septic patients found increased expression of the inhibitory receptor programmed cell death protein-1 (PD-1), suggesting that sepsis results in a lymphocyte cohort that suppresses cellular activation. Administration of antibodies designed to block PD-1 activity restored adaptive immune function, suggesting that PD-1 inhibited lymphocyte activation. These data are consistent with a T cell “exhaustion” phenotype and provide a possible means to reverse T cell dysfunction in patients using anti–PD-1 antibodies.
In addition to the demonstrated effects of sepsis on splenocytes, recent studies have found expansion of immature myeloid suppressor cells (Gr-1 + , CD11b + cells) in the spleen, lymph nodes, and bone marrow in prolonged sepsis. Studies of lung tissue from septic patients demonstrated increased numbers of myeloid-derived suppressor cells (MDSCs) in the lung as compared with healthy controls. Depletion of these cells in septic mice challenged with T-cell-dependent antigens blocked a T H 2 response. This evidence suggests a role for immature myeloid suppressor cells in sepsis-induced immune suppression.
Monocytes from septic patients also are dramatically affected. In patients with postoperative sepsis, there is an immediate suppression of proinflammatory and anti-inflammatory cytokines after lipopolysaccharide stimulation. Survival among these patients correlated with a recovery of the proinflammatory, but not the anti-inflammatory, response. Monocytes from septic patients have decreased cell surface markers, notably monocyte human leukocyte antigen type DR (mHLA-DR). These monocytes produce only small amounts of TNF-α and IL-1 in response to bacterial challenges. T cells from septic patients also have decreased human leukocyte antigen type DR (HLA-DR) expression, and ex vivo studies demonstrate that these cells are defective for antigen presentation. Numerous characteristics of immune suppression in sepsis have been identified. Nonetheless, researchers have yet to find diagnostic tests that can inform clinicians about the state of the immune system in septic patients. There are no discrete clinical signs or symptoms of immune dysfunction, and there is no gold standard available that can identify a patient in a state of immune suppression.
Identifying Immune Dysfunction in the Septic Patient
The host immune response to sepsis is complex and involves many circulating mediators and cells. Various cytokines and their correlation with mortality have been studied. Baseline circulating IL-6 and soluble TNF receptor have been shown to correlate with disease severity and 28-day all-cause mortality, and they may help in determining when an anti-inflammatory therapy may be of benefit. Levels of anti-inflammatory cytokines such as IL-10 may be more helpful in determining whether a patient is immunosuppressed ( Table 38-2 ). Elevated and sustained levels of IL-10 and high IL-10/TNF-α ratios were predictive of poor outcome. IL-10 correlated with the decreased expression of mHLA-DR in septic patients (another marker of sepsis-induced immunosuppression; see following paragraph) and may have mediated this finding. IL-10 may prove to be a useful marker of immune dysfunction, but it needs to be evaluated in larger clinical trials. For both pro- and anti-inflammatory cytokines, changes in circulating mediator levels may not correlate with biological activity. Sepsis is associated with changes in cytokine receptor levels and alterations in intracellular signaling pathways that make the biological consequence of circulating cytokine levels difficult to interpret.
Increased initial and sustained IL-10 levels |
High IL-10/TNF-α ratios |
Decreased mHLA-DR expression |

Full access? Get Clinical Tree
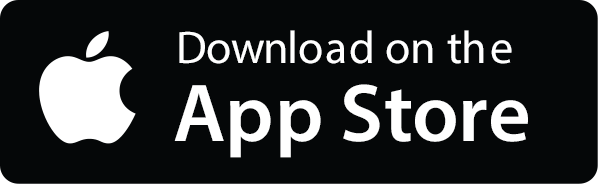
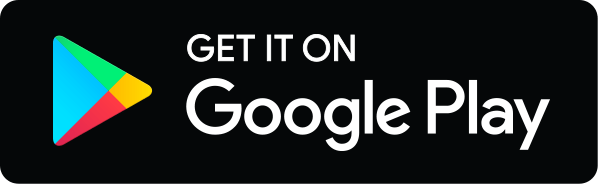
