Chapter 159 Many airborne toxins produce local noxious effects on the airways and lungs as irritants. The respiratory tract can also serve as a portal of entry for systemic poisons as simple or systemic asphyxiants. Inhalational exposure can be covert and indolent (as in occupational exposure to asbestos or urban exposure to photochemical smog) or fulminant and obvious. The circumstances and location of the exposure, the presence of combustion or odors, and the number and condition of victims assist in the diagnosis. Despite the array of possible toxic inhalants, identification of a specific inhalant is generally unnecessary because therapy is based primarily on the clinical manifestations (Table 159-1). Table 159-1 Most simple asphyxiations are workplace related and usually occur during the use of liquefied gas while the employee is breathing through an airline respirator or working in a confined space.1 Since the advent of catalytic converters, most deaths from the intentional inhalation of automotive exhaust result from simple asphyxiation, due to hypoxia, and not from carbon monoxide (CO) poisoning.2 Acute effects occur within minutes of onset of hypoxia and are the manifestations of ischemia. A fall in the FIO2 from normal, 0.21 (i.e., 21%), to 0.15 results in autonomic stimulation (e.g., tachycardia, tachypnea, and dyspnea) and cerebral hypoxia (e.g., ataxia, dizziness, incoordination, and confusion). Dyspnea is not an early finding because hypoxemia is not as potent a stimulus for this sensation, or for breathing, as are hypercarbia and acidosis. Lethargy from cerebral edema is expected as the FIO2 falls below 0.1 (10%), and life is difficult to sustain at an FIO2 below 0.06 (6%).3 Because removal from exposure terminates the simple asphyxiation and allows restoration of oxygenation and clinical improvement, most patients present with resolving symptoms. However, failure to improve suggests complications of ischemia (e.g., seizures, coma, and cardiac arrest) and is associated with a poor prognosis. Irritant gases dissolve in the respiratory tract mucus and alter the air-lung interface by invoking an irritant or inflammatory response. When these gases are dissolved, most of them produce an acid or alkaline product, but several generate oxygen-derived free radicals that produce direct cellular toxicity (Fig. 159-1). The clinical effects of pulmonary irritants can be predicted by their water solubility (see Table 159-1). Highly water-soluble gases have their greatest impact on the mucous membranes of the eyes and upper airway. Exposure results in immediate irritation, with lacrimation, nasal burning, and cough. Although their pungent odors and rapid symptom onset tend to limit significant exposure, massive or prolonged exposure can result in life-threatening laryngeal edema, laryngospasm, bronchospasm, or acute respiratory distress syndrome (ARDS) (formerly known as noncardiogenic pulmonary edema).4 Poorly water-soluble gases do not readily irritate the mucous membranes at low concentrations, and some have pleasant odors (e.g., phosgene’s odor is similar to that of hay). Because there are no immediate symptoms, prolonged breathing in the toxic environment allows time for the gas to reach the alveoli. Even moderate exposure causes irritation of the lower airway, alveoli, and parenchyma and causes pulmonary endothelial injury after a 2- to 24-hour delay. Initial symptoms consistent with acute respiratory distress syndrome may be mild, only to progress to overt respiratory failure and acute respiratory distress syndrome during the ensuing 24 to 36 hours.5 Gases with intermediate water solubility tend to produce syndromes that are a composite of the clinical features manifested with the other gases, depending on the extent of exposure. Massive exposure is most often associated with rapid onset of upper airway irritation and more moderate exposure with delayed onset of lower airway symptoms.6 Bronchospasm, cough, chest tightness, and acute conjunctival irritation frequently follow allergen exposure, but the history generally suggests the diagnosis. ARDS occurs after many physiologic insults, including trauma and sepsis, highlighting the need for accurate history taking.5 Bronchospasm generally responds to inhaled beta-adrenergic agonists; the role of ipratropium is not yet defined. Other than as a standard treatment of a comorbid condition, such as asthma, there is no clear indication for corticosteroids.7 Patients exposed to chlorine or hydrogen chloride gas receive symptomatic relief from nebulized 2% sodium bicarbonate solution.6 Because the inflammatory cascade is not altered, however, the component of lung injury mediated by free radicals probably continues and causes delayed deterioration. Patients receiving inhalational bicarbonate therapy require extensive discharge instructions for signs and symptoms of pulmonary irritation or admission to the hospital. Most smoke-associated morbidity and mortality relate to respiratory tract damage. Thermal and irritant-induced laryngeal injury may produce cough or stridor, but these findings are often delayed. Soot and irritant toxins in the airways can produce early cough, dyspnea, and bronchospasm. Subsequently, a cascade of airway inflammation results in acute lung injury with failure of pulmonary gas exchange. The time between smoke exposure and the onset of clinical symptoms is highly variable and dependent on the degree and nature of the exposure. Singed nasal hairs and soot in the sputum suggest substantial exposure but are neither sufficiently sensitive nor specific to be practical.8 Early death is caused by asphyxia, airway compromise, or metabolic poisoning (e.g., CO). Airway patency should be evaluated early. If evidence of significant airway exposure is present, such as carbonaceous sputum or hoarse voice, the airway should be examined by direct or fiberoptic laryngoscopy. Simply observing the patient for deterioration can result in airway compromise requiring rapid and, by then, very difficult airway intervention. Signs of alveolar filling or hyperinflation on chest radiography, abnormal flow-volume loop or diffusing capacity for CO on pulmonary function testing, or abnormal distribution and clearance of radiolabeled gas on ventilation scans can help predict lower airway injury.9 Metabolic acidosis, particularly when it is associated with a serum lactate level greater than 10 mmol/L, suggests concomitant cyanide poisoning.10 Oxygenation should be assessed by co-oximetry because blood gas analysis and pulse oximetry may be inaccurate in CO-poisoned patients (see later).
Inhaled Toxins
INHALANT
SOURCE OR USE
PREDOMINANT CLASS
Acrolein
Combustion
Irritant, highly soluble
Ammonia
Fertilizer, combustion
Irritant, highly soluble
Carbon dioxide
Fermentation, complete combustion, fire extinguisher
Simple asphyxiant; systemic effects
Carbon monoxide
Incomplete combustion, methylene chloride
Chemical asphyxiant
Chloramine
Mixed cleaning products (e.g., hypochlorite bleach and ammonia)
Irritant, highly soluble
Chlorine
Swimming pool disinfectant, cleaning products
Irritant, intermediate solubility
Chlorobenzylidene malononitrile (CS), chloroacetophenone (CN)
Tear gas (Mace)
Pharmacologic irritant
Hydrogen chloride
Tanning and electroplating industry
Irritant, highly soluble
Hydrogen cyanide
Combustion of plastics, acidification of cyanide salts
Chemical asphyxiant
Hydrogen fluoride
Hydrofluoric acid
Irritant, highly soluble; systemic effects
Hydrogen sulfide
Decaying organic matter, oil industry, mines, asphalt
Chemical asphyxiant; irritant, highly soluble
Methane
Natural gas, swamp gas
Simple asphyxiant
Methylbromide
Fumigant
Chemical asphyxiant
Nitrogen
Mines, scuba diving (nitrogen narcosis, decompression sickness)
Simple asphyxiant; systemic effects
Nitrous oxide
Inhalant of abuse, whipping cream, racing fuel booster
Simple asphyxiant
Noble gases (e.g., helium)
Industry, laboratories
Simple asphyxiant
Oxides of nitrogen
Silos, anesthetics, combustion
Irritant, intermediate solubility
Oxygen
Medical use, hyperbaric conditions
Irritant, free radical; systemic effects
Ozone
Electrostatic energy
Irritant, free radical
Phosgene
Combustion of chlorinated hydrocarbons
Irritant, poorly soluble
Phosphine
Hydration of aluminum or zinc phosphide (fumigants)
Chemical asphyxiant
Smoke (varying composition)
Combustion
Variable, but may include all classes
Sulfur dioxide
Photochemical smog (fossil fuels)
Irritant, highly soluble
Simple Asphyxiants
Clinical Features
Pulmonary Irritants
Principles of Disease
Clinical Features
Diagnostic Strategies and Differential Considerations
Management
Smoke Inhalation
Clinical Features
Diagnostic Strategies and Differential Considerations

Full access? Get Clinical Tree
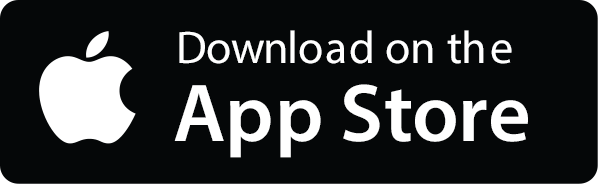
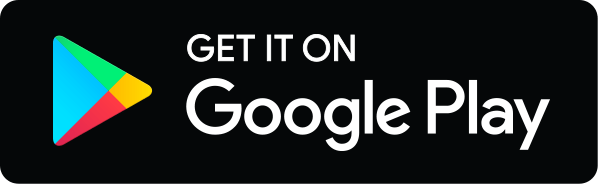