Chapter Outline
Invasive Arterial Blood Pressure Monitoring 148
General Indications For Arterial Blood Pressure Monitoring 148
Sites For Arterial Catheterization 148
Insertion Of An Arterial Catheter 149
Contraindications To Arterial Cannulation 149
Complications 149
Other Considerations 149
Cardiac Output 150
Central Venous Pressure Monitoring 150
Indications For Central Venous Catheterization 150
Sites For Central Venous Catheterization 151
Insertion Of a Central Venous Catheter 152
Complications Of Central Venous Catheterization 152
Central Venous Pressure Waveforms 152
Pulmonary Artery Catheter 152
Indications For Pulmonary Artery Catheterization 152
Complications Associated With Pulmonary Artery Catheterization 152
Insertion Of Pulmonary Artery Catheter 154
Determination Of Cardiac Output Using The Pulmonary Artery Catheter 156
Limitations In Using The Pulmonary Artery Catheter 156
Less Invasive Monitors For Cardiac Output 156
Esophageal Doppler 156
Indirect Fick Partial Rebreathing Method 157
Transpulmonary Thermodilution 157
Lithium Dilution Cardiac Output 157
Arterial Pulse Contour Analysis 157
Bioimpedance Cardiography 158
Echocardiography (see also Chapter 11 ) 158
The goals of hemodynamic monitoring in the operating room are to maintain optimal perfusion and oxygen delivery to tissues, ensure rapid detection of changes in clinical status, and monitor for response to surgery, anesthesia, resuscitation, and therapies. Although noninvasive monitors (such as a blood pressure cuff) are associated with less risks and complications, it is often necessary to use invasive monitoring techniques to achieve these goals, particularly in high-risk patients. The necessity for invasive monitoring may be driven by patient condition or by the surgical procedure. In general, invasive hemodynamic monitoring is indicated if significant physiological disturbances are anticipated based on patient comorbidities or the surgical procedure. Examples of patient comorbidities that may warrant invasive monitoring include previous cardiopulmonary illness, multiple trauma, age greater than 70 years and/or limited reserve, septicemia or other form of shock, respiratory failure, acute renal failure, and vascular disease.
Invasive Arterial Blood Pressure Monitoring
Invasive arterial monitoring is the “gold standard” blood pressure device. In addition to being the most accurate form of blood pressure monitoring, arterial cannulation allows continuous beat-to-beat monitoring. It also serves as a site for obtaining serial lab measurements of oxygenation, ventilation, pH, lactate, base deficit, electrolytes, and hematological data.
General Indications for Arterial Blood Pressure Monitoring
- ▪
Hemodynamic instability or predicted hemodynamic instability.
- ▪
Surgical procedures that will be prolonged, involve major fluid shifts, or significant blood loss.
- ▪
Monitoring the safety of certain anesthetic techniques, such as deliberate hypotension, cardiopulmonary bypass, or major vascular surgery involving arterial clamping.
- ▪
Monitoring the response to vasoactive drugs.
- ▪
Frequent blood gas measurements.
- ▪
Frequent blood sampling needed in patients without central venous access.
- ▪
Patients who are severely obese, have burned extremities, or are in shock (where noninvasive blood pressure monitoring may not be feasible).
Sites for Arterial Catheterization
The most common sites for arterial cannulation are radial or femoral arteries, but other arteries including the brachial, axillary, and dorsalis pedis can also be used. Axillary or brachial arterial catheterization, though feasible, may be associated with increased risk of extremity ischemia because they are end-arteries. Choice of artery is dependent on the ability to palpate a pulse or to locate it by Doppler ultrasonography. It may be prudent to assess the collateral circulation if cannulating the smaller arteries such as the radial or dorsalis pedis arteries. However, the commonly used Allen’s test has not been shown to be a reliable way of evaluating collateral circulation in the hand. The extremity distal to the catheter should be assessed frequently both before and after insertion, and the catheter should be removed immediately if there is evidence of ischemia. The risk of clinically significant arterial thrombosis is decreased if the catheter is continuously flushed.
Options for Arterial Catheterization
The radial artery can be palpated on the distal portion of the forearm between the radius and the tendon of the flexor carpi radialis.
The brachial artery can be palpated as it courses medial to the biceps muscle and tendon into the antecubital fossa, with the arm extended and the palm facing up.
The axillary artery may be best palpated in the axillary space, with the arm abducted and externally rotated.
The femoral artery may be best palpated below the inguinal ligament, midway between the anterior superior iliac spine and the symphysis pubis.
The dorsalis pedis artery may be palpated on the arch of the foot, between the first and second metatarsals.
Figure 10–1 shows the normal waveforms associated with pressures of the aortic root, brachial artery, radial artery, and femoral artery. Systolic pressure increases with progression away from the aorta due to a counterreflection phenomenon in which the forward-traveling wave generated by left ventricular ejection meets a later-arriving reflected wave from the periphery. In patients with significant peripheral arterial disease (and calcified vessels), this phenomenon is more pronounced. However, the mean arterial pressure (MAP) should be equivalent at all arterial sites.

Insertion of an Arterial Catheter
The most common method for gaining access into any desired vessel is the Seldinger technique, which was initially described by Sven-Ivar Seldinger in 1953. The technique begins by locating the artery with an introducer needle or catheter. Next a guidewire is passed through the lumen of the introducer needle/catheter and the introducer is removed leaving only the guidewire in the vessel. A soft dilator may be passed over the guidewire and then removed to dilate the skin and soft tissues leading to the artery. Finally, the arterial catheter is passed over the wire into the artery and the wire is removed. Special arterial catheterization kits are available in which the guidewire and arterial catheter are attached to the introducer needle, reducing the number of steps necessary and minimizing blood loss during the procedure. Figure 10–2 , A, shows the Seldinger technique (without the dilator step) and Figure 10–2 , B, shows the modified technique with use of a specialized arterial catheter.


Contraindications to Arterial Cannulation
Absolute contraindications to cannulation include localized infection at the site of insertion, preexisting ischemia, nerve damage, Raynaud phenomenon, or traumatic injury proximal to the site of insertion. It is usually possible to identify another artery that is amenable to cannulation. Relative contraindications include failure to demonstrate collateral flow in small vessels (e.g., by Doppler ultrasonography), presence of an arteriovenous fistula in the same limb, and history of surgery disrupting the lymphatics of the same limb (e.g., axillary lymphadenectomy). If there are no other arterial sites that can be used, these relative contraindications may be outweighed by other considerations.
Complications
Complications of invasive arterial blood pressure monitoring may be related to the placement of the catheter, care of the indwelling catheter, length of time the catheter is in place, or patient characteristics. Table 10-1 lists the complications associated with arterial cannulation and precautions to decrease these risks.
Other Considerations
As with all invasive pressure monitors, the transducer height should be level with the right atrium. An exception to this rule is when monitoring cerebral perfusion pressure, in which case the transducer height should be level with the tragus of the earlobe. If the patient’s head is elevated, the manometer will therefore be higher than the heart.
Volume status can be indirectly assessed by evaluating the arterial pressure height during controlled mechanical ventilation. Positive pressure ventilation will lead to greater systolic variation (>10 mm Hg) of the blood pressure in patients who are hypovolemic ( Figure 10–3 ).

Cardiac Output
Global oxygen delivery to the tissues is dependent on the oxygen content of blood (Ca o 2 ) and cardiac output (CO). Cardiac output is equal to the product of heart rate (HR) and stroke volume (SV):
CO = HR × SV
The variables that affect stroke volume include preload, afterload, and contractility. Preload is an estimate of left ventricular volume at the end of diastole. The Frank-Starling curve shows the relationship between preload and stroke volume ( Figure 10–4 ). In general, increases in preload lead to greater stroke volume. However, a point on the Frank-Starling curve is eventually reached where further increases in preload do not increase stroke volume and may instead lead to decreased stroke volume (as in congestive heart failure). Because it is difficult to measure ventricular volume, ventricular pressure is commonly used to estimate volume and thus preload. Use of a central venous catheter enables monitoring of right atrial pressure or central venous pressure (CVP), which is an estimate of right ventricular preload. In a patient without significant pulmonary hypertension or valvular disease, it can be assumed that right ventricular preload correlates with left ventricular preload because the same blood volume that enters the right heart will traverse the pulmonary circulation to enter the left heart. By way of this assumption, CVP is often used as an estimate of left ventricular preload.

Afterload refers to the myocardial wall tension that is required to overcome the opposing resistance to blood ejection. Right ventricular afterload is indirectly represented by the pulmonary vascular resistance (PVR) and left ventricular afterload is indirectly represented by the systemic vascular resistance (SVR). SVR may be calculated from the following equation if cardiac output has been measured:
SVR = [ ( MAP − CVP / CO ) ] × 80
where MAP = mean arterial pressure.
Contractility refers to the ability of the myocardium to contract and eject blood from the ventricle. Contractility depends on preload and afterload, so these variables should be optimized first to improve contractility. Contractility can be directly measured with the use of echocardiography to estimate ejection fraction. However, once preload and afterload are optimized, contractility is often indirectly represented by cardiac output. If cardiac output remains low despite improvements in preload and afterload, the use of inotropic pharmacological agents may be initiated to improve contractility.
Central Venous Pressure Monitoring
As described above, invasive central venous pressure (CVP) monitoring allows continuous measurement of right heart pressures, which can be used to reflect volume status. The catheter also provides venous access for rapid infusion of fluids or drugs. Normal CVP during positive pressure ventilation ranges from 6 to 12 mm Hg. A low CVP with hypotension and tachycardia usually corresponds to hypovolemia. Persistent hypotension following a fluid challenge and higher than normal CVP usually indicates cardiac congestion (as can occur with cardiac tamponade, tension pneumothorax, or myocardial ischemia). In addition to monitoring of pressures, central venous catheterization enables collection of blood from the superior vena cava (central venous blood) for measurement of central venous blood oxygenation that can be used as an indirect marker of global tissue perfusion and oxygenation.
Indications for Central Venous Catheterization
- ▪
Assessment of volume status
- ▪
Inability to obtain adequate peripheral intravenous access
- ▪
Rapid infusion of fluids through a large cannula (e.g., trauma patients, surgery with significant blood loss)
- ▪
Administration of drugs that may be toxic to peripheral veins (e.g., concentrated vasoactive drugs, hyperalimentation, certain antibiotics)
- ▪
Aspiration of venous air emboli (e.g., sitting craniotomy or other surgery with high-risk of venous air embolism)
- ▪
Blood sampling for frequent laboratory measurements
- ▪
Transvenous cardiac pacing
- ▪
Temporary hemodialysis
- ▪
Introducer for pulmonary artery catheterization
Sites for Central Venous Catheterization
Cannulation sites for CVP placement include subclavian, internal jugular, and femoral veins. Occasionally the external jugular vein may be used if it is large enough. Long-arm CVP catheters can also be passed from the brachial or cephalic vein into the central circulation.
Insertion of a Central Venous Catheter
The Seldinger technique is commonly used to insert a central venous catheter. Figure 10–5 shows the steps to catheterization of the right internal jugular vein with this technique.

To reduce the number of cannulation attempts and the risk of inadvertent arterial puncture, a portable ultrasound vessel-imaging device is often used to visualize the vascular structures as shown in Figure 10–6 .

There are several different kinds of catheters that can be inserted centrally, including single-lumen catheters, multi-lumen catheters, and introducer sheaths. Often a multilumen catheter will be inserted if the catheter is primarily intended for administration of medications and/or CVP monitoring. Introducer sheaths are used when there is the need for a larger catheter, such as for rapid volume administration or for the insertion of a pulmonary artery catheter. When placing an introducer sheath, the use of a tapered-tip, stiff dilator should be placed into the introducer to facilitate passage of this large cannula over the guidewire from the skin, through the subcutaneous tissues, and into the vein. Figure 10–7 shows examples of a multilumen catheter and an introducer catheter.

Complications of Central Venous Catheterization
Contraindications to central venous catheterization include trauma at the site or localized infection. Complications associated with placement of a central line are presented in Table 10–2. To decrease the risk of infection, strict sterile technique should be used. In emergency situations when lines are inserted without adherence to sterile technique, they should be replaced as soon as it is feasible (i.e., when surgery is over and the patient is stable [usually within 12 to 24 hours]).
Central Venous Pressure Waveforms
There are many useful parameters that can be analyzed from the central venous pressure waveform. Table 10–3 lists the components of the CVP waveform and their corresponding phase of the cardiac cycle. Figure 10–8 shows the normal waveform and its components in association with an EKG and arterial pressure waveform. There are also certain physiological abnormalities that are associated with specific changes in the CVP waveform components. Table 10–4 and Figure 10–9 review some of the more common abnormalities and how the CVP waveform is affected.


Pulmonary Artery Catheter
Indications for Pulmonary Artery Catheterization
As described earlier, left heart pressures may be estimated from right heart pressures in most circumstances and CVP may be used to approximate pulmonary capillary wedge pressure (PCWP). However, when left ventricular function is impaired, or significant valvular disease or pulmonary hypertension is present, the use of a pulmonary artery catheter (PAC) may be indicated for more accurate estimations of left heart pressures. Other possible indications for the use of a PAC include severe coronary artery disease, shock, massive trauma, severe lung disease, severe renal disease, and the need to place a temporary ventricular pacing wire via the PAC. Use of a PAC allows continuous monitoring of pulmonary artery pressures, intermittent monitoring of PCWP, and thermodilution for estimation of cardiac output and calculation of systemic vascular resistance. PCWP is used as the best estimation of left ventricular end-diastolic volume (preload), analogous to CVP estimation for the right ventricle.
The PAC can also be used to obtain blood samples for mixed venous oxygen saturation for evaluating the body’s oxygen balance (by calculating oxygen consumption and delivery). Specialized PACs can be used that enable continuous Sv o 2 monitoring.
Complications Associated with Pulmonary Artery Catheterization
Risks associated with PAC placement include those associated with central line placement and additional anatomic and functional disturbances (also see Table 10–2). The risk of complication increases with the duration of catheterization even when optimally placed; therefore, in general a PAC should not persist beyond 72 to 96 hours.
Standard PAC balloons contain latex and there have been reports of severe anaphylactic reactions attributed to the latex. Therefore, latex allergy or sensitivity is a contraindication to the use of a PAC, unless a special nonlatex catheter is used.
It should be noted that the use of a PAC has not been shown to improve survival. In addition, the value of the PAC is dependent on attainment of accurate measurements and the user’s ability to appropriately interpret these measurements.
Insertion of Pulmonary Artery Catheter
The PAC can be placed into any of the central venous cannulation sites that have been described. The procedure begins with the same steps as described above using the Seldinger technique to place a large-bore (7.5 to 9.0 French) introducer sheath into a central vein. This introducer has a hemostasis valve at its outer end, through which the PAC will be inserted, and a sidearm extension that allows continuous central venous access for fluid and drug administration. The final steps in placing a PAC require the aid of a skilled assistant to help prepare the catheter and attach it properly to pressure monitoring transducers. The assistant attaches the distal and proximal port hubs to the pressure monitoring system that will allow waveforms to be displayed on the bedside monitor. All ports should be flushed to remove air from the catheter lumens. The balloon should be tested to make sure there is no leak and that it inflates symmetrically. The balloon should only be inflated with air, not with liquid.
The PAC is inserted through the hemostasis valve of the introducer to a depth of 20 cm, or approximately 5 cm beyond the tip of the introducer sheath. A characteristic CVP waveform must be identified to confirm that the PAC tip is in the vena cava or right atrium. The gentle curvature of the PAC ( Figure 10–10 A) should be oriented to point just leftward of the sagittal plane (the 11-o’clock position as viewed from the patient’s head). This orientation will facilitate catheter passage through the anteromedially located tricuspid valve. Before further insertion of the catheter, the balloon should be inflated, and then the catheter is advanced into the right atrium, through the tricuspid valve into the right ventricle, through the pulmonic valve into the pulmonary artery, and finally into the pulmonary capillary wedge position. Characteristic waveforms from each of these locations confirm proper catheter passage and placement. After the pulmonary capillary wedge pressure is measured, the balloon should be deflated, and the pulmonary artery waveform should reappear for continuous monitoring. PCWP may be obtained periodically as needed by reinflating the balloon with 1.5 mL of air to allow the catheter to float distally until the pulmonary artery occlusion again occurs. Figure 10–10 B shows the characteristic pressure tracings along the path of PAC insertion.

Determination of Cardiac Output Using the Pulmonary Artery Catheter
Cardiac Output by Thermodilution
Cardiac output measurement using a pulmonary artery catheter is based on the principle of thermodilution, in which a known volume of cold fluid is injected into the catheter at a proximal point and the temperature change (cooling effect) is measured in the blood at a downstream point by a thermistor. The temperature change in blood caused by the cold injectate flowing past the thermistor is measured and plotted against time. The shape of the resulting curve is determined by the cardiac output. When cardiac output is low, there will be a larger temperature change because there is a longer delay from the time of injection until the blood reaches the thermistor (more time for cooling effect to occur).
The theory and calculation of cardiac output by thermodilution depend on proper representation of the volume and temperature of the injectate fluid, the thermodynamic properties of blood and injectate solution, and the integral of the temperature-time curve. Both patient and injectate factors can affect the accuracy of thermodilution. Patient factors that can lead to inaccuracy include the presence of tricuspid regurgitation, intracardiac shunts, dysrhythmias, or abnormal respiratory patterns. Injectate factors include errors in injectate volume, rate of injection, and injectate temperature.
Cardiac Output by the Fick Principle
Determination of cardiac output can also be based on the Fick principle, which states that in steady state conditions, the amount of oxygen consumed per unit of time is equal to the arterial oxygen content minus the venous oxygen content multiplied by the blood flow (cardiac output).
In equation form, the Fick principle can be represented by the following equation:
V · O 2 =( CaO 2 − CvO 2 ) × CO

Full access? Get Clinical Tree
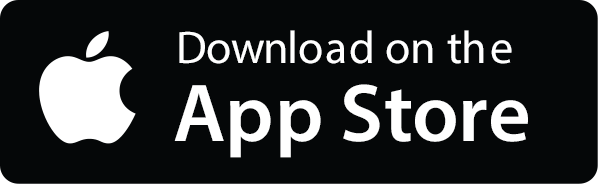
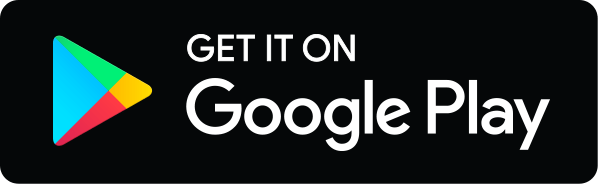