There has been a dramatic reawakening of interest in therapeutic hypothermia (TH) since the dawn of the twenty-first century as a consequence of the publication of a series of clinical trials that appeared to demonstrate improved clinical outcomes. The major attraction of TH is the combination of low cost, reproducibility, and simplicity. A multitude of animal studies have suggested that TH may be beneficial in clinical conditions that may result in neurologic injury.
Several mechanisms that mediate the protective effects of hypothermia have been identified. However, the overall response probably results from a combination of multiple mechanisms that vary with the level and duration of hypothermia. Thus the level of hypothermia to be used in different settings may vary widely: deep hypothermia (15 to 22 °C) is used in cardiac surgery to enable circulatory arrest while mild hypothermia (32 to 34 °C) is used to improve outcome after cardiac arrest (CA) and other ischemia/reperfusion events. Hypothermia can be induced simply, with surface cooling or with sophisticated techniques with specially designed catheters and blankets. The almost universal ability to induce hypothermia makes it a widely applicable, highly attractive approach.
Despite its long history, the widespread clinical application of hypothermia is a relatively new phenomenon. Two seminal clinical trials published in 2002 demonstrated the benefit of therapeutic hypothermia after CA. Recent findings suggest that even mild hypothermia (36 °C) may have favorable physiologic effects and that avoidance of hyperthermia may be a desirable clinical goal. In this chapter, we focus on mild to moderate hypothermia that does not require the use of cardiopulmonary bypass and can be accomplished in an intensive care unit (ICU).
Temperature Monitoring
The normal body temperature in healthy individuals (measured in the oral cavity) is 36.8 ± 0.4 °C with normal diurnal variations of 0.5 °C. Rectal temperatures are usually 0.4 °C higher than oral readings. Lower-esophageal temperature closely reflects the core temperature, as well as rectal temperature and bladder temperature. The temperature measured with pulmonary artery catheters most closely correlates with brain temperature during rapid cooling. Clinically, tympanic temperature, which measures radiating heat from the tympanic membrane, is often used as a surrogate for deep brain temperature. On the basis of the method of cooling, the difference in temperature between various monitoring sites could be significant. In addition, there is no generally accepted, clearly defined range for various levels of hypothermia. In clinical practice, temperatures of 33 to 36 °C are usually referred to as mild hypothermia, 28 to 32 °C as moderate hypothermia, and below 28 °C as deep hypothermia.
Cooling Methods
Traditionally, external cooling with ice packs applied over great vessels or ice-water soaked cloth blankets has been used to treat hyperthermia and, eventually, induce hypothermia. Gastric, peritoneal, or pulmonary lavage was used to rewarm drowning victims, and this approach could be used in reverse for cooling. Recently, cooling with a rapid intravenous (IV) infusion of ice-cold solutions gained popularity for its ease, general availability, and considerable lack of adverse effects even in CA victims. Bernard et al. used large volumes (30 mL/kg) of ice-cold (4 °C) IV fluid in CA victims and was able to decrease the core temperature from 35.5 to 33.8 °C within 30 minutes. Using a similar approach, Kim et al. achieved a 1.5 °C temperature decrease over 30 minutes. Most important, they did not observe any clinically important changes in vital signs, electrolytes, arterial blood gases, or coagulation parameters. Although IV fluids can initiate cooling effectively, they are not effective for maintaining hypothermia. Cooling blankets with circulating water offer fairly rapid cooling but require attaching a bulky control console to the patient. Similar limitations apply to intravascular cooling catheters. However, both contemporary surface cooling devices and intravascular cooling catheters are able to maintain hypothermia precisely. Kliegel et al. successfully combined the rapid induction of hypothermia with IV fluids and subsequent cooling with an intravascular catheter. Submersion in ice water represents the fastest cooling method (0.11 to 0.25 °C/min). This approach may be useful in heat stroke victims but is unlikely to be feasible in the ICU. An effort to eliminate the potential complications associated with whole-body hypothermia led to the development of devices to induce selective brain hypothermia. Cooling helmets have been used in multiple trials in both pediatric and adult populations. Other techniques that might provide more rapid cooling are being explored. Such techniques include nasopharyngeal cooling, neck cooling, and direct cooling of blood in the carotid arteries. Cooling with extracorporeal circulation is extremely effective, but its use is logistically limited.
Complications Associated with Therapeutic Hypothermia
Hypothermia initiates multiple physiologic changes in the circulatory, respiratory, neurologic, immunologic, and coagulation systems. It also has profound metabolic effects. These changes are temperature dependent. Mild hypothermia most often induces sinus tachycardia. More dangerous cardiovascular complications usually are seen at temperatures below 30 °C. These include atrial fibrillation, bradycardia, and terminal ventricular fibrillation (VF) at approximately 25 °C. The mild hypothermia currently used in clinical practice is hemodynamically well tolerated, with an approximately 25% decrease in cardiac output and an increase in systemic vascular resistance and central venous pressure. In healthy subjects, mild hypothermia increased myocardial perfusion. Hypothermia also induces the release of endogenous catecholamines with a fourfold to sevenfold increase in norepinephrine levels even with minimal temperature changes (0.7 to 1.2 °C). This adrenergic response is associated with an increase in blood pressure, vascular tone, and oxygen consumption that could be detrimental in patients with marginal cardiac reserve.
The impact of hypothermia on coagulation is a result of platelet depletion or dysfunction and clotting factor depletion. The magnitude of changes is often difficult to assess because clinical laboratories adjust the temperatures of all samples to a standard 37 °C. The effects of hypothermia on coagulation thus may be undetected. Reed and colleagues cooled plasma containing clotting factors equivalent to 100% of normal to 35, 33, and 31 °C. Partial thromboplastin time (PTT) in these samples was prolonged as if factor IX had been depleted to 39%, 16%, and 2.5% of normal, respectively. Factor activity is also severely impaired below 30 °C; for example, at 25 °C clotting activity ranges from 0% (factor VIII and factor IX) to 5% (factor II and factor VII). This suggests that factors are dysfunctional, not depleted, because the changes were observed despite 100% or greater factor concentrations measured in the studied samples. Thromboelastography (TEG) may be a useful tool in the setting of TH. TEG from hypothermic swine (32 °C) showed prolonged initial clotting time (R time) and decreased clotting rapidity (alpha angle). These changes suggest a deficit in thrombin availability and/or delay in thrombin generation or activation but not a decrease in clot strength or an increase in clot lysis. Other TEG-based studies suggest that clot firmness is decreased in temperatures less than 30 °C. Bleeding time, one indicator of platelet function, was prolonged 2.5-fold in a sample from a cold (32 °C) versus warm (37 °C) extremity in baboons. In a similar experiment in human volunteers, clotting times were three times longer at 22 °C than at 37 °C. Concurrent acidosis and hypothermia further impaired coagulation. These effects should be taken into consideration when resuscitating trauma victims with ongoing bleeding. Systemic and local normothermia is essential for coagulation. However, trials indicate that neither mild nor moderate therapeutic hypothermia is associated with bleeding complications in patients with severe traumatic brain injury (TBI). In patients after CA, there was a trend toward higher bleeding in patients treated with hypothermia (relative risk [RR], 1.30; 95% confidence interval [CI], 0.97 to 1.74), which did not reach significance ( P = .085).
Hypothermia may lead to leukopenia and an increased risk of infection. Several studies in patients after CA, TBI, or acute stroke showed an increased risk of pneumonia, especially when the duration of hypothermia was prolonged (>48 to 72 hours). Shorter hypothermic periods (<24 hours) appear to be safer. Recent meta-analysis confirms increased risk for pneumonia and sepsis (RR 1.44 [95% CI, 1.10 to 1.90]; 1.80 [95% CI, 1.04 to 3.10], respectively), but the prevalence of all infections was not increased (rate ratio, 1.21 [95% CI, 0.95 to 1.54]). Obviously, the trade off of increased infection rate may be worthwhile if greater neuroprotection can be achieved.
Electrolyte disorders, although common in TH, are usually minor and can be treated easily in a critical care setting. The most commonly observed abnormalities are hypernatremia and hypokalemia, as well as hypomagnesemia, hypophosphatemia, and hypocalcemia. Magnesium supplementation may be especially important given its known protective role in neuronal and myocardial injury.
Hypothermia-induced decreases in insulin sensitivity may lead to hyperglycemia. This could enhance the susceptibility to infection and also might exacerbate secondary brain injury. Tight glycemic control may be warranted, although work by Vespa et al. suggests caution and use of insulin at a higher glucose target level of probably more than 150 mg/dL (8 mmol/L).
Drug metabolism is profoundly altered by hypothermia. Some drugs are affected more than others. Mild to moderate hypothermia decreases systemic clearance of cytochrome P450-metabolized drugs by approximately 7% to 22% per degree Celsius below 37 °C. Hypothermia decreases the potency and efficacy of certain drugs.
Mechanism of Action of Hypothermia
Cerebral metabolic rate is decreased by 5% to 7% for each degree decrease in body temperature. However, this observation does not explain the ability of even small temperature changes to affect physiology and provide neuroprotection. Protection by hypothermia in experimental central nervous system (CNS) injury might involve a myriad of mechanisms: maintenance of physiologic adenosine triphosphate (ATP) concentrations, suppression of glutamate release, attenuation of oxidative or nitrative stress, blunting of the inflammatory response, prevention of energy failure, limitation of cytoskeletal damage, increased levels of neurotrophins, prevention of anoxic depolarization, regulation of gene expression, attenuation of apoptosis or limitation of blood-brain barrier injury, and vasogenic edema. In TBI or ischemic stroke, TH reduces intracranial pressure (ICP). However, direct neuroprotection has been more difficult to demonstrate outside of the laboratory.
Various combinations of those mechanisms could be responsible for the different outcome in the wide variety of CNS injuries, with hypothermia being beneficial in only selected settings.
Hypothermia in Cardiac Arrest
It has been known for decades that patients who undergo accidental hypothermia, such as near drowning in cold water, survive a much longer period of CA than would be expected if the accident happened at ambient temperature. The initial case series of therapeutic hypothermia applied to victims of CA of various origin (e.g., respiratory failure, trauma) was published in 1958. The target temperatures and duration of cooling were 30 to 34 °C for 24 to 72 hours. In 1959, Benson and colleagues reported the first case series of in-hospital CA patients. Their data revealed favorable neurologic recovery in 50% in the hypothermic group versus 14% in the normothermic group. Despite these early promising results, the clinical use of hypothermia was abandoned, for unclear reasons, until the late 1990s. It is possible that the complications associated with deeper levels of hypothermia (<30 °C) and prolonged use, as observed in animal studies, played a role. Laboratory studies in the 1980s explored the potential of mild hypothermia to protect while limiting complications. Busto and colleagues found that small increments in intraischemic temperatures (33, 34, 36, and 39 °C) translate into large differences in neuronal loss in a rat model. Safar’s group followed that work showing benefit in experimental CA. These studies provided evidence that even mild hypothermia could significantly improve outcome in CA.
Timing of hypothermia induction also is critical. Initiating hypothermia during the insult yields the best outcome but is rarely clinically feasible. Delayed hypothermia is beneficial in the early postinsult period, but the effect declined over time. On the basis of studies by Colbourne and colleagues in gerbils, minimal delay and longer duration are of utmost importance to fully benefit from hypothermia. Prehospital initiation of cooling in the clinical setting is feasible, and decreased time to target temperature surprisingly was not beneficial, possibly because it was associated with higher incidence of complications linked to IV cooling with fluids.
Several randomized human trials assessed the efficacy of hypothermia after CA. After a small study by Hachimi-Idrissi et al., two studies published in 2002 clearly established the value of hypothermia in CA. Bernard et al. in Australia studied 77 patients after CA from VF. The patients assigned to hypothermia were cooled to 33 °C over 12 hours with ice packs. A total of 21 (49%) of 43 patients in the hypothermic group survived with good neurologic outcome, whereas this was noted in only 9 (26%) of 34 patients who were not cooled ( P = .046). The odds ratio (OR) for a good outcome with hypothermia was 5.25 (95% CI, 1.47 to 18.76; P = .011). In the European multicenter Hypothermia After Cardiac Arrest (HACA) trial, patients resuscitated after CA from VF or ventricular tachycardia (VT) were randomly assigned to hypothermia (32 to 34 °C for 24 hours, cooling with cold air) or to normothermia. In the hypothermia group, 75 (55%) of 136 patients showed favorable neurologic outcome compared with 54 (39%) of 137 in the normothermic group (RR, 1.68; 95% CI, 1.29 to 2.07; number needed to treat [NNT] = 6). Hypothermia appeared effective despite a relative delay in initiation and slow onset. Three questions arose after publication of these trials: (1) Were these studies of hypothermia versus hyperthermia, rather than normothermia (fever was a frequent complication in the control groups)? (2) Is it necessary to cool the patient below 34 °C, with associated problems such as shivering and the requirement for deep sedation, to have a beneficial effect? (3) Is TH effective for CA caused from events other than VF/VT?
As TH began to be used routinely, several registries for follow-up were established. Arrich et al. evaluated the data from 650 patients from 19 centers entered into the European Resuscitation Council Hypothermia After CA Registry. Of all patients, 462 (79%) received TH, 347 (59%) were cooled with an endovascular device, and 114 (19%) received other cooling methods such as ice packs, cooling blankets, or cold fluids. The rate of adverse events was lower (hemorrhage, 3%; arrhythmia, 6%), and the cooling rate was faster than in published clinical trials.
Lopez-de-Sa et al. compared hypothermia at 32 °C versus 34 °C, concluding that lower temperature was associated with more favorable outcomes, and lower incidence of clinical seizures (1/18 vs. 11/18; P = .0002) in patients with shockable rhythms.
As a result of these studies the International Liaison Committee on Resuscitation recommended that, “Unconscious adult patients with spontaneous circulation after out-of-hospital CA should be cooled to 32 °C to 34 °C for 12 to 24 hours when the initial rhythm was VF. Cooling to 32 °C to 34 °C for 12 to 24 hours may be considered for unconscious adult patients with spontaneous circulation after out-of-hospital CA from any other rhythm or CA in hospital.”
Oksanen et al. reviewed the data from CA survivors admitted to Finnish ICUs between 2004 and 2005. Almost all ICUs used hypothermia (19 of 20), but it seemed to be implemented only in selected groups of patients (4% in 2004, 28% in 2005). Survival rate at 6 months was 55%. It was estimated that if physicians in the United States were to use TH in all eligible patients, 2298 additional patients per year might achieve a good neurologic outcome.
However, not all studies have reported improved outcomes with TH. Tiainen et al. studied cognitive and neurophysiologic outcome in a cohort of 70 patients randomly assigned to hypothermia (33 °C for 24 hours) or normothermia. Three months after CA, 28 of 36 patients in the hypothermic group compared with 22 of 34 in the normothermic group were alive ( P = .226). There was no difference in cognitive decline or neurophysiologic deficits.
Although TH has been widely adopted in ICUs worldwide, with anecdotal reports of improved outcomes, there remained the problems of selection bias, temperature control, and study size in the Bernard and HACA studies. Nielsen and colleagues undertook a large multicenter trial that randomized CA patients to targeted temperature management (TTM) at 33 or 36 °C for 24 hours, with gradual rewarming at temperature control to 37 °C for up to 72 hours. The primary endpoint of the study was survival at 180 days after inclusion. At 72 hours, 50% of the patients in the 33 °C group (235 of 473 patients) had died, compared with 48% of the patients in the 36 °C group (225 of 466 patients) (OR with a temperature of 33 °C, 1.06; 95% CI, 0.89 to 1.28; P = .51). At the end of the 180-day follow-up period, 54% of the patients in the 33 °C group had died or had poor neurologic outcome, compared with 52% of patients in the 36 °C group (OR, 1.02; 95% CI, 0.88 to 1.16; P = .78). There was no benefit to cooling below 36 °C. There was no evidence that early versus late cardiopulmonary resuscitation (CPR), duration of CPR, or time to hospital admission had any discernable impact on outcomes. On the basis of these findings, current guidelines suggest TTM at 32 to 36 °C (mild hypothermia).
It remains for future trials to determine if the benefits conferred by TTM or mild to moderate hypothermia can be extended to other settings. Recent meta-analysis of patients presenting with nonshockable rhythms documented reduced in-hospital mortality with hypothermia, but the quality of studies was low. Several additional studies that were not included in that meta-analysis supported this observation. A review of low-quality trials in a pediatric population demonstrated no difference in mortality, but no recommendations were drawn. A strict control of the temperature avoiding hyperthermia (>37.5 °C) and severe hypothermia (<32 °C) is recommended in children. Data from in-hospital CA are emerging, but the initial results are not compelling. The optimal selection of patient population is being debated. Multiple trials are underway. Prognostication of outcome of both adult and pediatric patients treated with hypothermia after CA remains challenging.
Therapeutic Hypothermia in Ischemic Stroke
In animal models of focal brain ischemia, hypothermia has been shown to reduce lesion size by up to 90%. These data resulted in interest in the use of TH in patients with ischemic stroke. Brain temperatures in stroke patients exceed core temperature by at least 1 °C (1.0 to 2.1 °C). A large cohort trial of 3790 patients demonstrated that the avoidance of hyperthermia in stroke patients improved outcome. However, a pharmacologic-based strategy to induce hypothermia with acetaminophen (paracetamol) resulted in a body temperature decrease of only 0.22 °C. Schwab et al. conducted two noncontrolled trials in patients who underwent acute ischemic stroke to evaluate the effect of hypothermia (33 °C for 24 to 72 hours). In the first study, hypothermia was initiated in 25 patients 14 hours after first symptoms (range, 4 to 24 hours). Target temperature was achieved after 3 to 6 hours. Passive rewarming was achieved over 18 hours (range, 17 to 24 hours). ICP decreased in all patients during hypothermia, but significant increases in ICP were observed during rewarming. Pneumonia was observed in 40% of patients. In the second study, 50 patients were subjected to hypothermia in a manner similar to that in the previous study. ICP decreased from 20 ± 14 to 12 ± 5 mm Hg during hypothermia. Shorter rewarming periods (<16 hours) were associated with a marked ICP increase and higher mortality when compared with longer rewarming periods. Mortality in this study was 38%, which compares favorably with outcomes of other studies with similar patient populations without hypothermia having mortality rates of 78% to 79%, although Hawthorne effects and selection bias should be considered.
Kammersgaard et al. used hypothermia (35.5 °C for 6 to 17 hours) in 17 awake stroke patients and compared the outcome data with matched subjects from the Copenhagen registry. Neurologic impairment as assessed by the Scandinavian Stroke Scale at 6 months was similar (42 ± 14 vs. 48 ± 11, respectively; P = .21).
De Georgia and colleagues conducted a feasibility trial of 40 patients randomized to intravascular cooling (33 °C for 24 hours) or control therapy after ischemic stroke. Clinical outcomes and lesion size at one month were similar in both groups. No adverse side effects were observed.
Hemicraniectomy represents the most invasive approach to treat ischemic stroke. Georgiadis et al. randomized 36 patients to either hemicraniectomy or hypothermia. Mortality was 12% in hemicraniectomy compared with 47% in hypothermia. The latter also was associated with a higher complication rate. Els et al. compared hypothermia with hemicraniectomy (HH) (n = 12) to hemicraniectomy alone (HA) (n = 13), with a trend toward better outcome in the HH at 6 months ( P < .08).
Thus there currently are no robust data to support the use of induced hypothermia in patients with ischemic stroke. Recent meta-analysis of seven small clinical trials did not show any benefit on mortality or stroke severity. However, some trials report benefits on incidence and severity of complications associated with stroke and on outcome. Given that several small trials suggested benefit, additional larger trials are planned or underway.
Hypothermia for Spinal Cord Injury
There are a limited number of studies addressing the use of hypothermia after traumatic spinal cord injury (SCI). The results from the animal studies are mixed but overall suggest beneficial effects. However, the models are varied. Several case series using whole-body hypothermia for SCI have been published, documenting feasibility. Regional cooling of spinal cord is a viable alternative. This approach, reviewed by Kwon et al., was assessed in small case series in 1970s and 1980s. Despite some encouraging results, the authors of all respective studies acknowledge the limitations (e.g., small number of patients, differences in clinical assessment of deficits, and lack of controls) and the need for larger controlled studies. A large multicenter prospective study (ARCTIC [Acute Rapid Cooling Trial for Injuries of the Spinal Cord]) is currently proposed.
Currently, there is emerging evidence suggesting that hypothermia might be beneficial after traumatic SCI but definitive studies are still lacking. Preventive induction of regional hypothermia for major vascular procedures with or without additional measures including systemic hypothermia has not been validated in a large prospective randomized study.
Hypothermia for Traumatic Brain Injury
Current diagnostic and therapeutic approaches to patients with TBI vary widely between institutions. Treatments are generally targeted at maintaining cerebral perfusion pressure, minimizing ICP, or reducing overall brain metabolic activity. In TBI, neuronal death is biphasic: early, due to the injury itself, and late, a consequence of hypoxic-ischemic and inflammatory damage.
Many experimental studies in animals have suggested benefit with the use of TH after TBI. Multiple RCTs have been conducted, investigating the use of hypothermia after TBI in both pediatric and adult populations. Be aware: the pathophysiology of TBI in pediatric and adult patients has distinct features that could contribute to different outcomes. The depth and duration of hypothermia applied have varied widely, as have the use of other therapeutic modalities (e.g., cerebrospinal fluid [CSF] drainage, osmotic therapy, and sedation). Better results were achieved in centers with expertise in applied hypothermia. Several published meta-analyses reported conflicting results ( Table 22-1 ): some indicated improved neurologic outcome and mortality when hypothermia was used, but others did not support that observation. No improvement in outcome with hypothermia was seen in the five pediatric studies. Varied results were reported in 14 studies on adult patients, 2 of which reported a tendency of higher mortality and worse neurologic outcome, 4 reported lower mortality, and 9 reported favorable neurologic outcome with hypothermia. The quality of several trials was low. More favorable results were observed in Asian populations versus American populations. Recent meta-analysis of pediatric patients with TBI documented increased mortality and increased incidence of cardiac arrhythmias with hypothermia.
Study, Year | Number of Trials | Number of Subjects (Intervention/No Intervention) | Intervention | Control | Outcomes |
---|---|---|---|---|---|
Cardiac Arrest | |||||
Holzer, 2005 | 3 | 195/198 | Hypothermia | Standard care | Improved survival with favorable neurologic recovery—RR = 1.68 (1.29-2.07) |
Cheung, 2006 | 4 | 231/203 | Hypothermia | Standard care | Reduced mortality—RR = 0.75 (0.62-0.92), Reduced poor neurologic outcome—RR = 0.72 (0.62-0.84) |
Nielsen, 2011 | 5 | 254/224 | Hypothermia | Standard care | Nonsignificant reduction in mortality—RR = 0.84 (0.70-1.01) and reduction in poor neurologic outcome—RR = 0.78 (0.64-0.95) |
Arrich, 2012 | 5 | 253/226 | Hypothermia | Standard care | Hypothermia improved good neurologic outcome—RR = 1.55 (1.22-1.96) and survival—RR = 1.35 (1.10-1.65) |
Traumatic Brain Injury in Adults | |||||
Harris, 2002 | 7 | 254/245 | Hypothermia | Standard care | Nonsignificant improvement in GOS—OR = 0.61 (0.26-1.46, P = .3); decrease of ICP—OR = −2.98 (−7.58-1.61; P = .2); significant prolongation of PTT—OR = 2.22 (1.73-2.71; P < .001) |
Henderson, 2003 | 8 | 748 total | Hypothermia | Normothermia | Nonsignificant reduction of mortality—OR 0.81 (0.59-1.13), strong trend for reduction of poor neurologic outcome—OR 0.75 (0.56-1.01; P = .06); reduced risk of pneumonia in normothermic group OR—0.42 (0.25-0.70; P = .001) |
McIntyre, 2003 | 12 | 543/526 | Hypothermia | Normothermia | Reduced mortality—RR = 0.70 (0.56-0.87) and reduced poor neurologic outcome—RR = 0.65 (0.48-0.89) |
Alderson, 2004 | 14 | 540/523 | Hypothermia | Open or normothermia | Nonsignificant reduction in mortality—OR = 0.80 (0.61-1.04); nonsignificant reduction of mortality or severe disability—OR = 0.75 (0.56-1.00); increase risk of pneumonia—OR = 1.95 (1.18-3.23) |
Brain Trauma Foundation, 2007 | 6 | 354/340 | Hypothermia | Normothermia | All-cause mortality not significantly different—RR = 0.76 (0.55-1.05; P = .16); increased chance of good outcome—RR = 1.46 (1.12-1.92); cooling >48 hr associated with reduction of mortality—RR = 0.51 (0.24-0.78) |
Peterson, 2008 | 8 | 407/374 | Hypothermia | Normothermia | Reduction in mortality—RR = 0.51 (0.33-0.79) and favorable neurologic outcome—RR = 1.91 (1.28-2.85) when hypothermia used for >48 hr; increased risk of pneumonia—RR = 2.37 (1.37-4.10); no benefit for cooling <48 hr |
Sydenham, 2009 | 23 | 803/784 | Hypothermia | Normothermia | No reduction in mortality—OR = 0.85 (0.68-1.06); hypothermia associated with less likely unfavorable outcome—OR = 0.77 (0.62-0.94) |
Georgiu, 2013 | 18 | 917/910 | Hypothermia | Normothermia | Reduction in mortality—RR = 0.84 (0.72-0.98] and of poor neurologic outcome—RR = 0.81 (0.73-0.89) |
Li, 2014 | 13 | 591/561 | Hypothermia | Normothermia | Trend to reduction in mortality—RR = 0.86 (0.73-1.01; P = .06) and unfavorable clinical neurologic outcomes—RR = 1.21 (0.95-1.53; P = .12); significant reduction of mortality with hypothermia in an Asian population—RR = 0.60 (0.44-0.83, P = .002) |
Crossley, 2014 | 20 | 863/976 | Hypothermia | Normothermia | Reduction in mortality—RR = 1.31 (1.13, 1.52; P = .0004); reduction in poor outcome—RR = 1.49 (1.27, 1.74; P < .00001) |
Traumatic Brain Injury in Children | |||||
Ma, 2013 | 6 | 366 total | Hypothermia | Normothermia | Increased mortality with hypothermia—RR = 1.73 (1.06-2.84) |
Hypoxic Ischemic Encephalopathy in Newborns | |||||
Jacobs, 2008 | 8 | 255/251 | Hypothermia | Normothermia | Reduced risk of death or major disability—RR = 0.76 (0.65-0.89) |
Jacobs, 2013 | 11 | 688/612 | Hypothermia | Normothermia | Reduction in mortality—RR = 0.75 (0.64-0.88); reduction in neurodevelopmental disability in survivors—RR = 0.77 (0.63-0.94) |
Pauliah, 2013 | 7 | 301/266 | Hypothermia | Standard care | Data from low- and middle-income countries; no reduction in neonatal mortality—RR = 0.74 (0.44-1.25); data on morbidity and long-term neurologic outcomes were insufficient |
Intracranial Aneurysm | |||||
Milani, 2011 | 4 | 605/611 | Hypothermia | Normothermia | No difference in outcome or complications |
Li, 2012 | 577/581 | Hypothermia | Normothermia | No effect on mortality—RR = 0.82 (0.62-1.09) | |
Zhao, 2012 | 3 | 575/584 | Hypothermia | Normothermia | No difference in outcome or complications |
Stroke | |||||
Lakhan, 2012 | 7 | 131/127 | Hypothermia | Normothermia | No difference in mortality or stroke severity |

Full access? Get Clinical Tree
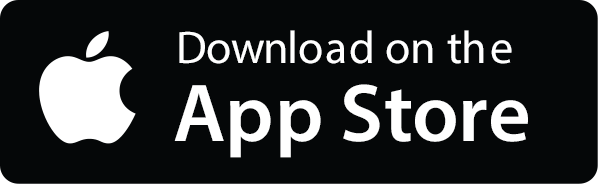
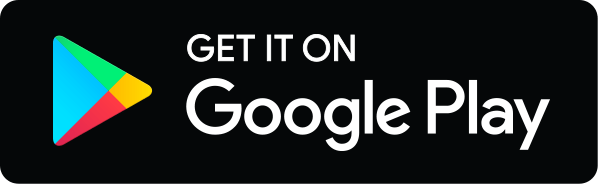
