Chapter 9
Intravenous Induction Agents
Nonbarbiturate Intravenous Anesthetics
Chemical Structure and Pharmaceutics
Propofol is a 2, 6-diisopropyl phenol (Figure 9-1). It is prepared as a 1% solution in a lipid emulsion of 10% soybean oil, 2.25% glycerol, and 1.2% purified egg lecithin. The pH of propofol (Diprivan) is 7 to 8.5, and the pKa (negative logarithm of the acid ionization constant) is 11. The pH of the generic form of propofol is 4.5 to 6.4, and the pKa is 11.1 This unique vehicle is especially favorable to bacterial contamination. The original trade product, Diprivan, contains 0.005% disodium edetate (EDTA [ethylenediamine tetraacetic acid]) as a preservative to inhibit bacterial and fungal growth. Generic forms of propofol contain 0.025% sodium metabisulfite or benzyl alcohol depending on the manufacturer. Clusters of infections reported after mishandling of earlier preservative-free preparations prompted the addition of these preservatives.2 Careful handling is still important. It is recommended that aseptic conditions be maintained and the drug be used immediately upon withdrawal from a vial for a single patient. Any remaining drug should be discarded. Syringes or vials should never be used for more than one patient. Opened vials or syringes should be discarded within 12 hours or 6 hours if propofol was transferred from the original container.
FIGURE 9-1 Agents used for the induction of general anesthesia, sedation, and benzodiazepine reversal.
The pharmaceutical preparations are shown in Table 9-1.
Reformulations of Propofol
Newer formulations of propofol are being investigated that will have a more favorable vehicle than the lipid emulsion. These include other soybean and albumin emulsions, micelles and nanoemulsion cyclodextrins among others.1,3
A clear aqueous preparation of propofol as a phosphate prodrug is available for conscious sedation. A prodrug is a drug administered in an inactive or significantly less active form. Once administered, the prodrug is metabolized in the body into the active compound. Although propofol has many advantages such as rapid onset and offset when given for minor procedures such as colonoscopy or bronchoscopy, it can also present some challenges. It has a narrow therapeutic index, and hemodynamic and respiratory depression as well as general anesthesia can easily result from minor dose adjustments. Unlike the benzodiazepines, there is no pharmacologic antagonist. Fospropofol (Lusedra®) is being marketed as a safer formulation for mild to moderate sedation.4 As a prodrug it requires metabolic conversion to active propofol. The resulting slow onset makes it unsuitable for general anesthetic induction. The aqueous preparation avoids the injection pain associated with lipid emulsions. Figure 9-2 depicts the conversion of the prodrug of propofol by plasma alkaline phosphatases to the active propofol molecule, releasing formaldehyde and phosphate. Formaldehyde is further metabolized to formate by formaldehyde dehydrogenase. Select pharmacokinetic and clinical properties of fospropofol are listed in Box 9-1.4–6
Pharmacokinetics
Propofol exhibits a generally favorable kinetic profile, which is one of its main clinical benefits in comparison with other induction drugs (Table 9-2).7,8 Rapid distribution following an IV (intravenous) bolus dose into the brain and other highly perfused areas results in a fast onset of generally one circulation time. Rapid redistribution from the central to the peripheral compartments as the drug more evenly distributes to the entire body produces a quick initial decline in blood levels. As distribution continues, the drug is circulated to less well perfused tissues such as muscle, and the brain concentration falls. This effect leads to a rapid reawakening after sedative and anesthetic doses. The time to awakening is dose and patient dependent but is usually in the range of 5 to 15 minutes. The movement of propofol through various body compartments over time is depicted in Figure 9-3. A visual conceptualization of the redistribution of drugs given by rapid push bolus is shown in Figure 9-4.
Metabolism plays little role in the initial awakening of the patient but is important in the eventual clearance of the drug. An interesting characteristic of propofol is its rapid metabolic clearance, which actually exceeds hepatic blood flow.8 These extra hepatic sites of metabolism probably account for the lack of changes in elimination seen in patients with severe cirrhosis. 9 Less than 1% of propofol is excreted unchanged. It is rapidly metabolized in the liver by CYP2B6, UGTHP4, and CYP2C6.10 The drug’s kinetics are also influenced by age, with the elderly requiring lower doses.11 Children require higher doses because they have an increased volume of distribution based on body weight. Their rate of clearance is also higher.12 Some accumulation can occur with prolonged infusion secondary to tissue saturation.13 The elimination half-life is 1 to 2 hours.
Concerns that the traditional concept of half-life is misleading when drugs are given for prolonged periods by infusion led to the introduction of context-sensitive half time. The context is the duration of the infusion.14 It is the time required for a 50% decrease in plasma concentration after an infusion. Further refinements have described decrement times that include the 20% and 80% declines.15 The decrement time is from the end of the infusion to 50% recovery and includes pharmacokinetic data related to concentration decrease and pharmacodynamic data correlating with recovery. These concepts are discussed in Chapter 6.
Propofol reversibly binds to erythrocytes (50%) and plasma proteins, most commonly to plasma albumin (48%). The free concentration is less than 2%.16 Decreased plasma protein levels resulting from severe renal or hepatic disease and those patients in the third trimester of pregnancy may lower drug binding and increase the free active fraction. This may increase the effects of propofol. Clinically this may be a factor when prolonged infusions are used.17 Factors that can alter protein binding are noted in Table 9-3. Drugs that are highly lipid soluble cross cell membranes rapidly, including the blood-brain and placental barriers. Propofol being highly soluble easily enters all body compartments.18
TABLE 9-3
Factors That Alter Protein Binding
Factors | Percent Bound |
Decreased lipid solubility | Decreases binding |
Increased pH (≤8.0) | Increases binding |
Increased drug concentration | Decreases binding |
Increased protein concentration | Increases binding |
Increased competition for binding sites with other drugs | Decreases binding |
Mechanism of Action
Like many other sedatives and anesthetics, propofol appears to exert its effect via an interaction with the inhibitory neurotransmitter γ-aminobutyric acid (GABA) and the GABAA glycoprotein receptor complex.19 γ-Aminobutyric acid (GABA) is a major inhibitory transmitter in the central nervous system (CNS). The GABAA receptor, which is a ligand-gated ion channel receptor, is activated by the binding of the neurotransmitter GABA. This binding of GABA to the GABAA receptor initiates the movement of chloride (Cl−) through ion channels into the cell. This results in hyperpolarization of postsynaptic cell membrane and the inhibition of neuronal cell excitation. A model has been developed that includes sites of action for GABA on the postsynaptic membrane, as well as sites for numerous other drugs. Propofol directly stimulates GABAA receptors and potentiates the actions of endogenous GABA. The GABA receptor and its function are described in Figure 9-5.
Pharmacodynamics
Central Nervous System Effects
Propofol produces a rapid and pleasant loss of consciousness and emergence from anesthesia. Low-dose infusions for conscious sedation result in dose-dependent anxiolysis, sedation, and amnesia.20 There are dose-dependent reductions in cerebral blood flow (CBF), cerebral metabolic rate of oxygen consumption (CMRO2), intracranial pressure (ICP), and cerebral perfusion pressure(CPP).21 These effects result in part from the decreased mean arterial pressure, depressed metabolic rate, and cerebral vasoconstriction produced by standard doses in a manner comparable to the effects of barbiturates and etomidate.22,23 Cerebral autoregulation and reactivity to changes in carbon dioxide (CO2) are preserved with propofol. Hyperventilation should be applied cautiously in patients with an increase in ICP to avoid hypocarbia-induced cerebral circulatory compromise.24
Electroencephalogram (EEG) data produced a delta rhythm without evidence of epileptiform activity and burst suppression with higher doses.25 Even with these findings, controversy exists over the use of propofol in the patient with epilepsy.26 Three epileptic patients were reported to experience increased epileptiform activity on EEG after the administration of propofol 2 mg/kg.27 Studies in patients with epilepsy showed a different response in that the EEG showed no increase in epileptogenic activity at any of the sites monitored.28 It was also found that activation or extension of EEG activity was greater with thiopental than with propofol (although not statistically significant).29 Propofol has been used successfully to manage status epilepticus.30 Myoclonia induced by propofol results in spontaneous excitatory movements secondary to selective disinhibition of subcortical centers. Adequate dosage may prevent the occurrence of these movements. Myoclonia may occur on induction, but the incidence appears to be lower than that with etomidate. Opisthotonos has also been associated with propofol. Intraocular pressure is decreased by propofol.31 Evidence for a neuroprotective effect is controversial because studies in both animals and humans have not been consistent. Propofol cannot be indicated as a clinical neuroprotectant alone, but it might play an important role in multimodal neuroprotection. Beneficial effects include preservation of cerebral perfusion, temperature control, prevention of infections, and tight glycemic control.32
The use of propofol for electroconvulsive therapy (ECT) has been somewhat controversial as a result of the drug’s effects on seizure duration. Several studies have shown that propofol reduces the duration of the ECT-induced seizure when compared with barbiturates. Evaluating the efficacy of ECT as an antidepressant was typically based on the duration of the seizure; a shortened seizure implied a less effective therapy. However, researchers have found that a reduction in seizure duration does not decrease the efficacy of the ECT, and that propofol is an appropriate agent for use in this procedure.33–35
CNS effects of the intravenous induction drugs are shown in Table 9-4.
Cardiovascular Effects
Propofol usually results in a mild to moderate transient decrease in blood pressure in healthy adults and children during anesthesia induction. The decrease in blood pressure is not usually clinically significant. The effects are more pronounced than those seen with equivalent doses of etomidate or midazolam. It can, however, result in significant hypotension in usual induction doses in select patients. Predictors of hypotension during induction are age greater than 50 years, ASA (American Society of Anesthesiologists) class 3 to 4, baseline mean arterial pressure less than 70 mmHg, and coadministration of high doses of fentanyl. Hypotension usually occurs within 10 minutes after induction and is more prevalent in the second half of the 0- to10-minute interval.36 As with most cardiovascular-depressing sedatives, these effects result from varying degrees of a combination of CNS, cardiac and baroreceptor depression, and decreases in sympathetic tone and systemic vasodilation. The primary reasons for propofol-induced hypotension are decreased sympathetic tone and vasodilation.37 Central nervous system and direct cardiac depression play less of a role. A decrease in dose or alternative agents should be considered in the elderly or cardiac-compromised patients. Propofol has been used successfully for cardiac anesthesia when combined with fentanyl in low-dose or infusion regimens (Table 9-5).38
Respiratory Effects
Transient respiratory depression, more prominent than that seen with etomidate, is common with induction doses of propofol. Decreases in tidal volume are greater than decreases in respiratory rate, although apnea is common on initial administration of induction doses.39 The frequency and duration of apnea are dependent on the dose, speed of injection, patient characteristics, and the presence of other respiratory depressant medications.40 Dose-dependent respiratory depression is also common with maintenance infusions. This appears to result from a decreased sensitivity of the respiratory center to carbon dioxide.41
Propofol has a minimal bronchodilating effect in patients with or without asthma and in patients who smoke. It does not cause histamine release and has been used successfully for anesthesia induction in asthmatic patients. After tracheal intubation, respiratory resistance is lower, and the incidence of wheezing is decreased, compared with the effects of etomidate.42,43 Propofol or ketamine are the preferred induction agents in patients with asthma.44
Other Pharmacologic Actions
Propofol possesses mild antiemetic effects that are most prominent when given by continuous infusion.45,46 The mechanism for the antiemetic effects is unclear. It also exhibits antipruritic effects against opioid-induced pruritus.47,48
Patients experience mild to moderate pain on injection with propofol. The incidence is approximately 60% and is most frequently related to the use of smaller veins for injection The two most effective interventions to reduce pain on injection are the use of the antecubital vein or pretreatment with lidocaine 20 to 40 mg in conjunction with venous occlusion when the hand vein was chosen. Other effective pretreatments are using a lidocaine-propofol admixture, opioids, ketamine, and nonsteroidal antiinflammatory drugs.49 Intraarterial injection of propofol does not cause vascular injury.
Contraindications and Precautions
Few absolute contraindications exist for propofol other than cases in which a known hypersensitivity exists to propofol or its components or the patient has a disorder of lipid metabolism.51,52 New generic formulations of propofol, which contain sodium metabisulfite, should not be used in sensitive patients. The sulfite may cause allergic-type reactions such as anaphylactic symptoms and life-threatening or less severe asthmatic episodes in certain susceptible people. Sulfite sensitivity is more common in patients with asthma than in patients without asthma.53 As noted, caution is advised in elderly, debilitated, and cardiac-compromised patients.
Allergy
Propofol lipid emulsion formulations have been scrutinized because of their lecithin content. Lecithin (from the Greek lekithos, meaning “egg yolk”) is a phospholipid compound composed of a range of phosphatidyl esters such as phosphatidylcholine, phosphatidylethanolamine, and phosphatidylserine. These are combined with varying amounts of triglycerides and fatty acids. Lecithin was originally obtained from eggs, although now soybeans and other vegetables with high lecithin content have also become useful sources. Apart from its use as an antioxidant synergist, lecithin has important surfactant properties and is used as an emulsifying agent.54
Controversy exists regarding whether propofol should be avoided in patients who are allergic to eggs, soy, or peanuts. Egg allergy is most common in children and usually outgrown by adulthood.55 The 2009 guidelines published by the Association of Anesthetists of Great Britain and Ireland claim there is no evidence that propofol should be avoided in egg- or soy-allergic patients.56,57 The warning labels differ among countries for the same formulation of propofol supplied by the same company. The product information for Diprivan 1% warns of its use in egg- or soy-allergic individuals in Australia, soy/peanut (but not egg) in the United Kingdom, and lists no food allergy warnings in the United States. This is despite all three formulations being supplied by the same company.56
A retrospective case review of 28 egg-allergic patients was recently reported. Two of the 28 patients had documented egg anaphylaxis. All but the two children with a history of egg anaphylaxis safely received propofol.58 Data on the two children with documented egg anaphylaxis were not conclusive. The authors suggest propofol is likely to be safe in most egg-allergic children; however, propofol should not be administered to any child with a history of egg anaphylaxis until further evidence is available. Other authors note that there are no confirmed reports of propofol-induced anaphylaxis in egg-allergic patients. They generally demonstrate immediate hypersensitivity to proteins from egg whites. Lecithin is from egg yolks, so there is no reason to contraindicate propofol in egg-allergic patients.59
Soy allergy is a common early onset food allergy in children. Many children outgrow the allergy by age 7.60 Refined soy oil of the types used in propofol have the allergenic proteins removed during refining; therefore it is safe in patients with soy allergy. The suggestion that peanut-allergic patients may exhibit propofol allergy is due to cross reactivity between these leguminous plants; therefore just as with soy allergy, there are also no data to support the avoidance of propofol in patients with peanut allergy.59
Propofol Infusion Syndrome
Propofol is frequently used in adult and pediatric intensive care units for prolonged sedation. Numerous case reports, however, describe findings of various metabolic derangements and organ system failures known collectively as propofol infusion syndrome (PRIS) with long-term high-dose infusions of propofol.61 This has not occurred in anesthesia, even with prolonged administration of relatively high doses, because it seems to require long-duration infusions of increasing doses, as only encountered in critical care units.62 This syndrome is associated with significant morbidity and mortality. A precise mechanism of action of PRIS has yet to be demonstrated.63 Risk factors include young age, doses greater than 4 to 5 mg/kg/hr, duration greater than 48 hours, critical illness, high-fat low-carbohydrate intake, inborn errors of mitochondrial fatty acid oxidation, concomitant catecholamine infusion, and steroid administration. Many of the patients who developed this syndrome have been children, but several cases in adults have been identified as well.
The syndrome has occurred in patients with acute inflammatory disease with infection or sepsis or acute neurologic disease. One common denominator was the presence of impaired systemic microcirculation with tissue hypoperfusion and hypoxia. Propofol seemed to be a triggering agent when catecholamines and corticosteroids are also administered. Fatty acid metabolism and mitochondrial activity are impaired, creating an oxygen supply-and-demand mismatch that results in cardiac and peripheral muscle necrosis. The current theory proposes that propofol inhibits oxidative phosphorylation by (1) inhibition of the transportation of long-chain fatty acids into the cell as well as (2) inhibitory effects on the mitochondrial respiratory chain. Symptoms include severe metabolic acidosis, refractory cardiac failure, persistent bradycardia refractory to treatment, fever, and severe hepatic and renal disturbances.64 The clinical features are noted in Box 9-2. Treatment is supportive and includes discontinuing the propofol, improving gas exchange, cardiac pacing for bradycardia, phosphodiesterase inhibitors, glucagon, extracorporeal membrane oxygenation, and renal replacement therapy.63
Key points regarding propofol are listed in Box 9-3. Induction doses of the intravenous anesthetics are shown in Table 9-6.
Etomidate
Etomidate (1-[1-phenylethyl]-1H-imidazole-5-carboxylic acid ethyl ester) is an intravenous induction agent whose current clinical niche is as an alternative to propofol with little if any cardiorespiratory effects. No intrinsic analgesic properties are associated with the use of this drug.65 Side effects such as pain on injection, myoclonia, nausea and vomiting, and adrenocortical suppression, however, have limited wider acceptance of the drug. Etomidate is a carboxylated imidazole derivative that was synthesized in 1965 and introduced to European anesthesia practice in 1972.66 Etomidate has two isomers, but the (+) isomer is the only one with hypnotic properties (see Figure 9-1 for the chemical structure of etomidate).67
Etomidate is currently supplied as a 2-mg/mL preparation; each milliliter contains 35% propylene glycol as a solvent and has a pH of 8.1 and a pKa of 4.2. This formulation has been changed over the years in an effort to decrease the incidence of pain on injection and spontaneous muscle movements or myoclonia. It is also supplied as a lipid emulsion, Etomidate-Lipuro.68 The lipid emulsion is associated with significantly less injection pain than propofol with added lidocaine in children.69 Previously used preparations included polyethylene glycol, aqueous ethanol, Cremophor EL, and an aqueous solution.70
New formulations are in clinical trials that are designed to maintain the favorable clinical features of etomidate while reducing the primary undesirable effect of prolonged inhibition of adrenal steroidogenesis. Methoxycarbonyl (MOC) etomidate is a “soft” analog that contains a second ester bond distal to the existing etomidate ester linkage.71 MOC etomidate is rapidly metabolized by nonspecific esterase enzymes in blood and tissue to inactive metabolites. The elimination half-life is a few minutes. No adrenal suppression is found 30 minutes after bolus administration because of the rapid elimination.72
Pharmacokinetics
Etomidate is rapidly metabolized in the liver by hepatic microsomal enzymes and plasma esterases. Ester hydrolysis is the primary mode of metabolism in the liver and plasma. Etomidate is hydrolyzed to form inactive carboxylic acid metabolites. Approximately 10% of the administered dose can be recovered in bile, 13% can be recovered in feces, and the remainder of the metabolites are eliminated by the kidney.73
The rapid redistribution of etomidate accounts for its extremely short duration of action (see Table 9-2). The drug is lipid soluble and has a volume of distribution that is several times greater than its body weight. Shortly after intravenous injection (within 1 minute), the brain concentration rises rapidly because of the drug’s lipid solubility, and over the next several minutes extensive redistribution to other organs and tissues occurs and the patient regains consciousness.
The total body clearance of etomidate is rapid. The hepatic extraction ratio is 67%. Studies examining dose-response relationships have found a lack of accumulation with this compound.65 The terminal half-life is 2 to 5 hours.72
The mechanism of action of etomidate, like many other CNS depressants, involves GABA modulation (see Figure 9-5).74 In a clinical investigation of 2500 cases, Doenicke et al.75 confirmed that no histamine is released by etomidate.
Pharmacodynamics
Central Nervous System Effects
Etomidate produces a dose-dependent CNS depression within one arm-brain circulation. Its duration of action is also dose dependent,65 with awakening occurring 5 to 15 minutes after a 0.2- to 0.4-mg/kg dose. The drug is devoid of analgesic properties.
Cerebral blood flow and cerebral metabolic rate of oxygen consumption are both decreased by etomidate.76,77 In a study of fully alert patients without neurologic deficit or impaired consciousness, an etomidate induction was followed by an infusion of 2 to 3 mg/min. Cerebral blood flow decreased 34%, and cerebral metabolism was also reduced (mean decrease of 45%). Decreased oxygen consumption and the associated decrease in carbon dioxide production can cause cerebrovascular vasoconstriction, decreased cerebral blood flow, and decreased intracranial pressure. Also noted during this trial was the maintenance of cerebral blood vessel responsiveness to changes in carbon dioxide levels.76
In a study of patients with intracranial pathology, etomidate (0.2 mg/kg given intravenously) was shown to decrease intracranial pressure while maintaining cerebral perfusion pressure. Because of the cardiovascular stability of this drug, mean arterial pressure did not decrease below cerebral autoregulation values at which cerebral blood flow would become pressure dependent. Cerebral perfusion pressure was maintained adequately in all study subjects.77
The electroencephalographic changes that follow administration of etomidate are similar to those that follow administration of other intravenous induction anesthetics. When compared with thiopental, a lack of beta-wave activity was present during induction, along with a longer duration of stages III and IV.78
One negative characteristic of etomidate is its excitatory phenomenon of muscle movements and tremors.78,79 Referred to as myoclonia, this phenomenon is defined as sudden, generalized, asynchronous muscle contractions.80 Myoclonia can affect many muscle groups or a single muscle. The movements can be so severe that they resemble, and are often mistaken for, seizures. In EEG patterns monitored during etomidate anesthesia, no specific EEG disturbances occurred during or after myoclonic episodes.78 The origin of these muscle movements is thought to be related to uneven drug distribution into the brainstem or deep cerebral structures and not to CNS stimulation.78,81 The incidence of myoclonia ranges between 10% and 60% and varies with the type and the amount of premedication given. Investigators associated the 35% incidence of myoclonic movement with painful stimuli (e.g., drug injection, mandibular lifting). Horrigan et al.79 reported that premedication with fentanyl (100 mg) given intravenously 2 minutes before induction did not significantly decrease motor activity. However, pretreatment with small doses of etomidate, dexmedetomidine, midazolam, rocuronium, and lidocaine are all effective in reducing myoclonia.82–86 Etomidate is shown to decrease intraocular pressure.
Electroencephalographic changes with etomidate are similar to those of other intravenous induction drugs. Bispectral index (BIS) monitor values decrease after a bolus of etomidate and correlate well with sedation scores. Etomidate decreases the amplitude and increases latency of auditory evoked potentials. The duration of epileptiform activity after ECT is longer after induction with etomidate versus propofol. Somatosensory-evoked potential amplitudes are enhanced by etomidate and motor-evoked potential amplitudes are suppressed less by etomidate than propofol.87–89
Cardiovascular Effects
The primary clinical advantage of etomidate over propofol is the hemodynamic stability upon induction in healthy or modestly debilitated patients. In patients with compensated heart disease, changes in heart rate, pulmonary artery pressure, cardiac index, systemic vascular resistance, and systemic blood pressure were not significant.90 In one study of high-risk patients with significant cardiac disease, hemodynamic stability was maintained with induction doses of 0.3 mg/kg. Also, minimal changes in heart rate, blood pressure, central venous pressure, and intrapulmonary shunting have been demonstrated after etomidate administration.91 Patients with aortic and mitral valve disease, however, are noted to have significant decreases in systemic blood pressure (17% to 19%), pulmonary artery pressure (11%), and pulmonary capillary wedge pressure (17%).92 Slight decreases in blood pressure are thought to be caused by decreases in systemic vascular resistance. The hemodynamic stability seen with etomidate has been attributed to a unique lack of depression of sympathetic nervous system and baroreceptor function.93 Myocardial oxygen supply and demand are kept constant by a balance of decreased myocardial blood flow and decreased oxygen consumption.94 No significant cardiac dysrhythmias are associated with etomidate administration. Both renal and hepatic blood flows are maintained by the stability of cardiac output (see Table 9-5). In summary, at equivalent anesthetic doses, propofol depresses cardiorespiratory function to the greatest degree and etomidate depresses it the least.
Respiratory Effects
Etomidate affects the respiratory system in a dose-dependent manner. Minute volume decreases, but respiratory rate increases. The respiratory depression seen with propofol use is significantly greater than that seen with etomidate.94 The ventilatory response to carbon dioxide is decreased, and etomidate administration may cause brief periods of apnea following induction. Etomidate has little effect on bronchial tone and does not cause histamine release (see Table 9-5).95,96
Adrenocortical Effects
Adrenal cortical suppression by etomidate has been widely studied, and this effect significantly limits it clinical use. It is useful as a single-dose anesthesia induction agent, but infusions are no longer used. The issue elicited widespread concern after 10 years of use in Europe. Researchers found an increased mortality rate in critically ill patients who received etomidate infusions. This phenomenon was attributed to adrenocortical hypofunction, demonstrated by decreased levels of plasma cortisol.97 Multiple studies have shown adrenal hormone levels to be decreased for up to 8 to 24 hours after a single induction dose or more than 24 hours with infusion.98–104 These effects are caused by a reversible dose-dependent inhibition of adrenal steroidogenesis. The enzymes inhibited are the cytochrome P-450–dependent mitochondrial enzymes and 11β-hydroxylase. To a lesser degree, 17α- and 18-hydroxylases are also affected. This results in an increase in cortisol precursors but a decrease in cortisol, aldosterone, and corticosterone levels. This enzyme inhibition results in decreased ascorbic acid synthesis, which is necessary for steroid production.105 In summary, these effects are primarily caused by a reversible dose-dependent inhibition of the adrenocortical enzyme 11β-hydroxylase. The mechanism for the adrenocortical hypofunction following administration of etomidate is shown in Figure 9-6.
The clinical significance of this pharmacologic effect continues to be discussed. There have been several reports of single doses of etomidate resulting in patient morbidity. Critically ill patients given corticosteroid therapy for septic shock had poorer results when etomidate was also administered.106 A large-scale follow-up study (CORTICUS) evaluating low-dose corticosteroid therapy in septic shock suggested that patients receiving etomidate before enrollment had a 28-day mortality, significantly higher than other patients in the trial, and that steroids provided no benefit to those who received etomidate.107,108 A further analysis of the data led the authors to report that the use of a bolus dose of etomidate in the 72 hours before inclusion in the study was associated with an increased incidence of inadequate response to corticotropin and an increase in mortality. They recommend that clinicians exercise caution in the use of etomidate in critically ill patients with septic shock.108 The duration of adrenal insufficiency after single-dose etomidate and its effect on outcomes in this population is longer than 24 hours and may last up to 72 hours.109 The administration of supplemental steroids to counter this effect is controversial. It did not appear effective in septic patients108; however, others suggest that the empirical use of steroid supplementation for 48 hours after a single dose of etomidate in critical patients without septic shock should be considered.109

Full access? Get Clinical Tree
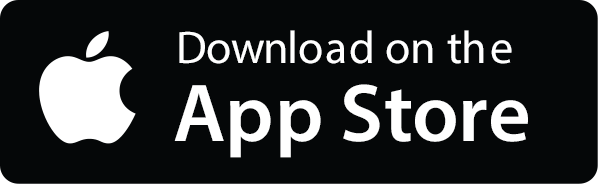
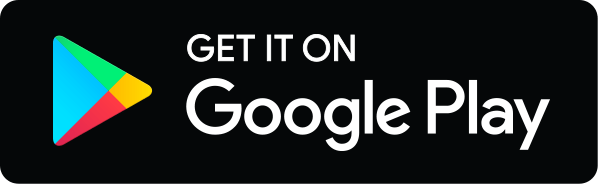
