As for the orientation of the pelvis, Fig. 7.3 illustrates the position of the bony structures in different postures. It is obvious that injury resulting for example from impact to the knee depends on the posture at the moment of impact.
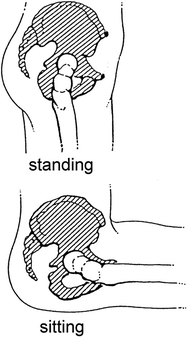
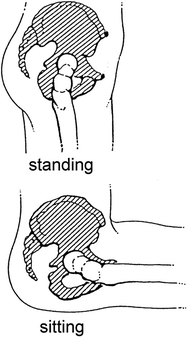
Fig. 7.3
Orientation of the hip in different postures: standing (top) and sitting (bottom) (adapted from Kramer 1998)
Differences between the male and female pelvis are also apparent, but will not be discussed here. Although the shape and the mechanical properties of the male and female bones are slightly different, their influence on injury mechanisms concerning automotive impacts are regarded minor—in contrast to various anatomical and physiological factors that might lead to different injury rates in sports injuries (e.g. Boles and Ferguson 2010).
The femur is the long bone of the thigh and is proximally connected by the hip joint to the pelvis and distally linked to the knee. The different regions and landmarks of the femur are shown in Fig. 7.4. Two bones, the tibia and the fibula, form the lower leg between the knee and the ankle. The knee is the joint that connects the femur and the lower leg (Fig. 7.1). It is an anatomically dense area involving several muscles, tendons, ligaments and menisci. Moreover, vulnerable structures of the knee like the patella are often subjected to direct impact. A strong musculature which can create considerable forces and thus may influence the injury mechanisms (see Sect. 7.2.2) surrounds the legs.
Finally the foot is adjoined to the lower leg. A foot consists of several bones: calcaneus and talus are located at the proximal end, the metastarsal bones and phalanx at the dorsal end (Figs. 7.1 and 7.5).
7.2 Injury Mechanisms
Regarding the pelvis and the lower extremities, fractures are the most common injuries sustained in accidents. Such fractures result from sports accidents or falls, respectively, rather than from automotive accidents. Hip fractures, for example, which are often caused by falls, particularly concerning the elderly, are a major concern in public health (Majumder et al. 2008). In contrast, pelvis fractures sustained in automotive accidents are quite rare. They contribute to only about 1 % the total Injury Priority Rating (IPR) (King 2002). Analysing frontal impacts of passenger cars, Kramer (1998) found that, while head injuries were the most common injuries sustained by 35 % of all occupants, pelvis and hip injuries were present in only about 7 % of all cases. However, 25 % of the occupants had leg and foot injuries. Similar results were obtained when evaluating the NASS data base (Crandall 2001). Moreover, checking for AIS ≥ 2 injuries (AIS, i.e. Abbreviated Injury Scale) in frontal impact, a strong influence of the restraint systems on the likeliness of injuries of the lower extremities was apparent. It was observed that the percentage of lower limb injuries is about twice as high than that for head injuries when the occupant is belted and the vehicle is equipped with airbags. The analysis of the different regions of the lower extremities showed that the feet and the ankles are at highest risk for AIS ≥ 2. Additionally, Morris et al. (2006) found that, based on UK accident data, lower extremity AIS ≥ 2 injuries are by far the most costly injuries and account for some 43 % of injury costs in both front and struck-side crashes.
Due to the fact that the pelvis and the proximal femur are often simultaneously injured, such injuries are commonly referred to as hip injury whereas the word “hip” does neither describe a particular anatomical structure that is injured nor is “hip injury” related to a particular injury mechanism. In the strict sense, the hip is the bony structure around the hip joint (femur head, pelvis, acetabulum). However, fractures involving the proximal part of the femur are commonly called hip fractures as well.
Generally, fractures are either open or closed. While the skin and soft tissues overlaying the fracture are intact in closed fractures, the bone is exposed to direct outside contamination in open fractures. Further characteristics to classify fractures include the position of the fractured segments (displaced/undisplaced), the location of the fracture along the bone (intraarticular, metaphyseal, diaphyseal) and others (see e.g. Levine 2002). Concerning fracture of long bones in general and with particular respect to the bony structures of the legs, fracture patterns are differentiated depending on the loading condition that caused the fracture, i.e. the injury mechanism. There are four possible types of fracture mechanisms: direct loading, indirect loading, repetitive loading and penetration. In motor vehicle accidents direct and indirect loading are the most frequent types. If in a frontal collision the knee of an occupant hits, for example, the dashboard, direct loading can cause fracture of the patella, indirect loading may lead to fractures of the femur shaft or the acetabulum (Fig. 7.6). Different fracture patterns that can arise from direct and indirect loading, respectively, are presented in Fig. 7.7.
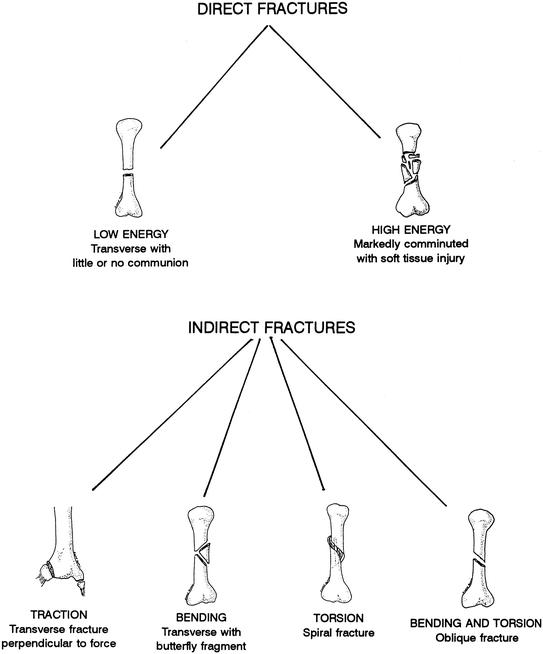
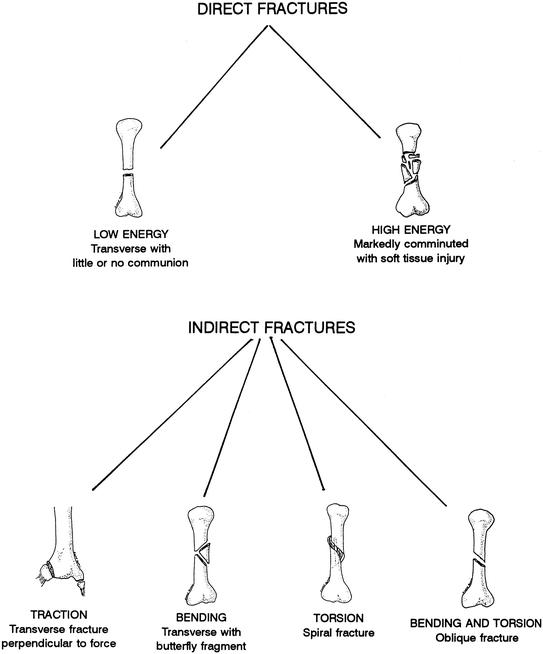
Fig. 7.7
Types of fracture according to loading (from Levine 2002). It should be noted that bending fractures can also occur directly, i.e. they are also a form of direct fracture
Like other injuries, injuries of the pelvis and the lower extremities are categorised in the AIS (Table 7.1). In the following sections possible injuries and their injury mechanisms are discussed; they are limited, however, to impact related injures only.
AIS code | Description |
---|---|
1 | ankle, hip: sprain, contusion |
2 | patella, tibia, fibula, calcaneus, metatarsal: fracture pelvis: fracture (closed, undisplaced) toe: amputation, crush hip, knee dislocation muscles, tendons: laceration (rupture, tear, avulsion) |
3 | femur: fracture pelvis: fracture (open, displaced) traumatic amputation below knee |
4 | pelvis: “open book” fracture traumatic amputation above knee |
5 | pelvis: substantial deformation with associated vascular disruption and blood loss >20 % by volume |
6 | – |
7.2.1 Injuries of the Pelvis and the Proximal Femur
Injuries to the pelvis are categorised clinically as isolated fracture of the pelvic ring, multiple fractures of the pelvic ring, sacrum fracture and associated injuries. An isolated fracture of the pelvic ring consists of a single fracture around the pelvic ring (for example of the pubic ramus or the ilium). Pubic ramus fractures are frequently observed in lateral impacts when the greater trochanter is hit. The pelvic ring remains stable after isolated fractures, i.e. significant displacement of the fractured segments is not found. This is different if multiple fractures occur. Here the pelvic ring becomes unstable enabling large displacement of the fractured segments. Uro-genital injuries can arise in combination with multiple pelvic fractures.
Sacrum fracture occurs in extensive pelvic injuries, fracturing usually across the foramina or in the vicinity of the holes through which the sacral nerves pass. Obviously, these nerves are also at danger in case of such injury. Moreover additional injury, especially haemorrhage, can be associated with pelvic fractures. Excessive bleeding from the large blood vessel in the pelvic wall as well as from the fractured surfaces themselves can be life-threatening (even if proper surgery is applied).
From a biomechanical point of view, the underlying mechanisms of pelvic fracture are either compression, vertical shear or a combination thereof. Compression of the pelvis can further be differentiated into lateral and frontal (i.e. anterior-posterior) compression. Figure 7.8 illustrates the possible locations of fracture in case of lateral compression. If an anterior-to-posterior directed force compresses the pelvis centrally, so-called straddle fractures occur, i.e. multiple fractures of the pubic rami. Anterior-posterior (a-p) compression with impact forces on the right and left iliac crest can result in a hinge or “open book” fracture (Fig. 7.8). Thus in a-p compression the pelvic diameter is acutely increased causing tensile forces to act on tissue hosted by the pelvis and possibly violating ligaments. If the pelvis is subjected to vertical loading, shear can cause fracture as well as rupture of ligaments (Fig. 7.9).
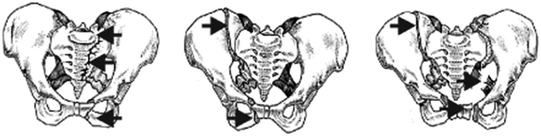
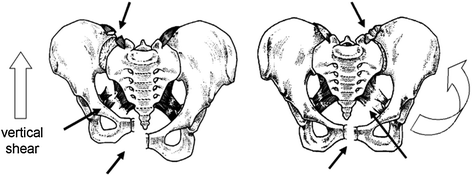
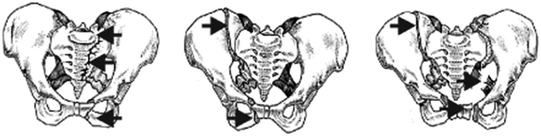
Fig. 7.8
Possible locations for fracture arising from lateral compression (adapted from Vetter 2000)
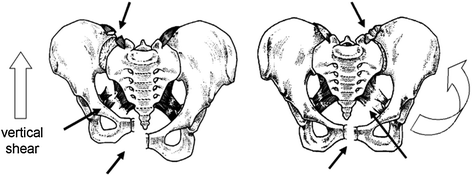
Fig. 7.9
Fracture and ligament rupture due to vertical shear forces (left). “Open book” fracture (right) (adapted from Vetter 2000)
Pelvic fractures used to be predominant in pedestrian collisions up to the early 1990s. When the pedestrian was struck by a car laterally on the pelvis, fractures of the pubic rami occurred (prevailing on the non-struck side). This type of injury has nearly vanished in today’s pedestrian collisions. Due to changes in the front shapes and the front structures of recent cars, pedestrian kinematics seem to be different such that pelvic fractures are prevented (Otte 2002; Snedeker et al. 2003; Simms and Wood 2009).
As described above, the hip is frequently injured in falls. Such lateral loading of the hip commonly causes the femur neck to fracture. Femoral neck fractures in geriatric patients tend to be very low energy injuries (Levine 2002) in particular, as processes such as osteoporosis increase the fracture risk. Falls are furthermore of concern with epileptic patients whereby in addition to the hip also the head is primarily involved; yet, severe injuries are rare (e.g. Lawn et al. 2004). In turn, goalkeepers in soccer are frequently exposed to sideways falls due to their task (Schmitt et al. 2008a, 2009). Various protection devices in the form of (visco-elastic) padding are in use for hip protection of elderly as well as of athletes (e.g. Schmid Daners et al. 2008; Schmitt et al. 2008b).
In automotive accidents, however, fracture of the proximal femur is rare. Lateral impact is more likely to cause fracture of the pubic rami instead. Luxation and dislocation of the hip joint (Fig. 7.10), possibly in combination with fracture of the acetabulum, can also result from lateral impact. In general, the hip joint is dislocated when the hip is flexed and adducted and simultaneously loaded along the femur in rearward direction. These conditions apply, for instance, to occupants experiencing a frontal collision while sitting with their legs crossed.
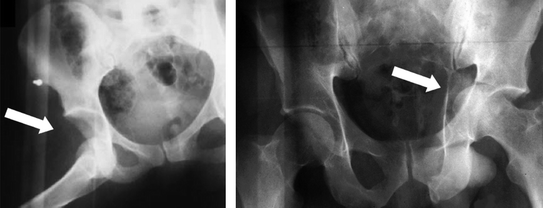
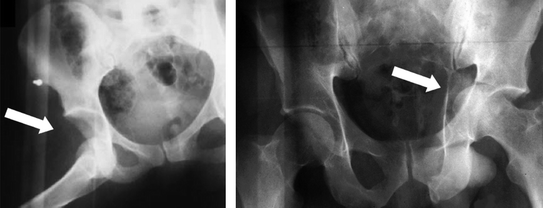
Fig. 7.10
Left Dislocation of the right hip. Right Dislocation and acetabulum fracture of the left hip after lateral impact. The head of the femur broke through the medial wall of the acetabulum (courtesy Prof. F. Walz)
7.2.2 Leg, Knee and Foot Injury
Addressing the injury mechanism for femur fractures sustained in frontal automotive collisions by interaction with the dashboard, it was found that axial compression accounts for the vast majority of fractures (62 %), followed by bending (24 %), torsion and shear (each 5 %) (Crandall 2001). In addition to the mechanical loading conditions, the fracture pattern of femur shaft fractures is influenced by the fact that the femur is not perfectly straight, i.e. it is slightly curved so that the convex side faces forward. This plays a role especially in indirect loading of the femur (Fig. 7.6).
Impact to the knee can cause the patella to fracture (direct loading of the patella). Indirect loading of the patella can likewise occur from strong muscle contraction (quadriceps) on the partially flexed knee, possibly resulting in patella fracture (Levine 2002). Of importance is furthermore the impact angle with respect to the upper leg at which the knee is hit (Meyer and Haut 2003). Unlike the hip joint which has a stable ball-and-socket joint (Sect. 7.2.1), the bone anatomy of the knee imparts little support to the joint’s stability. This makes the knee ligaments prone to injury (see also Sect. 7.5). When the knee is bent and an object forcefully hits the tibia backwards, the posterior cruciate ligament can be torn (this injury is commonly called “dashboard injury”). In lateral impacts (e.g. pedestrian impacts) also collateral ligament rupture is seen. Generally complete dislocation of the knee joint can result in tears of the major four knee ligaments.
In analogy to femur fractures, tibia fractures may be caused through direct or indirect loading of the leg. Tibia fractures are the most common fractures in long bones, and because the tibia lies subcutaneously (i.e. the bone is only covered by the skin), such fractures are often open fractures. Most fractures occur between the mid-shaft region and the distal third of the tibia, where the smallest cross-section and the smallest cross-sectional moment of inertia are found. As for the impact conditions, Crandall (2001) reported that for tibia as well as for fibula injuries, axial loading and direct impact are equally probable. If both the tibia and the fibula are fractured, stability depends on the level of the fractures, i.e. the fracture is more unstable if both bones are broken on the same shaft level. A more severe type of tibia fracture is the tibia plateau fracture, i.e. a fracture of the tibia including a disrupture of the articular surface of the knee joint. Tibial plateau fractures make up for only 1 % of all fractures seen clinically, but account for 10 % of all below-knee AIS ≥ 2 injuries sustained in vehicle crashes (Crandall 2001). Mechanisms resulting in tibia plateau fracture include direct impact to the knee, axial loading and axial loading plus hyperextension of the knee. Axial loading might occur due to simultaneous floorboard intrusion and knee entrapment (e.g. if the knee gets caught under the dashboard).
The injury mechanisms of ankle and foot injuries are closely related to the possible motion range of the ankle and the hindfoot (Fig. 7.11). According to Crandall (2001), ankle injuries sustained in frontal automotive collisions are primarily due to axial load (58 %), inversion (15 %) and eversion (11 %). Only 5 % resulted from direct contact. Metatarsal injuries, however, are solely caused by direct impact and 100 % of all calcaneus injuries are due to axial loading. Pure axial loading can also result in talus fractures and so-called pylon fractures, i.e. intra-articular fractures of the distal tibia involving the calcaneus, talus and the distal tibia, for instance by driving the talus into the tibia. Dorsiflexion (e.g. forced in crashes with toepan intrusion) and entrapment of the knee can increase axial forces.
Inversion and eversion account for the vast majority of malleolar injuries, especially ankle fractures, making the ankle the most frequently injured major joint of the human body (see also Sect. 7.5). Furthermore, the rate of rotation, the orientation of the ankle and individual factors of the occupant like age or pre-existing damage were found to influence the likeliness of malleolar injury (Crandall 2001).
Foot injuries, particularly metatarsal fractures that were sustained in automotive accidents, result mainly from contact to the foot pedals. If, in addition, the heel strikes the floorboard, local loading to the calcaneus occurs, possibly leading to its fracture (Levine 2002).
Investigating the effect of preload in the Achilles tendon with respect to ankle injuries, cadaver experiments showed that a 2 kN Achilles preload affects pylon fracture by decreasing the external force needed to cause this fracture (Kitagawa et al. 1998a). Achilles preload also leads to tension fractures of the calcaneus. Additionally, injury mechanisms are influenced considerably by the musculature of the lower extremities. Cappon et al. (1999) report the posterior muscles (plantarflexors) to be able to generate more than 3.5 kN and for the anterior muscles (dorsiflexors) 1.4 kN were determined (Petit et al. 1996). Likewise, in pre-impact bracing muscles can contribute more than 2.8 kN to the load of the lower extremities (Crandall et al. 1995). Sled testing and computer simulations showed that muscle tension largely increases the axial forces, increases the effective mass and stiffness of the leg, accounts for redistribution of the stress within the bones, and alters the kinematics with less forward excursion and small joint rotation (Crandall et al. 1995). However, to gain a better understanding of the injury mechanisms of lower limb injuries, more studies addressing the role of muscle force are necessary.
7.3 Impact Tolerance of the Pelvis and the Lower Extremities
The response to mechanical loading of isolated bones such as the femur, the tibia or the fibula was measured from experiments similar to those used in material testing (e.g. bending and tension tests). Table 7.2 summarises such results.
Table 7.2
Mechanical strength (average values) of the bones of the lower limbs as reported by Levine (2002)
Femur | Tibia | Fibula | ||||
---|---|---|---|---|---|---|
Male | Female | Male | Female | Male | Female | |
Torque (Nm) | 175 | 136 | 89 | 56 | 9 | 10 |
Bending (kN) | 3.92 | 2.58 | 3.36 | 2.24 | 0.44 | 0.30 |
Average maximum moment (Nm) | 310
![]() Full access? Get Clinical Tree![]() ![]() ![]() |