Traditional approaches to the carbon dioxide (CO 2 ) management of adults with acute respiratory failure have focused on the potential for hypercapnia to exert deleterious effects. Support for this paradigm is derived from the association between hypercapnia and adverse outcome in diverse clinical contexts, including cardiac arrest, sepsis, and neonatal asphyxia. However, this approach has been increasingly questioned, particularly in the setting of acute severe respiratory failure. Accumulating evidence from experimental and clinical studies clearly demonstrates that high-stretch mechanical ventilation can directly injure the lungs, a phenomenon termed ventilator-induced lung injury. Mechanical ventilation strategies that reduce the intensity of mechanical ventilation, resulting in a respiratory acidosis termed permissive hypercapnia (PHC), improve outcome. Consequently, PHC has been progressively accepted in critical care for patients requiring mechanical ventilation. Conventionally, the protective effect of ventilatory strategies incorporating PHC is considered to be due solely to reduction in lung stretch, with hypercapnia “permitted” to achieve this goal. However, CO 2 is a potent biologic agent with the potential to exert beneficial and harmful effects. Furthermore, it is possible to minimize the potential for hypercapnia in the context of low-stretch ventilatory strategies by manipulating the respiratory frequency or by incorporating extracorporeal CO 2 removal (ECCO 2 R) technologies. Therefore it is important to fully understand the biologic effects of hypercapnia in the critically ill. To address these issues, we examine the physiologic effects of hypercapnia, insights that have emerged from studies of hypercapnic acidosis (HCA) in preclinical models, and data from clinical studies.
Physiology and Molecular Biology of Hypercapnia
Respiratory System
HCA improves oxygenation by reducing ventilation-perfusion ( <SPAN role=presentation tabIndex=0 id=MathJax-Element-1-Frame class=MathJax style="POSITION: relative" data-mathml='V˙/Q˙’>V˙/Q˙V˙/Q˙
V ˙ / Q ˙
) heterogeneity. HCA increases parenchymal lung compliance by enhancing alveolar surfactant secretion and function and by inhibiting actin-myosin interactions. Although, HCA can increase pulmonary vascular resistance and worsen pulmonary hypertension (PH), recent laboratory data suggest that moderate hypercapnia attenuates structural and functional changes in the pulmonary vasculature and reverses impaired right ventricular function in preclinical models. In acute respiratory distress syndrome (ARDS), PHC appears to increase shunt fraction because of a reduction in tidal volume (V t ) and airway closure rather than to hypercapnia per se. Hypercapnia directly dilates small airways but also stimulates vagal-mediated large airway constriction with an overall minor net effect on airway resistance. The impact of HCA on diaphragmatic function is controversial. HCA impairs diaphragmatic contractility and increases diaphragmatic fatigue in spontaneously breathing subjects. In contrast, it restores diaphragmatic dysfunction induced by prolonged mechanical ventilation in experimental models when minute ventilation is controlled. HCA also prevents myosin loss and inflammation in diaphragmatic tissue after prolonged ventilation. The clinical impact of hypercapnia on diaphragmatic function, especially with regard to weaning from mechanical ventilation, has yet to be elucidated.
Cardiovascular System
The direct depressive effects of HCA on the cardiovascular system are counterbalanced by its stimulatory effects on the sympathetic nervous system. HCA directly reduces the contractility of cardiac and vascular smooth muscle. However, hypercapnia-mediated sympathoadrenal effects, including increased preload and heart rate, increased myocardial contractility, and decreased afterload, lead to a net increase in cardiac output. Hypercapnia also results in a net increase in the partial pressure of oxygen in arterial blood (Pa o 2 ) and increases global O 2 delivery by elevating cardiac output. Hypercapnia and acidosis shift the hemoglobin-O 2 dissociation curve rightward, reducing the O 2 affinity of hemoglobin, and may cause an elevation in hematocrit level, further increasing tissue O 2 delivery. The concurrent reduced cellular respiration and O 2 consumption observed during acidosis may further improve O 2 supply-demand balance, particularly in the setting of compromised supply.
Tissue Oxygenation
CO 2 increases the cardiac index by 10% to 15% by each 10 mm Hg of partial pressure of oxygen in arterial blood (Pa o 2 ) increase and consequently improves subcutaneous tissue O 2 tension and muscle tissue O 2 saturation. In contrast, even a short period of hypocapnic alkalosis significantly reduces cardiac output, portal blood flow, gut perfusion, and O 2 delivery. Although a small randomized study (n = 30), suggested that mild intraoperative hypercapnia improves colon tissue oxygenation in nonselected and morbidly obese surgical patients, this effect did not reduce surgical site infection in a large recent multicenter randomized controlled trial (RCT) (n = 1206) of patients undergoing colon surgery.
Central Nervous System
Hypercapnia is a potent ventilatory stimulant. HCA improves cerebral tissue oxygenation by augmenting Pao 2 and cerebral blood flow (CBF). HCA dilatates precapillary cerebral arterioles, a function attributed to acidosis rather than hypercapnia per se. Indeed, cerebral vascular reactivity to CO 2 measured by transcranial Doppler ultrasound may be used as a risk predictor for ischemic stroke. HCA-mediated increases in CBF are a clear concern in the setting of reduced intracranial compliance in which increased global CBF may critically elevate intracranial pressure. Therefore traditional management of traumatic brain injury recommended sustained hypocapnia to reduce cerebral blood volume. However, this technique has fallen out of favor as a result of concerns over hypocapnia-induced hypoperfusion, resulting in cerebral tissue ischemia, increased risk of vasospasm, neuronal excitability, and seizures.
Intracellular Mechanisms of Action of Carbon Dioxide
The molecular effects of hypercapnia—both beneficial and harmful—are increasingly well understood. Hypercapnia inhibits nuclear factor-κB (NF-κB), a key transcriptional protein regulating gene transcription in lung injury, inflammation, and repair via mechanisms that include reduced degradation of its cytosolic inhibitor, IκBα. This mechanism protects the lung in the setting of ventilator-induced and ischemia-reperfusion–induced injury, but it also delays pulmonary epithelial wound repair after injury. Hypercapnia-mediated NF-κB inhibition may contribute to its immunosuppressive effects, by decreasing NF-κB–dependent antimicrobial peptide gene expression in Drosophila , and it decreases macrophage phagocytic activity and cytokine production. These effects may reduce injury in early pulmonary and systemic sepsis but worsen injury in prolonged untreated pneumonia in preclinical models.
Alveolar fluid clearance is an important step for the resolution of ARDS. Recent data indicate that CO 2 may facilitate Na + /K + -ATPase (sodium-potassium adenosine triphosphatase) pump endocytosis—a key ion pump responsible for Na + and fluid shift across alveolar epithelial cells—and delays fluid absorption by activation of protein kinases C and A and extracellular signal-regulated kinase (ERK)–mediated mitogen-activated protein (MAP)–kinase pathways.
Mechanical stretch has been shown to activate “shedding” of endogenous ligands, such as tumor necrosis factor receptor (TNFR), by metalloproteases (ADAM-17) that upregulate the epidermal growth factor receptor (EGFR) pathway and drive the inflammatory response. HCA inhibits shedding in stretch-induced lung injury, resulting in reduced activation of EGFR and P44/42 MAP-kinase pathways ( Fig. 31-1 ).

Role of Permissive Hypercapnia in Adult Critical Care
Acute Respiratory Distress Syndrome
The only therapeutic intervention to convincingly demonstrate a significant reduction in mortality in patients with ARDS and acute lung injury is lung-protective mechanical ventilation. The potential for protective lung ventilation strategies incorporating PHC to improve survival in patients with ARDS was suggested initially by Hickling and colleagues. Of the subsequent five prospective randomized controlled trials of protective ventilatory strategies, two demonstrated an impact of ventilator strategy on mortality, although three did not. Although to some extent PHC developed in all of the trials, there was much variability ( Table 31-1 ). Therefore, although it is clear that ventilation strategy can definitely affect mortality—in the positive trials—there is no discernible relationship between levels of CO 2 and survival among these data.
Trial | Mortality Benefit | Control Pa co 2 (mm Hg, mean ± SD) | “Protective” Pa co 2 (mm Hg, mean ± SD) | Buffering Permitted |
---|---|---|---|---|
ARDSnet Trial | Yes | 35.8 ± 8.0 | 40.0 ± 10.0 | Yes |
Amato et al. | Yes | 36.0 ± 1.5 | 58.0 ± 3.0 | No |
Stewart et al. | No | 46.0 ± 10.0 | 54.5 ± 15.0 | No |
Brochard et al. | No | 41.0 ± 7.5 | 59.5 ± 19.0 | No |
Brower et al. | No | 40.1 ± 1.6 | 50.3 ± 3.5 | Yes |
The database of the largest of these studies (ARMA) has been subsequently analyzed to determine whether, in addition to the effect of V t , there might also be an independent effect of HCA. Mortality was examined as a function of PHC on the day of enrollment using multivariate analysis and controlling for other comorbidities and severity of lung injury. It was found that PHC reduced 28-day mortality in patients randomized to the higher V t but not in those receiving lower V t . A recent pilot study including 20 ARDS patients compared the effectiveness of a new open lung ventilation strategy—a combination of PHC, staircase recruitment maneuvers, positive end-expiratory pressure (PEEP) titration, and targeted low plateau pressure—with lung-protective ventilation applied in the ARMA trial. During the 7-day observation period of lung compliance, the Pa o 2 /F io 2 (fraction of inspired oxygen) ratio was significantly better with the new open lung strategy. Of note, both ventilation strategies resulted in similar arterial CO 2 and pH values, suggesting that the observed benefits were more likely related to better lung recruitment than to PHC per se. Overall, although these clinical studies suggest that PHC may be beneficial in ARDS, they do not confirm it. Further appropriately designed randomized clinical studies are needed to elucidate the direct effect of PHC on acute lung injury.
Acute Severe Asthma
Controlled hypoventilation with PHC was first described in severe asthma by Darioli and Perret in 1984, predating its described use in ARDS. PHC facilitates a reduction of dynamic hyperinflation during mechanical ventilation in acute severe asthma by increasing the expiratory time, reducing inspiratory flow rates and V t . Multiple reports exist of the successful use of PHC in severe asthma, and modest levels of PHC (mean highest levels 62 mm Hg) are routinely used for patients in Europe and North America with acute severe asthma that requires assisted ventilation.
Chronic Obstructive Pulmonary Disease
Acute exacerbations of chronic obstructive pulmonary disease (COPD) result in diffuse airway narrowing, which increases airway resistance and respiratory workload, causing muscle fatigue and respiratory failure. Although noninvasive ventilation is the first-line ventilation strategy, extreme respiratory muscle fatigue may necessitate invasive ventilation. The rationale for the use of PHC in COPD is similar to that for acute severe asthma (i.e., it can reduce dynamic hyperinflation).
Role of Permissive Hypercapnia in Pediatric Critical Care
Neonatal Respiratory Distress Syndrome
Acute respiratory failure in the preterm newborn is most commonly associated with sepsis and meconium aspiration. Ventilation strategies involving PHC are well tolerated and may lower the risk of chronic lung disease (CLD) in preterm infants. In contrast, hypocapnia has been shown to be a strong prognostic factor for CLD in neonates. Mariani et al. demonstrated that PHC reduces the duration of ventilation support and speeds weaning from ventilation in preterm infants. A multicenter factorial trial of PHC and dexamethasone was stopped early because of unanticipated nonrespiratory adverse events related to dexamethasone therapy. In the trial, there was a trend toward a lower incidence of death and CLD in the PHC group, and only 1% of the PHC group patients required mechanical ventilation at 36 weeks’ gestational age compared with 16% in the normocapnia group. A Danish study demonstrated that a ventilatory strategy incorporating PHC, nasal continuous positive airway pressure, and surfactant therapy reduced the incidence of CLD. Of concern is the potential of severe hypercapnia to cause intracranial hemorrhage in premature infants. An early meta-analysis of PHC in newborn infants suggested that PHC may decrease the incidence of intracranial hemorrhage. However, more severe hypercapnia (55 to 65 mm Hg) can worsen neurologic outcomes and increase the incidence of combined mental impairment and death compared with normocapnia. Further research is needed to determine safe levels of hypercapnia to harness the beneficial and reduce the potentially harmful effects of PHC in preterm infants.
Congenital Diaphragmatic Hernia
Congenital diaphragmatic hernia (CDH) occurs in approximately 1 in every 3000 to 4000 births. Traditional approaches to CDH, which advocated aggressive control of PH with hyperventilation and alkalinization, have been largely abandoned because of concerns regarding increased mortality secondary to barotrauma. PHC (60 to 80 mm Hg) ventilation strategies can reduce barotrauma to the hypoplastic lung, and, in combination with delayed surgery, it increases survival and decreases the need for extracorporeal membrane oxygenation (ECMO) lower airway pressure ventilation. Consequently, PHC strategies remain the standard of care for pediatric patients with CDH.
Persistent Pulmonary Hypertension of the Newborn
Persistent pulmonary hypertension (PPH) of the newborn is a clinical syndrome of multifactorial etiology characterized by hypoxemia secondary to elevated pulmonary vascular resistance and right-to-left shunting of blood across the foramen ovale and/or ductus arteriosus. Traditional management strategies, which emphasized the use of hyperventilation to decrease pulmonary arterial pressure, have been superseded by concerns regarding adverse neurologic outcomes with hypocapnia. The safety of strategies limiting ventilator intensity, with resultant PHC, is supported by a number of relatively small clinical studies. In one study of 40 infants, less aggressive ventilation increased the survival of the sickest infants with PPH from 17% to 90%. Marron et al. reported 100% survival, better neurologic outcome with reduced sensorineural deafness, and less CLD in a case series of 34 infants with severe PPH and severe respiratory failure at birth managed with PHC.
Congenital Heart Disease
Manipulation of arterial CO 2 tension has traditionally played an integral role in the management of patients with complex congenital heart defects. Impaired neurodevelopmental outcome remains the major cause of morbidity in survivors after heart surgery. In this regard, the potential of hypercapnia to improve brain and other systemic organ oxygenation is increasingly recognized. Licht et al. have demonstrated that inspired CO 2 reverses the periventricular leukomalacia caused by low CBF in neonates with severe CHD. Hypercapnia increased cerebral oxygenation and mean arterial pressure in patients with hypoplastic left heart syndrome and after cavopulmonary connection. Hypoventilation improved systemic oxygenation after bidirectional superior cavopulmonary shunt, potentially via a hypercarbia-induced decrease in cerebral vascular resistance, thus increasing cerebral, superior vena caval, and pulmonary blood flow. Finally, inspired CO 2 improved CBF and systemic oxygenation after cavopulmonary shunt. Taken together, these studies raise the potential that inhaled CO 2 might have a future therapeutic role in this context.

Full access? Get Clinical Tree
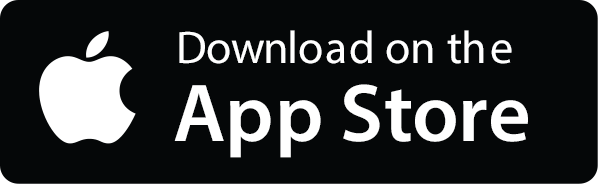
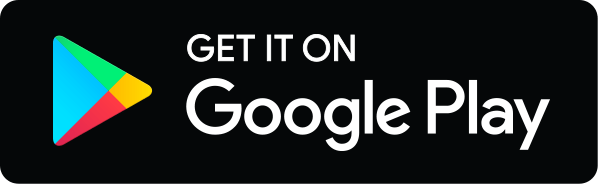