html xmlns=”http://www.w3.org/1999/xhtml” xmlns:mml=”http://www.w3.org/1998/Math/MathML” xmlns:epub=”http://www.idpf.org/2007/ops”>
Fluid infusions are an essential part of the management of the critically ill, and include maintenance fluids to replace insensible loss and resuscitation efforts to restore blood volume. This chapter is on fluid resuscitation. Fluid administration is a vital component of resuscitation therapy, with the aim being to restore cardiovascular sufficiency. Initial resuscitation aims to restore a minimal mean arterial pressure and cardiac output compatible with immediate survival. This is often associated with emergency surgery and associated life-saving procedures. Then, optimization aims to rapidly restore organ perfusion and oxygenation before irreversible ischemic damage occurs. Stabilization balances fluid infusion rate with risks of volume overload, and finally de-escalation promotes polyuria as excess interstitial fluid is refilled into the circulation. There is no apparent survival benefit of colloids over crystalloids. Hydroxyethyl starch solutions have deleterious effects in certain patient populations, for example in sepsis. Balanced salt solutions are superior to normal saline. Further fluid resuscitation should be guided by the patient’s need for increased blood flow and by their volume-responsiveness.
Introduction
Fluid infusions are an essential part of the management of the critically ill patient. There is a large pool of clinical evidence to guide resuscitation and fluid administration.[1,2] Yet the actual choice for fluids across physicians, hospitals, and counties varies widely.[3] Some of these differences may be driven by economic considerations,[4] whereas others probably reflect habit and lack of familiarity with recent findings and their associated recommendations. Fluid therapy in the intensive care unit can be separated into those fluids infused in patients unable to take fluid orally as maintenance fluids, and those fluids used in resuscitation. The former can become quite complex as insensible fluid losses can vary widely in patients with burns, open wounds, peritonitis, diarrhea, and polyuria. The latter refers to use of fluid therapy as part of resuscitation from circulatory shock. This chapter focuses on fluids in resuscitation.
The resuscitative management of the critically ill patient presenting with circulatory shock can be separated into four phases: rescue, optimization, stabilization, and de-escalation.[5] Rescue or salvage focuses on achieving a minimal arterial pressure and cardiac output compatible with immediate survival. This is often associated with performing appropriate life-saving interventions to treat the underlying cause of circulatory shock. Importantly, fluid resuscitation alone may not restore arterial pressure if either systolic pump function or arterial vasodilation co-exist. In these cases, inotrope and vasopressor therapies, respectively, need to be used. Still, rapid fluid infusion, independent of fluid type, is often indicated because of the presumed decrease in effective circulating blood volume. Importantly, approximately half the patients presenting in circulatory shock are not volume-responsive.[6] Presumably, the causes of shock in those cases include severe cardiac pump dysfunction of various etiologies. Following this short rescue interval, circulatory optimization begins, focusing on restoring global tissue perfusion and oxygenation. This phase often requires invasive hemodynamic monitoring. During this phase, serial measures of arterial and either mixed venous or central venous O2 and CO2 levels, blood lactate, and acid–base status are often used to track resuscitation effectiveness at achieving tissue blood flow and oxygenation adequate to meet the demands of tissue aerobic metabolism. These early phases are often marked by significant violation of integrity of the vascular endothelium with significant capillary leakage, and movement of fluid from the intravascular space to the interstitium resulting in edema, manifesting as a patient who is overloaded in total body fluid yet intravascularly depleted. These phenomena occur commonly in states associated with profound inflammatory response accompanied by cytokine release, such as severe sepsis, trauma, burns, and pancreatitis. Once the circulation has been optimized to reverse tissue hypoperfusion (and there is debate as to what defines such optimization), the stabilization phase commences with the aim of minimizing organ dysfunction due to iatrogenic factors, such as overhydration, with the goal of sustaining organ-system function. The de-escalation phase aims at promoting diuresis as the initial fluid resuscitation needed in the first two phases to maintain an effective circulating blood volume is now mobilized from the tissues and interstitium and returning to the bloodstream. This results in an autoregulatory polyuric response, but occasionally during this phase diuretics or dialysis can be needed to remove this newly resorbed fluid. Within each phase, fluid infusions are usually a central aspect of therapy. However, also important is the choice of which fluid to give as part of resuscitation.
Pathophysiology of cardiovascular insufficiency
Acute cardiovascular insufficiency or shock can be caused by processes that are often grouped into four pathological processes: cardiogenic, hypovolemic, obstructive, and distributive shock.[7] Clinical history and hemodynamic monitoring often allow for both the diagnosis of the shock etiology and monitoring of response to therapy. Importantly, if systemic hypotension co-exists with circulatory shock, vital tissue hypoperfusion will also be present, presenting a medical emergency. This hypotension needs to be rapidly reversed independent of fluid management. In practice, depending on the etiology of shock, a combination of intravenous fluids and vasopressors (e.g. noradrenaline) are used to restore mean arterial pressure. Although a complete description of the diagnosis and management of circulatory shock is beyond the scope of this chapter, some specific concepts are central to fluid management.
Acute cardiogenic shock is usually not associated with volume overload. Volume overload when present in heart failure is a chronic adaptive condition, caused by reactive renal sodium and water retention. Thus, any acute cardiogenic pulmonary edema is due to pump failure (e.g. acute coronary or acute valvular insufficiency) and its resultant elevated pulmonary venous back pressure. The initial resuscitative treatment is systemic arterial afterload reduction and increased inotropy if organ flow is reduced, and fluid resuscitation to sustain adequate filling pressures in the setting of left ventricular diastolic dysfunction, while simultaneously addressing the primary cause of pump failure (e.g. coronary angioplasty for coronary thrombosis, or valvular repair in the setting of acute mitral regurgitation due to papillary muscle rupture). Importantly, heart failure with preserved left ventricular ejection fraction, otherwise known as diastolic dysfunction, occurs commonly. The decreased left ventricular diastolic compliance makes fluid management difficult, with slight decreases in filling pressure causing hypovolemia and slight increases in filling inducing cardiogenic pulmonary edema. Examples of diastolic dysfunction include cor pulmonale secondary to pulmonary hypertension or vascular obstruction and left ventricular hypertrophy due to arterial hypertension or aortic stenosis.
Hypovolemic shock is usually due to hemorrhage, although excess fluid loss from the gut or kidneys, or insensible loss during prolonged surgical interventions with open abdomen or chest, can also cause profound hypovolemia. Importantly, cellular and interstitial fluids move into the vascular space, resulting in a whole-body volume deficiency proportional to the rate of volume loss and its duration. The longer that patients are hypovolemic, the greater their overall fluid deficiency. The normal adaptive response to acute intravascular volume loss is to increase sympathetic tone, decrease fractional excretion of sodium and water from the kidneys and divert blood flow away from less vital organs such as the skin and non-exercising muscle, and eventually causing profound hypoperfusion to the liver, gut, and kidney.[8] Rapid fluid administration (20–30 ml/kg) of crystalloid solution often restores arterial pressure because these patients usually have a high vasomotor tone and normal volume-responsive hearts. If arterial pressure is not restored rapidly to ≥65 mmHg, in an otherwise non-hypertensive patient, then vasopressor therapy needs to be started to reach this minimal arterial pressure target.[9]
Obstructive shock (e.g. massive pulmonary embolism and cardiac tamponade) require, vasopressors to sustain mean arterial pressure and treatments directed at the primary problem (e.g. thrombolysis, pericardiocentesis). Fluid resuscitation is usually only transiently effective in restoring cardiovascular sufficiency and if acute cor pulmonale is present, it can cause further deterioration by dilating further the stressed over-distended right ventricle. In these cases, fluid management needs to be closely titrated by cardiovascular monitoring, including echocardi-ography and hemodynamic monitoring so as to avoid irreversible right ventricular failure.
Distributive shock is caused by dysregulation of normal autonomic control of the circulation. The classic example is septic shock, although other etiologies such as anaphylaxis and high grade spinal anesthesia, as well as frank right ventricular failure, can present with a similar picture. With non-specific vasodilation, the unstressed blood volume greatly increases, making the patient appear to be hypovolemic because the driving pressure for venous return is markedly decreased. Here initial aggressive fluid resuscitation (20–30 ml/kg) to restore venous return and central venous pressure, combined with vasopressor therapy, if needed to maintain a mean arterial pressure >65 mmHg, is often required.[9] Once initial rescue has occurred, further aggressive resuscitation to optimize organ blood flow is required, driven by end-organ function and evidence of tissue hypoperfusion. Importantly, septic shock usually has elements of hypo-volemia along with loss of vasomotor tone.
As listed above, loss of vascular endothelial tight junction integrity often occurs in septic shock, leading to markedly increased plasma transudation into the interstitium, leading to secondary hypovolemic shock. Thus, the fluid requirements in severe sepsis and septic shock are often much greater than would be the case if pure vasodilation alone without associated inflammatory component were present. Indirect estimates of tissue hypoperfusion include venous O2 saturation (SvO2) and the veno-arterial PCO2 gradient.[10]
Since loss of vascular endothelial integrity commonly accompanies septic shock, referring to the Starling forces principle, many clinicians prefer using colloid solutions for resuscitation in an effort to retain the infused fluid in the intravascular space. However, recent work calls into question completeness of the initial Starling forces principle.[11] In sepsis and markedly altered circulatory states, the transvascular barrier, which comprises the endothelial glycocalyx layer and endothelial basement membrane, with tight junctions between cells and extracellular matrix, is damaged.[12] When the vascular barrier is intact, transcapillary movement of fluid is unidirectional, as there is no absorption of fluid from the interstitium back to the intravascular space, and drainage of the interstitium is accomplished primarily by lymphatic clearance. Transcapillary movement is then dependent on capillary pressure. Increasing capillary pressures increases edema formation. Under the same conditions, infusion of crystalloid solutions also increases capillary pressure, but by dilution decreases oncotic pressure, thus resulting in more transcapillary movement than colloids. At subnormal capillary pressures, however, transcapillary movement nears zero; thus both crystalloids and colloids result in increase in capillary pressure, but neither sufficient to result in change in transcapillary movement. Indeed, when physicians were blinded to the infusion of solution when managing patients in septic shock, they gave the same amounts of albumin and of crystalloid solution during resuscitation,[13] suggesting that the hemodynamic response to albumin and crystalloids infusion in a septic patient is not clinically significantly different.
Although much attention focuses on the rescue and optimization phases of resuscitation, management during stabilization and de-escalation is also important, because it is in those times that the deleterious effects of fluids become most manifest, presumably because the risks of the pathological process that caused the initial illness subsides. Still, those fluid-associated risks are probably present throughout all resuscitation phases.
Choice of resuscitation fluids
Primary decisions for fluid administration include the use of colloid or crystalloid, and which specific colloid or crystalloid to use within each family. The goal of resuscitation is initially to support intravascular volume and promote tissue perfusion, while minimizing causing interstitial edema.[14] All resuscitation fluids will expand the intravascular space.[15] The available colloids include albumin, hydroxyethyl starch (HES), gelatin, and dextran. Available crystalloids include 0.9% normal saline (NS), lactated Ringer’s (LR), Hartmann’s solution, and several similar balanced salt solutions (e.g. Plasma-Lyte, Normo-Sol). As clinical trials expand, analysis has switched from the colloid versus crystalloid debate to which colloid or crystalloid to use.[16] It should be noted that intraoperative choices of resuscitation fluids follow different physiology, and the discussion becomes altered when one considers resuscitation fluid choices in the ICU.
Crystalloids
Crystalloids are aqueous fluids that contain low-molecular-weight crystal-forming elements (electrolytes), which easily pass through vascular endothelial membrane barriers followed by water, leading to their equilibration between the intravascular and extracellular space.[17]
Crystalloid solutions can contain a variety of inorganic cations, such as K+, Ca2+, Mg2+, and organic anions such as lactate, acetate, gluconate, or bicarbonate as well as Cl−, allowing the Na+, Cl−, and K+ values to vary independent of each other. The term “normal” saline is a misnomer which was coined because its concentration 0.9% w/v is “normal” or about 3,000 mOsmol/l (or 9 g/l), not because its composition is normal or “physiological” as an electrolyte solution. Normal saline (NS) is slightly hypertonic and has equal amounts of Na+ and Cl−, making it both hypernatremic and hyperchloremic relative to plasma. Thus, NS infusions promote hypernatremia and hyperchloremic metabolic acidosis, resulting in renal vasoconstriction.[18] NaCl is available in varying degrees of non-hyperosmolar dilution (0.9, 0.45, and 0.225 NaCl) alone or with 5% dextrose. NaCl solutions are compatible with co-infusion of blood products. LR and other balanced salt solutions containing Ca2+ are not. Crystalloid alternatives to NS represent fluids more closely resembling the electrolyte composition of plasma, including LR, Hartmann’s solution, Normo-Sol, and Plasma-Lyte.
Studies have compared different crystalloid solutions in resuscitation, specifically chloride-liberal (i.e. NS) versus chloride-restricted (balanced salt) solutions.[19] These results come primarily from perioperative literature including mainly trauma patients and in-patients undergoing major abdominal surgery, and suggest that the use of balanced salt solutions in some patient populations decreases mortality and the incidence of acute kidney injury (AKI) when compared with NS. Subsequent studies of 2,012 patients demonstrated a decrease in incidence of AKI and in use of renal replacement therapy (RRT) in ICU patients with implementation of chloride-restricted strategy.[19] The use of NS has long been known to be associated with increased risk of hyperchloremic metabolic acidosis,[20] but it has only recently been shown that these metabolic changes can result in decreased renal blood flow and renal cortical hypoperfusion, as demonstrated in healthy volunteers.[18] These data suggest that the choice of crystalloid solution should be guided by individual patient characteristics so as to decrease use of NS in patients in whom its metabolic profile can cause harm.
Colloids
Colloids are aqueous solutions that contain both large organic macromolecules and electrolytes. These molecules are retained within the intravascular space to a greater degree than pure crystalloids under normal conditions owing to their higher oncotic pressure.
The first colloid solution used clinically was albumin. It is available in several concentrations (4%, 5%, 20%, and 25%). The greatest barrier to its use has been its cost. Synthetic colloids, in particular starches (hydroxyethyl starch, HES), gelatins, and dextran, present more economical alternatives. Gelatins are derived from bovine gelatin, and their colloid base is protein. HES are derived from potato or maize starch, and their colloid base is large carbohydrate. Solutions of various molecular weight are available (130, 200, and 450 kilodaltons, kDa). Dextran is also a polysaccharide-based colloid, made by bacteria during ethanol fermentation. The oncotic pressure of these solutions varies depending on the molecular weight and concentration, and both hypo-oncotic (gelatins, 4% and 5% albumin) and hyperoncotic solutions (20% or 25% albumin, dextran, and HES 6% and 10%) are available. Although albumin is degraded, the hydroxylation of starches in HES and dextran results in their accumulation in skin, kidney, and liver, resulting in organ-specific clinical manifestations and potential morbidities such as AKI or liver injury.[20–22] There is significant evidence that the use of HES in the ICU increases morbidity. Its use as a resuscitation fluid increases both serum creatinine and use of RRT in clinical trials [23,24] and in meta-analyses.[25–32] These effects likely result from the tissue accumulation with prolonged or repeated use, and based on these data, HES use in Europe has recently been limited to use only for routine intraoperative volume expansion.
Colloid versus crystalloid
Although, during conditions of normal capillary pressure, colloids result in transient greater increase in intravascular volume, it has not been shown that greater intravascular volume expansion translates to improvement in mortality outcomes.
Several studies focused on albumin as the comparator colloid. The large multicenter SAFE trial [13] showed no difference in mortality with the use of albumin versus NS, with the exception of the subgroup of traumatic brain injury patients whose outcomes were worse with albumin.[13] Recent meta-analysis suggests that in sepsis, albumin is superior to other colloids and NS but not to other balanced crystalloid solutions.[30] Still, the ALBIOS trial,[33] which compared 20% albumin with crystalloid in 1,800 severe sepsis and septic shock patients, does not corroborate this finding. Albumin-treated patients had higher serum albumin level and higher mean arterial pressure, but no differences in mortality at 28 or 90 days. A post-hoc subgroup analysis of only septic shock patients (>1,100 of the 1,800) showed a 90-day survival benefit in the albumin-treated patients, whereas the albumin-treated group in patients without septic shock had an increased mortality.
Multiple studies (Table 27.1) [19,23–25,33,34] and recent meta-analyses (Table 27.2) [26–32] evaluated the outcomes associated with the use of synthetic colloids, showing no benefit of individual synthetic colloids over other colloids or over crystalloids. Resuscitation with HES comes to question on the basis of mortality risk as well as risk of renal insult. HES resuscitation increased 90-day mortality when compared with LR in 800 patients with severe sepsis in the 6S trial.[23] The CHEST trial [24] showed no difference in mortality between HES and NS in a 7,000-patient general ICU population, and Bagshaw and Chawla [25] showed no mortality difference in a 7,000-patient multicenter randomly controlled trial comparing HES with NS. Similarly, a study evaluating goal-directed fluid therapy in colorectal surgery showed no mortality benefit of HES over balanced crystalloid solution.[34] Three recent meta-analyses [27,28,30] support the conclusion that use of HES in resuscitation does not reduce mortality when compared with other resuscitation fluids. In contrast, the recent CRISTAL trial, which studied 2,857 patients with hypovolemic shock, sepsis, and trauma,[35] and compared administration of colloids (of which HES was the most used) with crystalloids (of which NS was the most used), showed better 90-day survival in the colloid group. Although this study has been criticized amply, its conclusions leave unresolved the question of mortality risk of HES alone, rather than all colloids. Furthermore, given that the data regarding HES vary with variables such as molecular weight in ways that are not consistent with any particular hypothesis, this suggests that confounding factors may exist that are not being accounted for. One such confounding factor may in fact be the electrolyte composition of solution used for preparation of the starches.

Full access? Get Clinical Tree
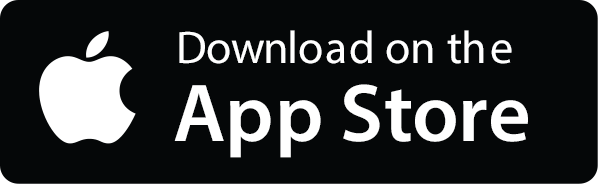
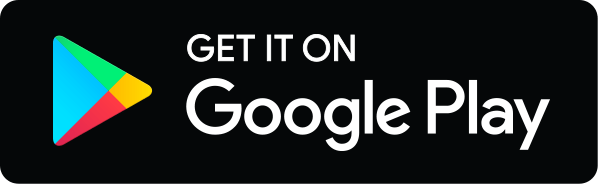
