Anesthesiologists use many potent drugs either as single agents or in combination. Each of these drugs has a unique profile in terms of how their concentrations and effects change over time and how they interact with other drugs. Although the basic features of anesthetic drugs are well established, the time course of how the drugs behave, especially in combination with other drugs, is complex and difficult to predict. Anesthesiologists rely on experience and training to formulate dosing regimens, yet they can be confounded by the dynamic changes encountered in the operating room and intensive care unit.
Numerous laboratory investigations have characterized important aspects of drug behavior that are of interest to anesthesiologists. These discoveries often involve complex mathematical formulas and, until recently, have been largely confined to drug package inserts, textbooks, and published manuscripts. With the advent of personal computers and hand-held devices powerful enough to process solutions to these mathematical formulas, models can be used to simulate drug behavior real time and at the point of care.
As a translational tool from laboratory to point of patient care, simulation can be used to visualize drug concentrations and effects over time and how drug combinations influence various drug effects. Figures 4–1 and 4–2 present an example of how simulation illustrates clinical effects of interest. This simulation assumes a 47-year-old, 100-kg, 183-cm male with a history of painful cholelithiasis and anorexia undergoing a laparoscopic cholecystectomy. Propofol, fentanyl, and succinylcholine are used for induction, and sevoflurane, fentanyl, and rocuronium are used for maintenance of anesthesia. Figure 4–1 presents the dosing regimen and the predicted drug effect-site concentrations, and Figure 4–2 presents the predicted drug effects.
Figure 4–1
Simulation of drug effect-site concentrations for a combined anesthetic technique using sevoflurane, fentanyl, succinylcholine, and rocuronium. The top section presents the dosing timeline for a 60-minute procedure. Intravenous bolus doses of fentanyl, propofol, succinylcholine, and rocuronium are presented as colored vertical lines. Sevoflurane vaporizer settings are presented in orange. Using published models,1, 2, 3, 4, 5, 6, 7, 8, 9, 10, 11, 12, 13, 14, 15, and 16 predicted effect-site concentrations (Ce) over time were estimated for each drug and are presented in the 3 plots below the dosing time line: A for sedatives, B for analgesics, and C for neuromuscular blockers. The orange, black, and purple lines represent the Ce values for propofol, sevoflurane, and fentanyl. The dashed black line represents the end tidal sevoflurane concentration. The orange solid and dashed lines represent the Ce values for succinylcholine and rocuronium.

Figure 4–2
Predicted drug effects over time. The top section presents the dosing timeline presented in Figure 4–1. Combined effect of propofol, sevoflurane, and fentanyl are presented as a filled-in plot (green), and the effect of individual anesthetics are presented as black lines. Using published interaction models,17, 18, and 19 predicted effects include A, loss of responsiveness; B, loss of response to a moderately painful stimulus for fentanyl alone and in combination with sevoflurane; C, loss of response to a moderately painful stimulus for sevoflurane alone and in combination with fentanyl; and D, loss of train-of-four for succinylcholine and rocuronium. Loss of response to a painful stimulus was defined as a loss of response to 30 pounds per square inch of anterior tibial pressure, a surrogate of pain experienced in the recovery room following laparoscopic procedures.18

This example uses several types of models. Pharmacokinetic models are used to predict both plasma and effect-site concentrations. For intravenous agents, models use weight and in some cases age to make predictions. For inhalation agents, models use weight, minute ventilation, cardiac output, and fresh gas flow to make predictions. Pharmacodynamic interaction models use predicted effect-site concentrations to estimate selected drug effects. There are many drug interaction models available, each with unique drug interactions and dose-response relationships. Some examples include probability of moderate sedation, ventilatory depression, loss of response to esophageal instrumentation, etc. See Chapter 3 for a complete list; only a few are presented in Figure 4–2. These simulations illustrate several important points.
Each anesthetic drug has a unique kinetic profile. This can be appreciated by comparing the rate of drug dissipation following the propofol, fentanyl, succinylcholine, and rocuronium boluses. Of these drugs, fentanyl has the slowest rate of decline whereas propofol has the fastest. It is important to recognize that the rate of drug dissipation does not directly correlate to the duration of effect. It is simply a description of anesthetic drug kinetics. Duration of effect is dependent on drug effect-site concentration and interactions with other anesthetic drugs.
Changes in effect-site concentrations lag behind changes in end-tidal concentrations for inhaled agents and behind changes in plasma concentrations for intravenous agents. This is especially important under non–steady-state conditions (ie, induction and emergence). In this example, after induction, sevoflurane is titrated to maintain unresponsiveness (between 1.5 and 2.5 vol%). The time course of end-tidal and effect-site concentrations is presented as dashed and solid lines in Figure 4–1A. The sevoflurane effect site lags behind end-tidal concentrations. With conventional ventilation (minute volume of 6 L/min), normal cardiac output, and normal fresh gas flow (2 L/min), the time required for effect-site concentration to be near the end-tidal concentration (pseudo–steady-state) can be up to 14 minutes.
Using effect-site concentration models make predictions of effect. For potent inhaled agents, anesthesiologists have a working understanding of drug effect as a function of drug concentration. For example, end-tidal sevoflurane concentrations are used to estimate drug effect in terms of minimum alveolar concentration (MAC) (2.2 vol%), MACbar (3 vol%), and MACawake (0.6–1.0 vol%). For intravenous agents, anesthesiologists’ working understanding of drug concentration versus effect is not as well developed. This is because with intravenous agents, there is no presentation of drug concentration to associate with drug effect as there is with potent inhaled agents.
In these simulations, the concentration versus time profile for each drug is presented in graphical form along with a prediction of drug effect. For example, the 2 mg/kg propofol dose led to a predicted peak effect-site concentration of 5 mcg/mL (see Figure 4–1A). This resulted in a high (> 90%) probability of unresponsiveness within 90 seconds that lasted for 3 minutes (see Figure 4–2A).
As dosed, the probability of sedation–hypnosis between the induction with propofol and the administration of sevoflurane remains greater than 95%. As propofol’s effect dissipates, the onset of effect from sevoflurane increases (see arrows in Figure 4–2A). This combined effect is dependent upon the time lapse between the administration of propofol and sevoflurane. In this simulation, it is 2.5 minutes. Longer delays may lead to brief gaps in the sedative–hypnotic effect.
The induction dose (2 mcg/kg) of fentanyl reached a peak effect-site concentration of 2.2 ng/mL within 5 minutes and then dissipated over the next 30 to 40 minutes (see Figure 4–1B). This bolus was associated with greater than a 50% probability of analgesia (defined as a loss of response to a moderately painful stimulus) for 15 minutes. The fentanyl dose near the end of the procedure (1 mcg/kg) yielded a peak effect-site concentration of 1.6 ng/mL. This bolus was less effective with only a brief period (2 to 3 minutes) where the probability of analgesia is above 50%.
The induction dose (1 mg/kg) of succinylcholine reached a peak effect-site concentration of 1.1 mcg/mL (see Figure 4–1C). This provided a probability greater than 95% of no train-of-four for 6 minutes. Because of the short procedure time, a small dose of rocuronium (0.3 mg/kg) was administered. It provided an additional 10 minutes of no train-of-four (see Figure 4–2D).
Perhaps the most important feature of this simulation is visualization of anesthetic drug interactions. Even with a routine anesthetic, several pharmacodynamic characteristics are not always easy to predict. Experienced anesthesiologists have a sense of onset and duration of action for most, if not all, of these drugs when administered alone and may, at first glance, find drug display technology unnecessary. But even experienced clinicians may find it difficult to predict the onset and duration of a simple bolus of fentanyl when coadministered in the presence of other anesthetic drug. For example, clinicians may find it difficult to predict the duration of analgesia for a 1 mcg/kg fentanyl bolus in the presence of 2 vol% sevoflurane. Or they may have difficulty predicting the duration of unresponsiveness when the same bolus is administered just before adjusting the sevoflurane vaporizer from 2% to 0% at the end of an anesthetic. Low-dose opioids can substantially prolong emergence from an inhalation agent, even at concentrations well below MAC.
Not all drug–drug interactions are alike. For example, sevoflurane provides the majority of the sedative–hypnotic effect with a minor contribution from fentanyl. If no fentanyl were administered, there would likely be no substantial difference in loss of responsiveness during the anesthetic. If no sevoflurane were administered, the patient would likely remain awake. When combined, sevoflurane and fentanyl interact to provide a slightly more pronounced loss of responsiveness. This interaction is best visualized during emergence, where the fentanyl slightly prolongs the return to responsiveness (see Figure 4–2A).
By contrast to loss of responsiveness, both fentanyl and sevoflurane have analgesic properties, and when combined they produce a profound analgesic effect. As dosed, fentanyl alone provides analgesia for a brief period of time (probability > 50% for 15 minutes at induction) but is inadequate to meet the analgesic requirements for the entire procedure (see Figure 4–2B). Sevoflurane at 2 vol% alone provides analgesia for the entire procedure (see Figure 4–2C). When combined, the probability of analgesia to a moderate stimulus is greater than 99% for the entire 60-minute anesthetic. The impact of drug synergism may again be best visualized once the anesthetic is terminated. With both drugs, the duration of analgesia after the anesthetic is terminated is much longer than with either drug alone.
Simulation allows visualization of a dosing regimen prior to administering it. Figure 4–3 presents a drug display rendition of induction with propofol, and fentanyl followed by maintenance with sevoflurane and intermittent boluses fentanyl. The display provides prediction that span from 30 minutes in the past to 10 minutes into the future. This feature provides the ability to explore various dosing regimens prior to the end of an anesthetic. In this example, the 10-minute time window into the future is labeled Plan A. With that plan, the influence of a 100-mcg fentanyl bolus on analgesia and sedation is well visualized. Other plans might explore the behavior of hydromorphone or sufentanil in a similar fashion and provide additional information before an opioid is selected and administered. Similar explorations of different sevoflurane vaporizer settings can be made to identify doses that account for the addition of an opioid on the time to reach a high probability of return of consciousness. If helpful, time boundaries can also be modified to visualize predicted drug effects into the future longer than 10 minutes. This may be useful for longer acting anesthetics, where accounting for drug accumulation and associated prolonged effect may be difficult to predict.

Full access? Get Clinical Tree
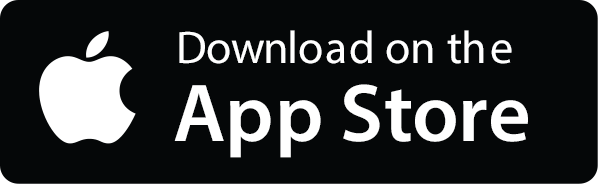
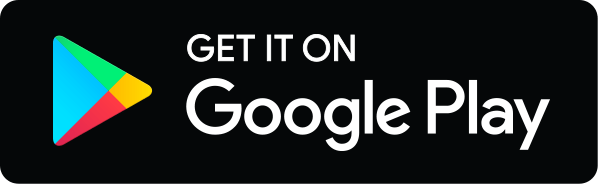