Adverse physiologic changes in obese patients (Table 20–1) present multiple challenges in the operating room, including intravenous line placement, airway management, positioning, surgical exposure, and blood glucose control.10 Perhaps most important is determining the appropriate dose of anesthetic, as it will impact airway management and extubation and pain management. Although manufacturer’s dosing recommendations are weight-normalized (mg/kg), obese patients are often excluded from clinical trials during drug development.9 Anesthesiologists rarely use total body weight (TBW) when programming an infusion pump or administering an induction dose for fear of an overdose; they often use something less than TBW to get “close enough” to the desired effect while avoiding significant adverse effects.11,12
Organ System | Adverse Effects |
---|---|
Cardiovascular | Increased vascular volume and cardiac output1,2 and 3 Decreased myocardial compliance3 Hypertension and left ventricular hypertrophy1 |
Respiratory | Decreased lung compliance and functional residual activity4 Restrictive lung disease5 Rapid oxygen desaturation following apnea4 Increased risk of sleep apnea6 High airway pressures required to achieve adequate ventilation4 |
Airway | Increased likelihood of difficult ventilation and tracheal intubation6 |
Hepatic | Minimal effect on drug metabolism. Drug clearance: variable cytochrome P450 enzyme activity with altered drug binding to α1 glycoprotein but not albumin7,8 |
Renal | Glomerular filtration and creatinine clearance may exhibit no change, an increase, or decrease8,9 |
A popular method of administering intravenous anesthetics is as a continuous infusion. To improve the accuracy of infusion delivery, computerized infusion pumps that use pharmacokinetic models have been developed to quickly achieve and maintain desired target effect-site concentrations. This delivery technique, known as target-controlled infusions (TCI), has become popular worldwide. Unfortunately, neither have models used to drive TCI been validated in obese patients nor has agreement regarding the correct weight to input been identified. Most models were developed with normal-sized patients or volunteers and extrapolated for use in larger patients. The main limitation is that the composition of normal size and obese patients is not the same; hence, kinetic model predictions are likely to be less accurate in the obese.
To address these issues and others, clinical pharmacologists have put forth various “scaled weights” for dosing anesthetics and recommended modified or improved kinetic models to drive TCI pumps for selected anesthetics (ie, propofol and remifentanil). For most anesthetics, however, dosing recommendations are extrapolated and not based on studies in obese patients, making them difficult to reliably use.
A simple approach when formulating a dose in obese patients is to use TBW. This approach assumes that the volume of distribution and clearance are the same in all patients, lean or obese.11 This holds true if drugs are highly soluble and distributed throughout all tissue and if drug clearance is the same regardless of body habitus. For anesthetics, the assumptions of ubiquitous drug distribution and clearance regardless of body habits are wrong,1 and dosing to TBW can often lead to a significant overdose.
To get beyond the limitations of TBW, various estimates of body habitus have been adapted for dosing anesthetic drugs.1,2,9,10 Some of these include ideal body weight (IBW), lean body weight (LBW), and fat-free mass (FFM). Each makes assumptions and has limitations (Table 20–2), but none enjoys applicability across all anesthetic drugs. Table 20-3 presents dosing weights for lean and obese females, both 155 cm tall, using various dosing scalars. A key point is that using many of these scalars, especially LBW, may substantially under dose anesthetics.
Name | Equations | Advantages | Disadvantages |
---|---|---|---|
Ideal body weight | Male: 50 kg + 2.3 kg for each 2.54 cm (1”) over 152 cm (5 ft) Female: 45.5 kg + 2.3 kg for each 2.54 cm over 152 cm | Accounts for gender and height | May under dosea Does not account for body habitusb |
Lean body mass | Male: 1.1 × TBW – 128 × (TBW/Ht)2 Female: 1.07 × TBW – 148 × (TBW/Ht)2 | Accounts for gender and body habitus (height and weight) | May under dosea For a BMI > 35 kg/m2, LBM becomes smaller than for lower BMIs |
Fat-free mass13 | Male: (9.27 × 103 × TBW)/(6.68 × 103 + 216 × BMI) Female: (9.27 × 103 × TBW)/(8.78 × 103 + 244 × BMI) | Accounts for gender and body habitus (height and weight) | May underdosea |
Pharmacokinetic mass14,15 | 52/[1 + (196.4 · e-0.025 TBW – 53.66)/100] | Correlates well with measured fentanyl concentrations | Does not account for gender or height |
Modified fat-free mass16,17 | FFM + 0.5*(TBW – FFM) | Accounts for gender and body habitus (height, LBW, and adipose weight). | Empirically derived |
Besides under dosing, LBW has an additional issue. As TBW approaches morbid obesity, LBW starts to decrease in size (Figure 20–1) for 2 reasons: (1) estimates of LBW are based on a quadratic equation fit to old population height–weight data, when morbid obesity was rare, and (2) there was little need to predict LBW for a body mass index (BMI) greater than 45 kg/m2.
Figure 20–1
Predictions of scaled weight based on lean body mass, fat-free mass,13 modified fat-free mass,16,17 ideal body weight, and pharmacokinetic (PK) mass14,15 are presented for female and male individuals of various heights over a range of total body weights. At a total body weight of 90 kg for the female and 130 kg for the male, the lean body mass predictions start to decrease for increasing total body weight represented with a dashed blue line. The ideal body weight remains the same regardless of total body weight (red line). PK mass remains the same regardless of height and gender (black line).


To achieve a more appropriate (larger) dosing weight, authors have also put forth dosing scalars that not only account for the lean or fat free mass, but a component of fat mass as well.18,19 Methods have been developed to estimate normal and excessive amounts of fat. Dosing weights for patients with a BMI greater than 30 kg/m2 have been devised that include lean body and normal fat mass.19
Empirical approximations of this approach have been successfully used to dose anesthetics.16,17 One approximation used by Cortinez et al, modified fat-free mass (MFFM), is defined as FFM + fat mass × (TBW – FFM), where fat mass is a parameter that accounts for the different types of fat with a range from 0 (some combination of normal and excessive fat) to 1 (normal fat only). Prior work has used a fat mass of 0.4 to dose propofol in obese patients16 but is likely to vary for different anesthetic drugs and the extent of obesity. For simulation purposes, the fat mass will be assumed to be 0.5.
Propofol is difficult to characterize in obese patients for several reasons. Plasma propofol concentrations are highly sensitive to cardiac output20; obese individuals often have an increased cardiac output compared to their lean counterparts.1 In terms of distribution, propofol is lipophilic and rapidly moves from the plasma to the peripheral tissues,10 suggesting that distribution is a function of body habitus (ie, with more adipose tissue, more propofol can leave the plasma). Propofol clearance, unlike distribution, is independent of body habitus and correlates well with TBW.16 Both distribution and clearance influence drug concentration changes over time; distribution influences concentration peaks with bolus dosing, and clearance influences concentrations during and following infusions. Authors have put forth recommendations consistent with these observations to include LBM21 for induction and TBW or MFFM for infusions.16,22 One concern with using TBW to drive propofol infusions in obese patients is drug accumulation that may lead to prolonged drug effect and delayed emergence. Prior work using TBW compared to other scaled weights has not supported this concern.16
Simulations of a bolus followed by an infusion using various weight scalars (Table 20–3) are presented in Figure 20–2. The simulations present the predicted propofol effect-site concentrations from a bolus (2 mg/kg) and 1-hour infusion (150 mcg/kg/min) in a 155 cm (5’1”) obese (140 kg) and lean (60 kg) female. If dosed according to TBW, plasma concentrations in a lean and obese individual are similar; their respective peak propofol concentrations are approximately 7 and 8 mcg/mL (gray and black lines). The probability of unresponsiveness is also similar during the bolus and infusions, but the obese individual has a prolonged duration of effect once the 1-hour infusion is terminated. The time required to reach less than a 5% probability of unresponsiveness once the infusion is terminated is 18 and 26 minutes for a lean and obese individual, respectively.
Figure 20–2
(A) Simulation of propofol effect-site concentrations (Ce) that result from a bolus (2 mg/kg) and 1-hour infusion (150 mcg/kg/min) for a 53-year-old 155-cm (5’1”) female. (B) Simulation of probability of unresponsiveness after a 2 mg/kg bolus and 1 hour infusion (150 mcg/kg/min) for a 53-year-old 155-cm (5’1”) female. Simulations include 2 dosing weights: (1) total body weight (TBW) of 60 kg with a body mass index (BMI) of 25 kg/m2 and (2) 140 kg with a BMI of 58 kg/m2. Simulations also include several weight scalars for the 140-kg weight: FFM, fat-free mass; MFFM, modified fat-free mass; LBM, lean body mass; and IBW, ideal body weight. Estimates of propofol Ce levels were made using pharmacokinetic parameters published by Cortinez et al.17 Predictions of loss of responsiveness were made using the pharmacodynamic model published by Kern et al.23

Of note, dosing according to IBW, LBM, and FFM all lead to effect-site concentrations that are substantially lower than those dosed to TBW and are likely to yield inadequate effect, especially during the infusion. The most worrisome of these 3 weight scalars is LBM. The obese patient from Table 20-3 dosed using LBM would be given an induction dose of 2 mg/kg, or 58 mg, in contrast to the induction dose of 120 mg given to her lean counterpart.
A reasonable dosing scalar for propofol is the MFFM. When using this scalar, plasma concentrations are similar but somewhat lower to what is achieved when dosing a lean individual to TBW (green versus gray lines in Figure 20–2). Following the bolus dose, the probability of unresponsiveness does drop below 80%, making this dosing scalar perhaps unattractive if propofol is used as the sole anesthetic.
Several kinetic models of propofol are available for TCI and have been used with obese patients with varied results. Perhaps the 2 most widely used models are those published by Marsh et al and Schnider et al.24,25 The Marsh model was built from data collected in a pediatric population. Although it is useful, it may have limited application in obese patients. Using the Marsh model, researchers have explored different dosing weights for propofol in morbidly obese patients when coadministered with remifentanil.22,26 Results have been variable. Although TCI using TBW may better predict measured plasma concentrations in this population than the corrected weight used by Servin et al,16 authors recommend that TCI using the Marsh model should be titrated to target processed electroencephalogram (EEG) values22 in this patient group regardless of weight is used.
The Schnider model was built from data collected in adults over a range of weights, heights, and age, but it did not specifically include obese patients. It uses LBM as described above and so may have limited application in morbidly obese patients.27 By way of comparison, researchers have explored dosing requirements needed for induction of anesthesia using either the Marsh or the Schnider model on morbidly obese patients. They concluded that either model works when dosing to TBW but that the target concentrations should be different for each model; they reported a target effect-site concentration to achieve a 95% probability of effect of 4.2 and 5.5 mcg/mL for the Marsh and Schnider models, respectively.28
Cortinez et al developed a model based on measured propofol concentrations in obese patients specifically designed to administer propofol TCI.17 The authors took advantage of an international data repository called “Open TCI” (http://www.opentci.org) to build a model using propofol concentrations from a wide range of body weights. They published model parameters that scaled normal size to obese individuals using an empirically derived formula. The formula uses TBW and accounts for differences in distribution volumes and clearances in obese patients; TBW is divided by a standard patient size (70 kg) and raised to the power of 1 for distribution volumes and by the power of 0.75 for clearances.
A simulation of 90-minute propofol target controlled infusion using each of these models17,24,25 is presented in Figure 20–3 for a 155-cm, 140-kg female. Of note is the difference in the total amount of propofol delivered using each model to maintain a plasma propofol concentration at 4 mcg/mL. Driving the infusion with the Cortinez model used less propofol than the others. The difference is substantial—up to 374 mg less propofol over 90 minutes—indicating that model choice can impact clinical performance of TCIs. By comparison, a 2-mg/kg bolus followed by a 150-mcg/kg/min infusion dosed to TBW for 90 minutes requires 2170 mg, yielding predicted effect-site concentrations just above 4 mcg/mL, a total dose similar to what is delivered when using the Marsh model to drive the TCI. Each model also makes different predictions about how quickly propofol plasma concentrations and drug effect (ie, loss of responsiveness) will dissipate. Once the infusion is stopped, the Schnider model predicts a rapid decline in propofol concentrations compared to the others.
Figure 20–3
Simulations of a 90-minute propofol target controlled infusion set to achieve and maintain a target effect-site concentration (Ce) of 4 mcg/mL using 3 different pharmacokinetic models for a 53-year-old 140-kg, 155-cm (5’1”) female, with a body mass index of 58 kg/m2. The pharmacokinetic models include parameters published by Marsh et al,24, Schnider et al,25 and Cortinez et al.17 The top panel presents the propofol infusion rates for each model. Not shown are the initial bolus doses, which include 1000 mg, 1200 mg, and 1300 mg for the Cortinez, Schnider, and Marsh models, respectively. The bottom panel presents the propofol effect-site concentrations as predicted by each model.

In summary, the Cortinez model may be best suited for TCI in this patient group. It was derived from data collected in obese patients and delivers less propofol by weight compared to other models. This model, however, is not yet available in many commercial TCI pumps, leaving clinicians to use techniques described above with the Marsh or Schnider models. It is important to remember that differences between models may be overshadowed by substantial interindividual variability and using any one of the three models may yield similar clinical results, especially when titrated to effect with processed EEG monitoring.
Unlike propofol, the behavior of many other sedatives (ie, etomidate, ketamine, barbiturates, and midazolam) is not as well characterized in obese people. One study, however, has compared midazolam pharmacokinetics in obese and normal size volunteers with interesting results.29 They found the volume of distribution, even when normalized to weight (ie, L/kg) was larger in obese patients. These results suggest that adipose tissue may take up more midazolam than other lean tissues. Hence, the volume of distribution of midazolam has a linear relationship with TBW; as patient size increases, so does the volume of distribution. They also found that the rate of drug elimination is the same in obese and lean volunteers. These results suggest that regardless of the amount of midazolam administered, the hepatic biotransformation of midazolam is fixed, meaning that the larger volume of drug distribution in the obese will require more time for elimination. However, despite the larger volume of distribution, peak plasma drug concentrations of midazolam and time to peak concentration were the same in obese and lean volunteers given the same dose.
Although these findings have not been clinically validated in obese patients, they may have important dosing considerations. Given that the volume of distribution was larger in the obese population, the authors concluded that bolus doses should be scaled to TBW (ie, 0.03 mg/kg). If other dosing scalars are used (ie, IBW), the authors postulated that midazolam may not achieve its desired effect (ie, anxiolysis or sedation). By contrast, since midazolam clearance is proportional to IBW, continuous infusion rates should use IBW for dosing purposes.29
It is well known that opioids cause worrisome respiratory depression and diminished ventilatory response to elevated PaCO2 levels in obese patients.30 With the high incidence of obstructive sleep apnea in this population, identifying the appropriate opioid dose is particularly relevant.31 Unfortunately, with the exception of remifentanil, minimal work has described the kinetic and dynamic behavior of opioids in obese individuals.
The kinetic profile of remifentanil is not substantially altered by body habitus, due to the metabolism of remifentanil by plasma esterases. The volume of distribution and clearance are similar in lean and obese patients. To illustrate these features, consider the simulations presented in Figure 20–4. Similar to the simulations with propofol above, these simulations presents the predicted remifentanil effect-site concentrations from a bolus (1 mcg/kg) and 1-hour infusion (0.15 mcg/kg/min) in two 155-cm (5′1″) females, one obese (140 kg), and one lean (60 kg). Again, for the obese individual, dosing is scaled by weights presented in Table 20–3.
Figure 20–4
(A) Simulation of remifentanil effect-site concentrations (Ce) that result from a bolus (1 mcg/kg) and 1-hour infusion (0.15 mcg/kg/min) for a 53-year-old 155-cm (5’1”) female. (B) Simulation of the probability of analgesia after a bolus (1 mcg/kg) and 1-hour infusion (0.15 mcg/kg/min) for a 155-cm (5’1”) female. Simulations include 2 dosing weights: total body weight (TBW) of (1) 60 kg with a body mass index (BMI) of 25 kg/m2 and (2) 140 kg with a BMI of 58 kg/m2. Simulations also include several weight scalars for the 140-kg female: FFM, fat-free mass; MFFM, = modified fat-free mass; LBM, lean body mass; and IBW, ideal body weight. Estimates of remifentanil Ce level were made using pharmacokinetic parameters published by Minto et al.32 Predictions of analgesia were made using a pharmacodynamic model published by Johnson.33 Analgesia was defined as loss of response to 30 pounds per square inch of pressure on the anterior tibia in healthy volunteers. Of note, predicted remifentanil Ce levels when dosed using pharmacokinetic mass were similar to those when dosed using MFFM.

If dosed according to TBW, what leads to reasonable plasma concentrations in a lean individual is excessive in an obese individual. For example, the peak concentrations are 5 and 11 ng/mL for the lean and obese individual (gray and black lines, respectively). Analgesic effect is also prolonged in the obese individual. Once the infusion has ended, the time required to reach less than a 5% probability of analgesic effect is 18 and 27 minutes for the lean and obese individual, respectively.

Full access? Get Clinical Tree
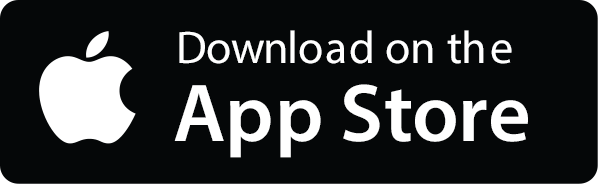
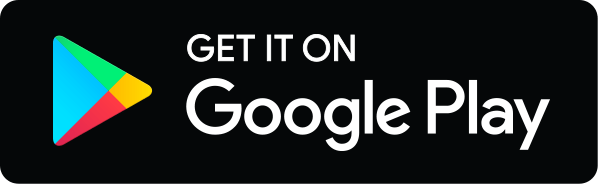