KEY POINTS
1. Mechanisms of myocardial ischemic injury are multifactorial and include some of the following: Depletion of high-energy phosphates, intracellular acidosis, alterations in intracellular calcium homeostasis, complement activation, generation of oxygen-free radicals upon reperfusion, and myocardial edema.
2. Myocardial stunning represents viable myocardium that has systolic and/or diastolic dysfunction in the presence of normal myocardial perfusion.
3. Hibernating myocardium is viable myocardium that is chronically underperfused and subsequently has downregulated its contractile elements.
4. The ultimate measure of improved myocardial protection is improved survival or lessening of low-output syndrome (LOS). While LOS has declined in frequency over the decades, its prognosis has worsened with a high mortality associated with LOS.
5. The purpose of cardioplegia in addition to preservation of cardiac function is to provide cardiac quiescence and a bloodless operative field.
6. Diastolic arrest with potassium-rich solution and hypothermia are the mainstays of cardioplegic protection.
7. Although benefits to blood cardioplegia may include improved systolic functional recovery, decreased ischemic injury, and decreased myocardial anaerobic metabolism, it appears that there is no difference in operative mortality or long-term ventricular function when compared to crystalloid cardioplegia. However, most operative centers in the United States use blood cardioplegia.
8. Cardioplegia can be delivered anterograde through the aorta or coronary ostia or retrograde through the coronary sinus. There are advantages and disadvantages to each and both are often combined in the same procedure.
I. Introduction
Cardiac surgery is performed to preserve or restore cardiac function in a diseased heart. However, the performance of the procedure is, by necessity, accompanied by myocardial injury. This injury occurs when there is a significant imbalance between myocardial oxygen delivery and energy requirements. In the vast majority of cases, perioperative myocardial injury is minimal and is well tolerated. However, severe perioperative myocardial dysfunction is often lethal. Unlike the situation of a myocardial infarction, ischemia during cardiac surgery is usually planned, global in nature, and starts with the application of the aortic cross-clamp or some other intentional maneuver. The ischemic period may be continuous or intermittent, depending on the conduct of the operation.
Adequate myocardial protection can minimize the detrimental effects of the operation on the heart and can allow the heart to tolerate the prolonged periods of planned ischemia that are often necessary to conduct the operation. A proper strategy of myocardial protection encompasses events before, during, and after the initiation of planned myocardial ischemia, including treatment of the patient both preoperatively and postoperatively. As such, all medical personnel involved in the perioperative care of the cardiac patient should be cognizant of the implications of their actions toward myocardial preservation. A strategy of myocardial protection may be made infinitely complex, but most patients will be adequately served through the use of a limited number of techniques and agents designed to minimize the difference between oxygen delivery and utilization during ischemia. Beating heart surgery requires different strategies for myocardial protection, but they remain based on the balance between the supply and demand of oxygen. In addition, the use of minimally invasive techniques, such as port access, has increased the role of the anesthesiologist in the perioperative aspects of myocardial protection.
II. History of myocardial protection
A. Before the introduction of the cardiopulmonary bypass machine, the earliest cardiac procedures were performed without myocardial protection. Topical cooling and systemic hypothermia were used to facilitate the conduct of the operation with relatively little regard to the metabolic needs of the heart.
B. In 1955, Melrose advocated the use of hyperkalemic cardioplegia to provide cardiac quiescence during the operation. This approach was later abandoned because of the permanent myocardial injury (“stone heart”) produced by the high potassium concentrations used.
C. In 1956, Lillehei introduced the technique of administration of cardioplegia through the right atrium or coronary sinus (“retrograde cardioplegia”) for use in operations on the aortic valve.
D. Procedures used to provide myocardial standstill, such as intermittent aortic cross-clamping, were primarily performed to facilitate the conduct of the operation, with less regard to the ischemic consequences to the myocardium. Interest and research in chemical cardioplegia persisted but was not used clinically.
E. Topical myocardial cooling with iced saline or slush was used as an early form of myocardial protection once it was possible to support the circulation with cardiopulmonary bypass.
F. A correlation was made between poor myocardial protection and the development of myocardial necrosis in patients who succumbed to postoperative cardiogenic shock. Clinical confirmation was obtained when a high rate of perioperative myocardial infarction was demonstrated in patients undergoing either coronary artery bypass grafting (CABG) or valvular procedures.
G. In 1973, Gay and Ebert reintroduced hyperkalemic cardiac arrest but with potassium concentrations less than 20 mmol, thus preventing the occurrence of stone heart syndrome seen with the earlier use of potassium cardioplegia. This marked the beginning of the use of a technique that provided a combination of effective myocardial protection and cardiac quiescence.
H. In the late 1970s, Follette et al. [1] and Buckberg popularized the use of blood cardioplegia, citing the advantages gained by using the intrinsic characteristics of potassium-enriched blood, such as its natural buffers and oxygen delivery capabilities.
III. Cardiac physiology
A. The myocardium has a high rate of energy consumption under normal circumstances. This requires a constant supply of oxygen to the myocardium. Myocardial ischemia occurs when the supply of oxygen is exceeded by the demand, and infarction occurs when this occurs for a prolonged period of time.
B. Oxygen delivery to the myocardium is dependent upon the concentration of hemoglobin in the blood, the arterial oxygen saturation, and the flow of oxygenated blood to the myocardium. Under normal conditions, the blood flow to the myocardium is controlled by autoregulation, in which blood flow is matched to myocardial requirements. Autoregulation is not active when the blood pressure is above or below the autoregulatory range (roughly 60 to 180 mm Hg).
C. The subendocardium receives its nutritive flow primarily during diastole and is vulnerable to variations in blood flow.
1. Flow is dependent upon the transmural gradient, which is the difference between the aortic diastolic pressure and the intraventricular end-diastolic pressure.
2. Oxygen delivery may be insufficient because of either a decrease in perfusion pressure (systemic hypotension or coronary artery disease) or an increase in ventricular end-diastolic pressure (aortic stenosis, ventricular fibrillation, or ventricular distension).
3. The right ventricle may be less susceptible to injury since its subendocardium receives nutritive flow throughout the cardiac cycle as a consequence of RV systolic pressures well below systemic levels, with the exception of instances of severe pulmonary hypertension.
D. The heart depends upon a continuous supply of oxygen to maintain full function.
1. Adenosine triphosphate (ATP) is generated at a rate of 36 moles per mole of glucose in the presence of oxygen.
2. Under anaerobic conditions, ATP production falls to 2 moles of ATP per mole of glucose. Lactate and hydrogen accumulate in the tissues, which further inhibit glycolysis and other cellular functions.
E. Myocardial oxygen consumption is dependent upon the work performed by the heart (Fig. 23.1) [2,3].
Figure 23.1 LV myocardial oxygen uptake of the beating empty, fibrillating, and arrested perfused hearts at varying myocardial temperatures. The greatest decrease in myocardial oxygen uptake at a given temperature occurs with mechanical arrest. The addition of hypothermia decreases the oxygen consumption to a lesser degree. (From Buckberg GD, Brazier JR, Nelson RL, et al. Studies of the effects of hypothermia on regional myocardial blood flow and metabolism during cardiopulmonary bypass. I. The adequately perfused beating, fibrillating, and arrested heart. J Thorac Cardiovasc Surg. 1977;73:87–94, with permission.)
1. Normal working ventricular myocardium consumes 8 mL of O2 per 100 g of myocardium per minute.
2. This decreases to 5.6 mL of O2 per 100 g of myocardium in the empty beating heart and to 1.1 mL of O2 per 100 g of myocardium per minute in the potassium-arrested heart.
3. Myocardial cooling provides an additional decrease to 0.3 mL of O2 per 100 g of myocardium.
1
IV. Mechanisms of myocardial ischemic injury.
The mechanisms by which ischemia and reperfusion injure the heart are complex, and the contributions of the individual components to this process are hotly debated. It is possible that the process of reperfusion may be equally as harmful to the myocardium as the ischemic insult itself. Thus, in the field of myocardial protection, it is important to have an understanding of the components thought to contribute to the damage. Strategies to ameliorate these factors may lessen the injury, whereas ignoring important aspects of ischemia–reperfusion injury may make all other protective interventions ineffective.
A. Depletion of high-energy phosphates occurs during ischemia. Breakdown products may be washed away with reperfusion, prohibiting the rapid conversion back to ATP once perfusion is restored.
B. Intracellular acidosis develops during anaerobic metabolism, and the accumulation of hydrogen ions interferes with the function of many intracellular enzymes.
C. Calcium is important in numerous cellular functions. The intracellular fluxes in calcium concentration are responsible, in part, for contraction and relaxation of the myocardium. Alterations in intracellular calcium homeostasis have been documented after ischemia and reperfusion. Changes in the rate of calcium uptake or release within the cell can have profound functional consequence and injured ischemic myocardium rapidly becomes calcium overloaded.
D. Direct myocellular injury from ischemia may cause myocardial dysfunction.
E. In addition to the alterations in calcium homeostasis that have been documented, intracellular calcium overload can occur at the time of reperfusion as calcium is released from the sarcoplasmic reticulum or enters the cell through calcium channels, such as the sodium–calcium exchanger or the L-type calcium channel. Alterations in intracellular calcium levels may activate enzymes, trigger second messenger cascades, or alter excitation–contraction coupling. Calcium concentrations may be so high that contracture develops. In the presence of ischemia, ATP necessary for extrusion of sodium is absent and cells become sodium loaded which leads to intracellular calcium loading through the sodium–calcium exchanger.
F. Generation of oxygen-derived free radicals occurs upon reperfusion. These are highly unstable compounds that are capable of damaging proteins, nucleic acids, phospholipids, and other cellular components. Natural free-radical scavengers prevent damage under normal circumstances, but these endogenous systems are depleted during a significant period of ischemia and are quickly overwhelmed.
G. Complement activation may occur as part of the generalized inflammatory process that occurs with injury.
H. Adverse endothelial cell–leukocyte interactions occur after ischemia and reperfusion. Under normal conditions, the endothelium and neutrophils are the producers of, and responsive to, numerous signaling compounds. There is a delicate balance between vasoconstriction and vasodilation, as well as between the promotion and prevention of thrombosis. Adenosine, nitric oxide, endothelin, and thromboxane are a few of the potent substances whose production and effects are altered after ischemia and reperfusion. This may produce a state of altered endothelial cell–leukocyte interaction, leading to areas of myocardial malperfusion and damage from increased endothelial adherence [4].
I. Myocellular edema may result from ischemia–reperfusion injury. Edema may occur in response to numerous injurious events and can alter the function of all of the cells within the myocardium. Edema has been implicated in contractile dysfunction, decreased ventricular compliance, and capillary plugging that inhibits reperfusion of the coronary microcirculation.
J. Damage to non-myocyte components of the heart may cause systolic and diastolic dysfunction. This includes injury to the endothelium of the coronary circulation, as well as to fibroblasts and other structural components of the heart.
V. Consequences of ischemia–reperfusion injury.
The severity of myocardial injury after a period of ischemia and reperfusion depends on numerous factors, including the length of ischemia (Fig. 23.2) [5], the temperature of the myocardium, the conditions of the myocardium before, during, and after the ischemia, and the method in which the myocardium is reperfused. The resulting myocardial injury can be described according to the established criteria.
Figure 23.2 Relationship among myocardial temperature, duration of ischemia, and recovery of aortic blood flow. Two sets of experiments are shown. In the duration of ischemia studies (top horizontal axis, blue line), the heart was made ischemic for varying times at 30°C and then reperfused. The percentage myocardial recovery is shown on the vertical axis, and it declines steadily with time. In the temperature studies (lower horizontal axis, red line), hearts were subjected to 60 min of ischemia at varying myocardial temperatures and then reperfused. As the temperature of the myocardium is decreased below 28°C, myocardial recovery increases. (From Hearse DJ, Stewart DA, Braimbridge MV. Cellular protection during myocardial ischemia. The development and characterization of a procedure for the induction of reversible ischemic arrest. Circulation. 1976;54:193–202, with permission from Lippincott Williams & Wilkins.)
2
A. Brief periods of ischemia may produce no readily identifiable functional deficit.
3
B. Myocardial stunning represents ischemia–reperfusion injury in its mildest form. Although stunning may be severe, it represents viable myocardium that has systolic and/or diastolic dysfunction in the presence of normal myocardial perfusion. The etiology of the functional changes seen in stunning is multifactorial and likely includes altered calcium handling, cellular edema, and other factors. By definition, there is no necrosis in stunned myocardium. Given sufficient time, stunned myocardium will manifest complete functional recovery in the absence of additional injury. Stunned myocardium is distinct from hibernating myocardium, which is viable myocardium that is chronically underperfused and subsequently has downregulated its contractile elements. Upon revascularization, hibernating myocardium begins to return to its normal phenotype and subsequently returns to normal function.
C. Myocardial necrosis occurs when myocytes are irreversibly injured. Necrosis may not be readily identifiable by functional or histologic means early after injury and thus may not be distinguishable from stunned myocardium at this early time point. However, these cells eventually die despite reperfusion and are replaced by a noncontractile scar.
4
VI. Measuring success.
Myocardial protection in cardiac surgery has advanced a long way since the first cardiac operations were performed, and morbidity and mortality rates have dropped significantly. The ultimate measure of improved myocardial protection is improved survival or a lessening in the occurrence of “low-output syndrome” (LOS). While the occurrence of LOS has declined in frequency over the decades, its prognosis has worsened with a higher mortality rate associated with LOS [6]. Since mortality and LOS are rather uncommon in elective cardiac cases today and the incremental benefit of a new protection strategy is likely to be small, randomized trials to demonstrate a benefit in terms of survival or LOS will have to be large. Due to this impracticality, many current trials use surrogate endpoints such as lowered levels of cardiac enzymes or shorter ICU stays. However, the clinical significance of these endpoints is less well defined. Importantly, many agents touted to improve myocardial protection have shown remarkable results in animal studies only to fail to achieve significance in humans. Thus, it is important to determine if a protective strategy has a clinically meaningful endpoint in humans, and to ensure that not too much weight is given to results from animal studies, regardless of the degree of benefit seen.
5
VII. Purpose of cardioplegia.
Historically, most cardiac operations performed over the past few decades have been done under conditions of cardiac arrest. Despite the availability of beating heart surgery techniques, cardiac arrest is still predominantly used during valve repair/replacement, cardiac transplantation, procedures on the aortic root, and most cases of cardiac revascularization. Cardioplegia serves separate, but often interrelated, purposes.
A. Cardiac quiescence facilitates most cardiac procedures as they are more easily performed on the flaccid, noncontracting heart than on the beating heart. Additionally, this lessens the possibility of air embolism occurring during open procedures performed on the left-sided chambers of the heart. Although cardiac standstill was originally produced via cooling of the heart, potassium-based cardioplegia can provide rapid and reversible arrest.
B. Interruption of myocardial blood flow facilitates the operation by providing a bloodless field, enhancing visibility. During most cardiac procedures, a cross-clamp is applied across the ascending aorta, which eliminates continuous coronary blood flow. Through the use of cardioplegia, the energy requirements of the myocardium can be significantly reduced, thus increasing the safety and allowable duration of this interruption of blood flow.
C. Through the reduction in myocardial energy consumption produced by electromechanical arrest, there is preservation of myocardial function, despite significant periods of myocardial ischemia.
D. Current methods of cardioplegic myocardial arrest allow for the rapid resumption of contractile activity at the end of the procedure. The period of contractile arrest can be lengthened by the administration of additional doses of cardioplegia, and it can be shortened by the restoration of myocardial blood flow with washout of the cardioplegia. This control allows the surgeon to minimize the time of cardiopulmonary bypass awaiting the return of cardiac function while providing maximal myocardial protection during the performance of the procedure.
VIII. Interventions before the onset of ischemia (aortic cross-clamping).
Optimal myocardial protection requires a complete, well-conceived strategy that takes into consideration the unique characteristics of the individual patient. While it may be possible to treat every patient the same, a tailored approach is more likely to minimize cardiac damage and thus mortality. Aortic insufficiency, ventricular hypertrophy, and severe obstructive coronary disease are a few of the factors that may alter the intraoperative management in order to obtain maximal cardiac protection. As a part of planning ahead, there are several interventions before the onset of global cardiac ischemia (aortic cross-clamping) that influence the effectiveness of myocardial protection. Almost all aspects of myocardial protection involve maintaining balance between myocardial oxygen supply and demand.
A. Minimization of ongoing ischemia may require the use of nitrates, anticoagulants, antiplatelet agents, or insertion of an intra-aortic balloon pump in the preoperative period. Since there may be asymptomatic cardiac ischemia at baseline, hypertension, tachycardia, and patient anxiety should be controlled. Supplemental oxygen should be used liberally.
B. Perioperative b-blockade has been shown to decrease cardiac-related mortality in most patients undergoing surgical revascularization, although there may be a slight increase in mortality in those patients with an ejection fraction less than 30% who are treated with preoperative β-blockers. Unless contraindicated, preoperative β-blockers should be given in patients undergoing CABG surgery in the absence of severe depression of left ventricular (LV) function.
C. Rapid revascularization. Any sign of ischemia should warrant aggressive diagnosis and management. If ischemia cannot be readily controlled and infarction is imminent, then emergent operation to alleviate ischemia is required unless otherwise contraindicated. This requires the active involvement of all members of the surgical team so that the operation can proceed without delay. In this setting, rapid reversal of ischemia may require the use of saphenous vein grafts as opposed to arterial conduits if the harvesting of the internal mammary artery would unduly delay resolution of the ischemia. Similarly, the choice between on-pump and beating heart revascularization may depend upon the urgency of the operation and stability of the patient.
D. Nutritional repletion may be possible in the setting of elective cardiac surgery. The depleted heart has little reserve and may not tolerate ischemia well. Depressed glycogen levels have been correlated with poor postoperative outcomes, and repletion with the preoperative administration of GIK solution (glucose, insulin, potassium) [7] has been demonstrated to reduce complications and improve early postoperative cardiac function.
E. Avoidance of ischemia. Although some regional myocardial ischemia is required during any bypass operation, the intraoperative global cardiac ischemia that accompanies aortic cross-clamping may be avoided through one of several mechanisms.
1. CABG may be performed using beating heart techniques. This strategy allows continuous perfusion of the coronary vasculature, with the exception of the territory being bypassed while the anastomosis is being performed. With the use of an intraluminal flow-through device (shunt), some blood flow can be maintained to the distal vessel during the creation of the anastomosis, except for brief periods of time. However, perfusion pressure must be maintained within the physiologic range to avoid cardiac and peripheral ischemia. Insertion of an intra-aortic balloon pump may be useful in this setting if hemodynamic instability results from cardiac manipulation.
2. Bypass grafting may be performed with minimal cardiac ischemia through the use of cardiopulmonary bypass without aortic cross-clamping. In the setting of the empty beating heart, perfusion to both the body and coronary arteries is supported by the bypass machine, eliminating the hemodynamic instability and subsequent hypoperfusion that may be associated with cardiac manipulation in some off-pump patients. Although this technique does not avoid the systemic effects of extracorporeal perfusion, it eliminates the effects of systemic hypothermia and avoids the myocardial effects of cardioplegia. By eliminating the work performed by the heart, myocardial energy requirements may drop by 20% to 30% of those in the working heart.
3. Intracardiac procedures may be performed without significant cardiac ischemia with the patient on bypass, without aortic cross-clamping. Although visibility may be limited, procedures such as tricuspid or mitral valve repair or replacement, or atrial or ventricular septal defect repair may be performed with continuous coronary perfusion. On procedures of the left heart, the heart is often briefly fibrillated while the chambers are opened. The LV must remain decompressed to avoid the ejection of air through the aortic valve and subsequent air embolization.
F. Fibrillatory arrest creates a nearly motionless heart to allow the performance of many cardiac procedures. It may be produced through either electrical stimulation or myocardial cooling.
1. Normothermic fibrillatory arrest is produced by placing an alternating current generator in contact with the ventricular myocardium. As long as contact is maintained, the ventricle will remain in fibrillation, allowing procedures to be performed upon the heart with little ventricular motion. In this state, the left side of the heart can be opened to allow procedures such as closure of an atrial septal defect, without the fear of ejecting air into the arterial circulation.
a. Since the myocardium remains warm and the heart is essentially in a continuous state of contraction, energy consumption remains high.
b. The fibrillating myocardium has increased wall tension since it is in a state of continuous systole.
c. Since perfusion to the endocardium occurs primarily during diastole, endocardial perfusion is compromised, thus allowing possible subendocardial infarction.
d. Thus, it is impossible to produce a balance between oxygen supply and demand. Since myocardial ischemia is the inevitable result, this technique is not recommended.
2. Hypothermic fibrillatory arrest [8] occurs as the myocardial temperature falls when the body is cooled. Energy consumption of the myocardium is less than during warm ventricular fibrillation, but not as low as during complete arrest. Procedures such as CABG or mitral valve replacement may be performed without interruption of myocardial perfusion in the cold fibrillating heart. Fibrillation should be avoided in the setting of ventricular hypertrophy (Fig. 23.3) [9] or significant aortic insufficiency (see “Prevention of ventricular distention”).
Figure 23.3 LV myocardial flow distribution in the normal and hypertrophied heart. In LV hypertrophy, endocardial blood flow is compromised during fibrillation, as the ratio of endocardial to epicardial flow decreases. This does not occur in the normal ventricle. (From Hottenrott CE, Towers B, Kurkji HJ, et al. The hazard of ventricular fibrillation in hypertrophied ventricles during cardiopulmonary bypass. J Thorac Cardiovasc Surg. 1973;66:742–753, with permission.)

Full access? Get Clinical Tree
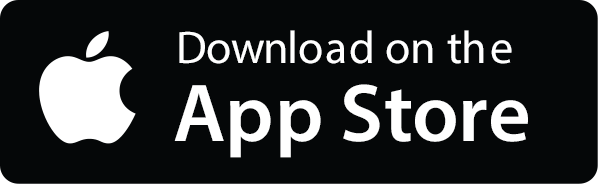
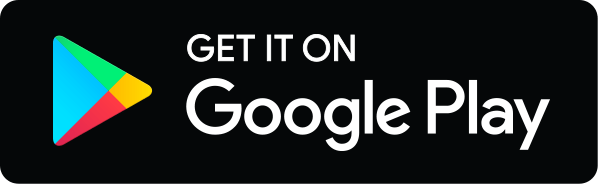