Inhibition and Facilitation of Nociception
Jürgen Sandkühler
The intensity of pain felt by a patient may not always match the level of nociceptor activation. For example, immediately after an accident a severely wounded patient may feel no pain at all for some time, whereas a minor trauma might have evoked intense pain in the same person. In a healthy human subject, the intensity of pain induced by identical, repetitive noxious stimuli may vary with a circadian rhythm. In situations of emotional stress, during prolonged body exercise (e.g., among long distance runners), and during sexual intercourse the pain threshold may be elevated considerably. On the other hand during infection and other forms of disease, pain thresholds may be generally lowered as part of the sickness syndrome. This mismatch between the level of nociceptive input and the perceived intensity of pain can now be explained by neuronal circuits in the central nervous system that modulate the transmission of nociceptive information. Neurons in spinal dorsal horn or in its trigeminal correlate, the trigeminal subnucleus caudalis (medullary dorsal horn), are relay stations that receive nociceptive information from peripheral nociceptors and transmit this information to the brain, which ultimately leads to the sensation of pain. These relay stations are important sites for clinically relevant inhibition or facilitation of nociception. All vertebrates investigated so far, including humans, possess powerful endogenous antinociceptive systems that may reduce or even abolish transmission of nociceptive information at spinal or trigeminal relays. Some of the most effective forms of pain therapy known today activate these endogenous mechanisms of antinociception. The progress in the treatment of pain over the last decades witnesses some outstanding examples for rapid and successful transfers of discoveries made in basic sciences to clinical applications. On the other hand, effective clinical treatments of pain syndromes have guided basic researchers to identify new antinociceptive principles in the nervous system. The neuronal mechanisms of endogenous inhibition and facilitation of nociception and their clinical implications are summarized herein.
Various forms of endogenous antinociception have been identified that can be classified into two major categories: (a) descending inhibition of nociception in spinal or medullary dorsal horn by systems that originate from brainstem sites and (b) afferent-induced inhibition in spinal or medullary dorsal horn, which is evoked by stimulation of sensory nerve fibers. In any case, the final inhibition takes place at or near the first synapse of the nociceptive pathways. Even though most of the previous work has been dealing with spinal dorsal horn mechanisms of antinociception, numerous studies show that similar or identical mechanisms also apply to the trigeminal subnucleus caudalis (5,40). Recent evidence suggests that an imbalance in activity between brainstem nuclei mediating antinociception and vascular control may be relevant to the pathogenesis of migraine (28,47).
DESCENDING INHIBITION OF NOCICEPTION
Powerful supraspinal descending systems have been identified that are mainly inhibitory, but facilitatory effects have also been observed. These systems are tonically active and depress spinal nociception under physiologic conditions. In addition, descending inhibition can be activated by environmental stimuli, pharmacologically, or by deep brain stimulation.
Tonic Descending Inhibition
The existence of tonic descending inhibition of spinal nociception is now well established. Complete spinalization or circumscribed lesions in the lateral funiculi of the spinal cord invariably and immediately lead to a disinhibition of nociceptive neurons in spinal dorsal horn caudal to the lesion. Both background activity and stimulus-evoked responses of nociceptive spinal dorsal horn neurons are strongly enhanced and thresholds for nociceptive behavior are lowered in spinalized animals. This indicates
that the transmission of nociceptive information in spinal cord is subject to tonic descending inhibition from pathways that travel in the lateral funiculi. The supraspinal origins of these descending antinociceptive systems have not been identified with certainty. In the cat, but not in the rat, the medullary lateral reticular nucleus may be involved. The spinal mechanisms of tonic descending antinociception are largely unknown. It has been suggested that some forms of chronic, spontaneous pain and centrally mediated hyperalgesia may result from insufficient activity in these tonic descending antinociceptive pathways. Regular fluctuations of activity in these systems, for example, during sleep-wake cycle, may contribute to the circadian rhythm of nociception (reviewed by Millan [29] and Willis and Coggeshall [48]).
that the transmission of nociceptive information in spinal cord is subject to tonic descending inhibition from pathways that travel in the lateral funiculi. The supraspinal origins of these descending antinociceptive systems have not been identified with certainty. In the cat, but not in the rat, the medullary lateral reticular nucleus may be involved. The spinal mechanisms of tonic descending antinociception are largely unknown. It has been suggested that some forms of chronic, spontaneous pain and centrally mediated hyperalgesia may result from insufficient activity in these tonic descending antinociceptive pathways. Regular fluctuations of activity in these systems, for example, during sleep-wake cycle, may contribute to the circadian rhythm of nociception (reviewed by Millan [29] and Willis and Coggeshall [48]).
Descending Antinociception Activated From Brainstem Sites
Analgesia is often a characteristic feature of a more complex behavioral response pattern. For example, acute, massive stress is typically accompanied by cardiovascular and respiratory reactions and also by profound analgesia. Nociceptive thresholds may also be elevated during physical exercise and sexual intercourse. These complex and highly integrated activities are organized at supraspinal levels, suggesting that the corresponding antinociception is also initiated supraspinally. Indeed, electrical stimulation at brainstem sites may induce strong antinociception (34). This phenomenon was then systematically investigated by numerous groups and, as a consequence, deep brain stimulation was soon introduced into clinical practice as a new therapeutic tool in human pain patients. Stimulations at numerous sites in brainstem, diencephalons, and somatosensory cortex were shown to depress or abolish nociceptive neuronal responses in spinal dorsal horn (Fig. 11-1) (reviewed by Gebhart and Randich [12]). Some of the antinociceptive stimulation sites have direct descending projections to spinal cord via long serotonergic, adrenergic, and/or peptidergic nerve fibers that mainly travel in the dorsolateral or ventrolateral funiculi. The major antinociceptive stimulations sites that send direct projections to spinal or medullary dorsal horn are listed from caudal to rostral: Antinociceptive neurons in the lateral reticular nucleus of the caudal ventrolateral medulla are tonically active in some species and can be
further activated by focal stimulation. The inhibition involves the release of norepinephrine and activation of α2-adrenoceptors in spinal cord. Because the direct projections from the lateral reticular nucleus to spinal cord are not adrenergic, an ascending-descending loop via adrenergic cell group A5 may also be involved (vide infra). The medullary nucleus raphe magnus and adjacent reticular formation have strong, direct serotonergic projections to spinal dorsal horn and stimulation in nucleus raphe magnus may produce a powerful antinociception with relatively few observable “side effects.” In spinal cord the, raphe-spinal inhibition is mediated by 5-HT1, GABAA, and GABAB receptors and possibly also by opioid receptors but not by glycine receptors. Some raphe-spinal neurons display discharge patterns that correlate well with the level of vigilance and may be most active during sleep. In the pons, the locus coeruleus/subcoeruleus is the major source of direct noradrenergic innervation of the spinal cord in some species and stimulation in this nucleus also produces a strong antinociception that is mediated by spinal α2-adrenoceptors. Neurons in nucleus locus coeruleus change activity with different levels of vigilance from arousal to sleep-wake cycle and play a role in vestibulospinal reflexes and spinal motor output. Locus coeruleus neurons also control cerebral circulation and may induce vascular changes similar to those in migraine (2). The parabrachial area, including the A7 adrenergic cell group and the Kölliker-Fuse nucleus in the midbrain, also sends direct antinociceptive fibers to the spinal cord. In addition these nuclei project to preganglionic sympathetic neurons in the thoracic intermediolateral column.
further activated by focal stimulation. The inhibition involves the release of norepinephrine and activation of α2-adrenoceptors in spinal cord. Because the direct projections from the lateral reticular nucleus to spinal cord are not adrenergic, an ascending-descending loop via adrenergic cell group A5 may also be involved (vide infra). The medullary nucleus raphe magnus and adjacent reticular formation have strong, direct serotonergic projections to spinal dorsal horn and stimulation in nucleus raphe magnus may produce a powerful antinociception with relatively few observable “side effects.” In spinal cord the, raphe-spinal inhibition is mediated by 5-HT1, GABAA, and GABAB receptors and possibly also by opioid receptors but not by glycine receptors. Some raphe-spinal neurons display discharge patterns that correlate well with the level of vigilance and may be most active during sleep. In the pons, the locus coeruleus/subcoeruleus is the major source of direct noradrenergic innervation of the spinal cord in some species and stimulation in this nucleus also produces a strong antinociception that is mediated by spinal α2-adrenoceptors. Neurons in nucleus locus coeruleus change activity with different levels of vigilance from arousal to sleep-wake cycle and play a role in vestibulospinal reflexes and spinal motor output. Locus coeruleus neurons also control cerebral circulation and may induce vascular changes similar to those in migraine (2). The parabrachial area, including the A7 adrenergic cell group and the Kölliker-Fuse nucleus in the midbrain, also sends direct antinociceptive fibers to the spinal cord. In addition these nuclei project to preganglionic sympathetic neurons in the thoracic intermediolateral column.
Effective antinociceptive stimulation sites are also found in areas of the brain that have no direct projections to spinal cord. The descending inhibitions evoked from these sites are mediated via synaptic relays in one or more of the mentioned brainstem nuclei. From caudal to rostral: Neurons in the nucleus of the solitary tract may mediate descending antinociception, probably via a relay in the lateral reticular nucleus. This inhibition can be triggered by sensory stimulation of vagal cardiopulmonary afferents. Acute noxious stimuli may activate a descending inhibition of nociceptive responses in those spinal segments that do not process the conditioning noxious stimulus. This phenomenon has been termed diffuse noxious inhibitory control and appears to be mediated by neurons in the subnucleus reticularis dorsalis. In healthy subjects but not in patients with lesions in the retro-olivary part of the medulla (Wallenberg syndrome), a diffuse noxious inhibitory control of pain perception can be elicited (44). Descending inhibition induced by stimulation in various subdivisions of the midbrain periaqueductal grey (PAG) and the nucleus raphe dorsalis has been intensively studied (Fig. 11-2). Descending inhibition from the PAG is partly mediated by synaptic relays in the medullary nucleus raphe magnus and adjacent reticular formation. However, during focal chemical stimulation at the various subdivisions of the PAG, numerous neurons inside the PAG (39) and in well-defined nuclei of the brainstem are also activated. Thus, focal stimulation of cell bodies at well-defined brainstem sites may activate a large number of nearby and remote nuclei in brainstem that may act synergistically to produce antinociception and may also serve other functions, for example, during stress response or defense reaction (36). Neurons in the anterior pretectal nucleus can be activated by electrical stimulation of dorsal column fibers and may mediate a descending inhibition via reticulospinal neurons in the ventrolateral medulla. This may be a mechanism underlying analgesia by electrical stimulation of the dorsal columns. Results obtained by positron-emission tomography in patients during a spontaneous migraine attack suggest that pathologic activity in brainstem nuclei regulating antinociception and vascular control may be related to the pathogenesis of this disease (47). Recently, a discrete acute sclerotic lesion in the PAG could be identified as the cause for severe headache in a young woman.
Opioid Analgesia via Supraspinal Descending Systems
Three families of endogenous opioids have been identified that include dynorphins binding predominantly to κ-opioid receptors, enkephalins that bind to δ-opioid receptors, and β-endorphins that bind to μ-opioid receptors. Opioid receptors are found at numerous sites in the brain and in virtually all of the mentioned antinociceptive brainstem sites. Different theories have been advanced to explain the action of opioids in the brain (reviewed by Fields [9], Gebhart [11], and Yaksh et al. [49]).
The microinjection of the μ-opioid receptor agonist morphine in microgram doses into the PAG induces an analgesia that is attenuated by transection of spinal pathways, suggesting that morphine acting in the PAG may induce a descending inhibition in the spinal cord. Morphine given systemically or microinjected into brainstem sites modulates spinal reflexes, evokes the release of monoamines from the spinal cord, and reduces nociceptive responses of dorsal horn neurons (Figs. 11-2 and 11-3). Microinjection of morphine at PAG sites is mimicked by microinjection of excitatory amino acids and by focal electrical stimulation. These supraspinally induced effects are reduced significantly by spinally administered monoamine receptor antagonists (19). Morphine may activate antinociceptive output neurons in the PAG by inhibiting GABAergic interneurons that tonically inhibit output neurons (disinhibition). An activation of descending inhibitory systems by systemic or supraspinal morphine has been directly shown by some authors, albeit not by others. The α4 nicotinic receptor agonist epibatine acts on dorsal raphe nucleus but not adjacent PAG to induce antinociception (6). The nicotine-induced antinociception is reduced in mice lacking the preproenkephalin gene (3), indicating that the endogenous opioid system is involved. In rostroventral medulla two distinct types of neurons may modulate spinal nociception. ON-cells are believed to facilitate and OFF-cells depress spinal nociception. μ– and δ-opioid receptor agonists activate OFF cells and may thereby exert their antinociceptive effect (14).
DEEP BRAIN STIMULATION IN HUMANS FOR PAIN RELIEF
Stimulation of deep brain structures was introduced into medical practice (1,16) following the promising experimental observation that focal electrical stimulation in PAG produced powerful antinociception in animals. In studies on humans, profound and long-lasting analgesia was achieved in some patients, whereas others did not benefit from deep brain stimulation. Stimulation in the thalamus, the PAG, the mesencephalic reticular formation, and rarely in the hypothalamus has been applied to patients suffering from various chronic nociceptive, neuropathic, or mixed pain conditions. The results differ markedly between centers, but in a recent survey the average success rate was reported to be 50%. The development of tolerance to stimulation of brain sites, crosstolerance with morphine, and the reversal of stimulationproduced analgesia by naloxone suggest that the analgesic effect of deep brain stimulation is in part mediated by endorphins.
In conclusion, antinociceptive neurons have been identified at numerous brainstem sites that are also implicated in a wide variety of nonsensory functions. A dense network of connections exists between these antinociceptive nuclei and other areas of the brain, which is in line with the fact that analgesia and hypoalgesia are often part of complex behavioral patterns such as acute stress responses or sleep-wake cycles. This limits the use of analgesic deep brain stimulation to those sites with few and tolerable side effects.

Full access? Get Clinical Tree
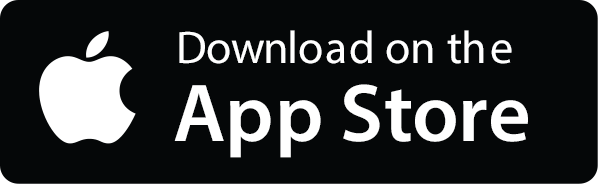
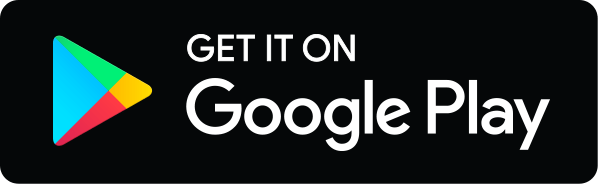