Questions
- 1.
What is cerebral autoregulation?
- 2.
What factors contribute to increased intracranial pressure?
- 3.
How do anesthetic agents and vasoactive drugs affect cerebral blood flow and intracranial pressure?
- 4.
What are the signs and symptoms of increased intracranial pressure?
- 5.
How is intracranial pressure monitored?
- 6.
How is increased intracranial pressure treated?
- 7.
How is venous air embolism detected and treated?
- 8.
What are contraindications to the sitting position?
- 9.
How would you induce and maintain anesthesia in this patient?
- 10.
A 63-year-old woman with a brain tumor presents for bifrontal craniotomy in the supine position. She is neurologically intact but recently experienced headaches, nausea, and blurred vision. Magnetic resonance imaging (MRI) revealed a large meningioma near the sagittal sinus. After induction of anesthesia and the start of surgery, the surgeon begins drilling a burr hole in the skull. End-tidal carbon dioxide (ETCO 2 ) starts to decrease within 5 minutes, and the patient becomes hypotensive.
1
What is cerebral autoregulation?
Cerebral autoregulation is the control process by which cerebral blood flow (CBF) is maintained constant over a wide range of cerebral perfusion pressure (CPP) ( Figure 14-1 ). CPP represents the difference between mean arterial pressure (MAP) and intracranial pressure (ICP). Autoregulation adjusts cerebral vessel caliber as CPP changes. Normal CBF is 45–65 mL/100 g of brain tissue per minute. It is coupled to alterations in cerebral metabolic rate, which is linked to oxygen consumption (CMRO 2 ). CBF parallels the changes in CMRO 2 . Several parameters affect CBF ( Box 14-1 ).

Cerebral autoregulation
CPP
Cerebral metabolic rate
PaCO 2
PaO 2
CPP, Cerebral perfusion pressure; PaCO 2 , arterial carbon dioxide tension; PaO 2 , arterial oxygen tension.
Autoregulation maintains CBF at a CPP of approximately 50–150 mm Hg. At CPP <50 mm Hg, cerebral blood vessels achieve maximal dilation (i.e., resistance to flow is low), and CBF decreases in direct proportion to CPP. Chronically hypertensive patients undergo an upward shift of autoregulation to higher perfusion pressures. Consequently, these patients require higher CPP to maintain normal CBF. At the upper level of autoregulation, cerebral blood vessels are maximally constricted (i.e., resistance to flow is high), and CBF increases linearly with increasing CPP. Integrity of the blood-brain barrier (BBB) is lost at these high pressures, and transudation of fluid occurs resulting in cerebral edema. Autoregulatory compensation generally occurs over 1–3 minutes.
The second parameter affecting CBF is arterial carbon dioxide tension (PaCO 2 ). Increasing levels of PaCO 2 produce elevated levels of extracellular hydrogen ions, which induce cerebral vessel smooth muscle relaxation and vasodilation. Consequently, cerebral vascular resistance decreases, increasing CBF by twofold. This effect plateaus at a PaCO 2 of approximately 80 mm Hg. Conversely, decreasing PaCO 2 increases cerebral vasoconstriction, and CBF decreases. At a PaCO 2 of 20 mm Hg, cerebral vasoconstriction is maximal, and CBF decreases by 50%. Further decreases in PaCO 2 have no greater vacoconstricting influence. These physiologic changes remain in effect for several hours, after which cerebrospinal fluid (CSF) bicarbonate levels decrease to compensate for induced CSF alkalosis. When CSF pH returns toward normal, respiratory alkalosis no longer provokes cerebral vasoconstriction. The PaCO 2 response at the limits of autoregulation can be blunted. If CPP is low and cerebral vessels are maximally dilated, lowering PaCO 2 would have little beneficial effect on cerebrovascular resistance.
The third parameter affecting CBF is arterial oxygen tension (PaO 2 ). At a PaO 2 <50 mm Hg, CBF increases linearly with decreasing PaO 2 . Local accumulation of acidic metabolites (e.g., lactate) results in cerebral vasodilation. In contradistinction, hyperoxia has no effect on cerebrovascular tone.
2
What factors contribute to increased intracranial pressure?
The cranial vault contains brain tissue, blood, and CSF. It has a fixed volume with one exit, the foramen magnum. A small increase in the contents of the cranial vault alters intracranial pressure (ICP) minimally because compensation occurs by decreasing CSF and blood volumes. However, when compensatory mechanisms have been exhausted, intracranial compliance decreases dramatically, and ICP increases above its normal range of 5–13 mm Hg. At this point, any increase in the volume of the three intracranial components has a profound effect on increasing ICP.
Brain tissue volume increases by two mechanisms. Tumors enlarge the parenchymal tissue volume, and surrounding edema increases brain water content. Both contribute to expanding total intracranial mass, which can be compounded by hemorrhage into or around the neoplasm. Surrounding edema may be decreased by administration of steroids, usually dexamethasone, which reduces the activity of sodium-potassium pumps at the choroid plexus epithelial membrane. Improvement of symptoms may occur in hours to days.
CSF is contained in the subarachnoid space of the central nervous system and in the ventricles within the brain. In adults, total CSF volume approximates 150 mL, and daily production is about 600 mL. Enough CSF is synthesized to replace itself three to four times a day. CSF is formed in the choroid plexus and the ventricles within the brain. Production is reduced by decreased CPP and increased ICP, but the latter effect is minimal. Absorption of CSF occurs at the microscopic villi of the subarachnoid membrane. The rate of absorption is a balance between two interrelated factors: CSF pressure and resistance to absorption. Resistance to absorption decreases dramatically as CSF pressure increases to >30 mm Hg; this appears to represent a closed loop venting mechanism to prevent excessive increases in ICP. The amount of CSF present is determined by the balance between production and reabsorption.
Changes in cerebral blood volume usually have little effect on ICP. However, when intracranial compliance is low and intracranial volume has increased to critical levels, these changes may have dramatic consequences.
3
How do anesthetic agents and vasoactive drugs affect cerebral blood flow and intracranial pressure?
The effects of anesthetic agents and vasoactive drugs are multifactorial ( Table 14-1 ). They are described assuming normal brain anatomy and physiology, which is not commonly the situation for neurosurgical patients. Potent inhaled anesthetics are generally cerebral vasodilators, which attenuate cerebral autoregulation. Inhalation anesthetics produce increases in CBF in a dose-dependent manner, while producing progressive depression of cerebral metabolism. The mechanism by which inhalation anesthetics produce vasodilation is not clearly understood. Mechanisms that partially explain the vasodilation include effects on nitric oxide and adenosine triphosphate–dependent potassium channels.
Agent | Effect | Agent | Effect |
---|---|---|---|
Potent inhalation agents | Lowers CMRO 2 Increases cerebral blood flow by vasodilation; this effect is prevented by prior hyperventilation | Curare | Decreases MAP Cerebral vasodilator |
Desflurane | Similar to isoflurane, rapid uptake | Pancuronium | Increases heart rate Increases MAP |
Enflurane | Increases CSF production Decreases CSF reabsorption | Vecuronium * | No significant effects |
Halothane | Decreases CSF production Decreases CSF reabsorption | Atracurium | Large bolus doses may release histamine, predisposing to decreased MAP and cerebral vasodilation |
Isoflurane * | No effect on CSF production Increases CSF reabsorption May protect from ischemia | Alfentanil | Decreases MAP Decreases CPP |
Sevoflurane | Autoregulation maintained at 1.5 MAC | Fentanyl * | With normal or low PaCO 2 plus N 2 O, decreases CMRO 2 , decreases CBF With increased PaCO 2 , cerebral vasodilator |
Nitrous oxide | No change in CMRO 2 , CSF production, or CSF reabsorption Cerebral vasodilating effects inhibited by hyperventilation Expands intradural air Expands venous air emboli | Remifentanil | Decrease in MAP No change in ICP Very short-acting Increases CSF reabsorption |
Barbiturates * | Decreases CMRO 2 Decreases CBF Cerebral vasoconstrictor Decreases CSF production Increases CSF reabsorption | Sufentanil | Decreases CVR Increases CBF |
Ketamine | Increases ICP Increases CBF Increases MAP No change in CMRO 2 No change in CSF production Decreases CSF reabsorption | Lidocaine | Low dose: Decreases CBF, and decreases CMRO 2 High dose: Increases CBF, and increases CMRO 2 |
Etomidate * | Similar to barbiturates Myoclonus indistinguishable from seizures | Sodium nitroprusside | No effect on CBF despite cerebral vasodilatation Decreases MAP Increases CBV |
Benzodiazepines * | Decreases CBF Decreases CMRO 2 | Nitroglycerin | Increases CBF Increases CBV Decreases MAP |
Propofol | Decreases MAP | Hydralazine | Increases CBF Increases CBV Decreases MAP Onset 10–20 minutes |
Succinylcholine | Increases CMRO 2 Increases CBF Increases muscle spindle activity; diminished by pretreatment with nondepolarizing neuromuscular blocker | Labetalol * | Decreases MAP No effect on CBV |

Full access? Get Clinical Tree
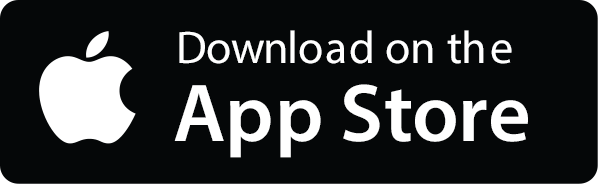
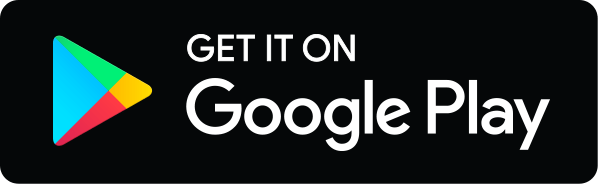
