Circulatory dynamics in seven subjects at rest and during passive head-up tilt until the onset of (pre)syncopal symptoms, and return to the supine position. Values are mean and SE. * Different from rest.
With a moderate reduction of the CBV, mean arterial pressure (MAP) is maintained by peripheral resistance compensating for an approximately 20% reduction in cardiac output (CO).[1] As demonstrated during gravitational stress, MAP is stable at the level of the carotid baroreceptors because reduced distension of the carotid sinus elicits sympathetic excitation. In support, and as an extreme example, the approximately two-fold elevated BP of the giraffe [14] is related to the height of the animal, making its cerebral perfusion pressure similar to that of humans.
In addition, volume and/or pressure receptors within the central circulation that transmit through myelinated nerve fibers respond to a reduced CBV and initiate sympathetic activation. Enhanced sympathetic activity results not only in a relatively stable MAP but also in an elevated HR,[15] albeit with values typically being lower than 100 bpm (Figure 29.1). Yet values above 100 bpm are recorded occasionally (Figure 29.2), and the HR response to (central) hypo-volemia depends on age and does not always reach statistical significance.[16]
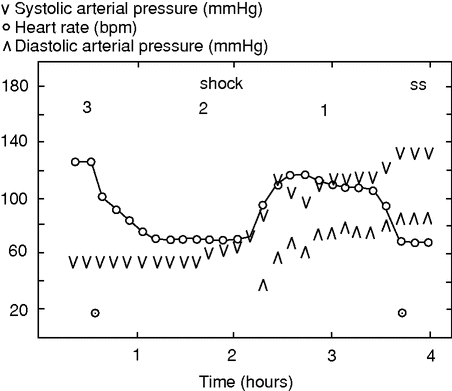
Heart rate and systolic and diastolic pressures during surgery for a ruptured abdominal aortic aneurysm. Stages 1–3 of shock indicated.
Stage II of hypovolemic shock
For volume treatment it is important that the second stage of hypovolemic shock represents a reversal of the autonomic response (Figure 29.1). Whereas sympathetic activation dominates the first stage, parasympathetic activity is prevalent during the second stage that is entered when CBV is reduced by 30%.[17] However, sympathetic activity to the adrenal gland is maintained, as identified by a progressive increase in plasma adrenaline.[18] In contrast, plasma nor-adrenaline reaches a plateau or decreases when central hypovolemia progresses to provoke cerebral hypo-perfusion with loss of consciousness. Reduced sympathetic activity is also reflected by muscle sympathetic activity [19] and an increase in muscle oxygenation and explains the fall in peripheral resistance that lowers MAP. The rise in plasma adrenaline is not important with regard to loss of vascular tone.[20]
Bradycardia
Parasympathetic activity is provoked by a significant reduction in CBV as indicated by plasma pancreatic polypeptide,[18,21] and there is usually a decrease in HR that is blocked by glycopyrron.[15] The bradycardia developed during central hypovolemia may be profound with no ECG activity detected on a monitor and, accordingly, hypovolemia should be suspected whenever “cardiac arrest” manifests in trauma patients, as in patients during and after surgery. Initiation of cardiac resuscitation, including external cardiac compression by applying pressure to the chest, besides positive-pressure ventilation to patients in hypovolemic shock further reduces CBV and could provoke an irreversible stage of shock.
Conversely, immediate restoration of CBV leads to recovery of both circulation and ventilation, within seconds, corresponding to the salutary effects of termination of passive HUT (Figure 29.1), LBNP, or pressure breathing, and indeed by providing ample volume to the patient in shock [21] (Figure 29.2). For surgical patients, therefore, cardiac resuscitation procedures may appear counterproductive unless it is verified that rapid volume infusion is without an effect. If it is not possible to administer such a volume immediately when the patient becomes ill, CBV can, at least partly, be restored by elevating the legs or placing the patient in Trendelenburg’s position. Only after such measures are found futile should a failing circulation be considered of cardiac origin, if not obvious from recording of the ECG.
Unfortunately the bradycardic response to hypo-volemia is not regularly included in textbook descriptions (for example Mair [22]). The texts seem to be based on observations derived from acute animal experiments rather than from observations in chronically instrumented conscious animals [7] or in humans.[23] Also it may seem “unreasonable” that vagal activity can be provoked by hemorrhage, but there are also beneficial effects of vagal activity under those circumstances. Vagal activity promotes hemostasis to an extent that it limits blood loss and, conversely, administration of atropine maintains bleeding and can, eventually, be fatal.[24] The second stage of hypovolemic shock may be seen as an attempt by the body to stop bleeding by lowering BP, at the same time as coagulation competence is enhanced by combined increase in vagal activity and plasma adrenaline concentration.
Yet, obviously, not all patients in hypovolemic shock present with a low HR. The bradycardic response to a significantly reduced CBV carries the prerequisite that efferent parasympathetic sinus node activation is intact, and that may not be the case for all patients, as exemplified by those suffering from atrial fibrillation or autonomic dysfunction, e.g. in consequence of diabetes mellitus. Of more general relevance to a surgical environment, vagal tone to the heart is overruled by pain-related sympathetic activity,[25] and many trauma patients are in pain due to crushed tissue. Also, ileus is associated with an elevated HR during hemorrhage.[26]
Pale skin and sympathetic activity
Besides the bradycardic response to stage II hypo-volemic shock, it is a characteristic for significant hemorrhage that the skin is pale, as can be observed during a vasovagal syncope, a condition that shares most if not all of the manifestations associated with stage II of hypovolemic shock. Perhaps the pale skin has inspired the notion that peripheral resistance is elevated in response to enhanced baroreceptor activity as the arterial pressure becomes low although peripheral resistance, as mentioned, decreases in reflection of ceased sympathetic activity.
Ceased sympathetic activity reflects that baroreceptor control of BP and HR is eliminated at this stage of shock.[27] Rather than being caused by sympathetic activity during (central) hypovolemia, pale skin reflects a marked (about 25-fold) increase in plasma vasopressin,[28] while a similar reduction in cutaneous blood flow by the increase in plasma angiotensin II is irrelevant to the appearance of the patient.[29] The marked increase in plasma vasopressin, together with lowering of plasma atrial natriuretic peptide (ANP) level, also explains the prolonged low urine production following hypovolemic shock and, conversely, conforms to maintained CO during surgery promoting diuresis. Similarly, cardiac afferent nerves inhibit gastric mobility,[30] which explains why maintained stroke volume of the heart (SV) during surgery reduces postoperative nausea and vomiting (PONV).[31]
The Bezold–Jarisch reflex
Collectively, bradycardia, low vascular resistance, increase in plasma vasopressin, etc., during hemorrhage confirms that a critically reduced CBV is characterized by responses similar to those described in the pharmacological literature as a Bezold–Jarisch reflex. However, it remains uncertain which afferent input elicits the reflex. Öberg and White [32] demonstrated the Bezold–Jarisch-like reflex by activation of unmyelinated nerve fibers from the left ventricle and suggested it to be provoked when the heart is emptied of blood. The second stage of hypovolemic shock is associated with only a 10–25% reduction in the diastolic filling of the heart,[33] and yet it remains possible that the most densely innervated apical part of the left ventricle is emptied by a significant reduction in CBV.
A concomitant reduction in HR and BP can, however, also be provoked by hemorrhage following cardiac denervation.[34] Therefore, the specific trigger for the reflex in response to hemorrhage remains in doubt, or it might vary depending on circumstances. The common and clinically relevant finding is that the reflex originates from the central circulation with a contribution from the central nervous system, as when a persons faints when a vein is cannulated.
Stage III of hypovolemic shock
Although stage II of hypovolemic shock may be fatal, there is also a third stage. If the reduction in HR in response to a low CBV is not a terminal event, HR increases again, typically to 120–130 bpm (Figure 29.2),[35] conforming to the tachycardia that most textbooks hold as a key feature of hypovolemic shock.[22] As demonstrated in animals, sympathetic activity is resumed during severe hemorrhage as indicated by the plasma catecholamine level.[26] It may be that cerebral ischemia, in consequence of prolonged hypotension and a low CO, is important for reactivation of sympathetic activity, and critically reduced cerebral perfusion could indicate that stage III represents a transition to an irreversible stage of shock.[12] However, in contrast to the common descriptions indicating an increase in total peripheral resistance during severe hemorrhage, total peripheral resistance decreases or does not change.[26]
Central vascular pressures during hypovolemic shock
Cardiovascular monitoring of critically ill patients is supplemented by recording of central vascular pressures. In experimental studies, central venous pressure decreases (Figure 29.1) together with mean pulmonary artery and wedge pressures with increasing levels of HUT or LBNP.[17,18]
For clinical evaluation of the circulation during progressive hypovolemia, however, it is a problem that reduction in central vascular pressures relates to the intervention rather than to the well-being of the subject. Although the pressure challenge (HUT or LBNP) may be established, the subject may faint at some later point not preceded by any specific change in central vascular pressure. During sustained HUT or LBNP, the reduction in CBV progresses with accumulation of fluid in the legs [36] and, consequently, CO also decreases, although there is a tendency for the pulmonary artery wedge pressure to increase.[37]
Stable “filling pressures” of the heart do not secure that CO is sufficient to maintain cerebral blood flow and oxygenation, and there are no data to support volume treatment based on central vascular pressure.[38] In fact, for patients, CO is not related to the filling pressures of the heart, although there is a relationship between CO and diastolic filling.[39]
Normovolemia
Patients need volume supplementation during anesthesia and in an intensive care setting, but the strategy remains debated both in regard to the amount that should be provided and to the preferred solutions. What seems established is that for surgery not associated with a significant blood loss, patients should be administered 1 liter of crystalloid.[5] Otherwise, it can be stated only that it is intuitively difficult to defend a volume treatment regime that keeps the patient hypovolemic or one that provides the patient with a volume overload, and yet there is no agreement on the volume load that defines “normovolemia.”
With the importance of CBV for circulatory shock, a definition of normovolemia seems desirable, not only to the patient in shock but also to patients throughout the perioperative period, and to patients in general. It appears important that monitoring of the circulation allows for intervention well before cerebral blood flow and oxygenation become affected, and evidence is provided for a volume administration strategy that is accurate within 100 ml.
Cerebral blood flow and oxygenation become affected by a blood loss corresponding to 30% of the (central) blood volume [40] or a blood loss of 1.0–1.5 liters. The proposed volume administration strategy thereby allows volume administration within approximately one-tenth of the volume loss that is significant for brain function.
The impact of a reduced CBV for SV, CO, and thus central or mixed (from the pulmonary artery) venous oxygen saturation (SvO2) offers monitoring modalities for evaluating the functional consequence of a reduced CBV.
Tilt table experiments
The influence of CBV on flow-related variables is readily illustrated during tilt table experiments. As mentioned, SV, CO, and thus SvO2 decrease during HUT, while maximal values are obtained during supine rest since, with the increase in central pressures and filling of the heart during the transition from supine to the head-down tilted position, there is no further increase in SV, CO, or SvO2,[37,41] and SV decreases only during extreme (90°) head-down tilt.[42]
Similarly, healthy non-fasting supine subjects are not volume-responsive with regard to SV.[43] Together, these observations indicate that for supine humans, maximal flow-related variables define nor-movolemia.
The surgical patient
In contrast to supine healthy subjects, the preoperative patient [3,4] and many patients under intensive care are volume-responsive. To supplement volume is important since any limitation to CO has consequences for all vascular beds, independent of an eventually large metabolic demand as exemplified by muscle blood flow during exercise.[44] Likewise, cerebral blood flow and oxygenation become affected even with the moderate reduction of CO that is associated with standing up.[40] Even more so, skin, muscle, and notably splanchnic and renal blood flow decrease in response to the elevated sympathetic activity provoked by a limited CBV and thereby CO. Conversely, a volume strategy that secures CO preserves not only splanchnic and renal flows of relevance for surgical healing and diuresis, respectively, but also for cerebral oxygenation, which is widely independent of MAP (Figure 29.3).[45] Thus, it seems evident that the primary focus of volume therapy is to prevent episodes of hypovolemia, and on-line monitoring of flow-related variables makes that possible, with consequences for postoperative complications.[31]
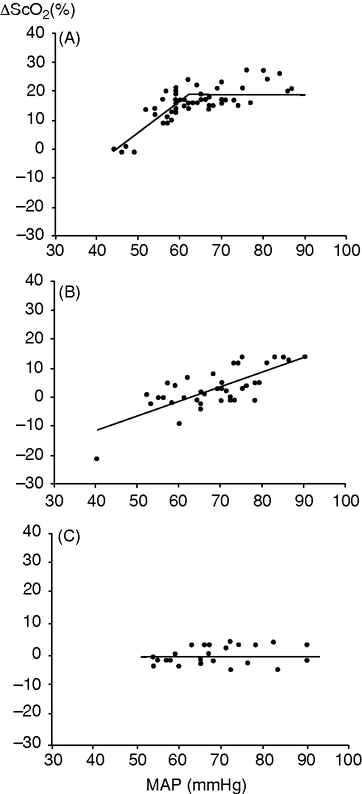
Changes in frontal lobe oxygenation (ScO2) and mean arterial pressure (MAP) during anesthesia. Illustrated are three patients, one of whom demonstrated a lower limit of cerebral autoregulation (A); for another no cerebral autoregulation was found (B); and for the third, no lower limit of cerebral autoregulation was detected (C).
Maintenance of cerebral oxygenation may require a MAP of 90 mmHg, probably because of arteriosclerosis in the vessels that serve the cerebral circulation. Monitoring of cerebral blood flow and/or oxygenation is advocated for older patients and for patients with vascular and/or cardiac disease, also considering that cerebral autoregulation might be compromised by the inhalation agents used for general anesthesia (Figure 29.3).
Evaluation of cerebral oxygenation is relevant especially to cardiac surgery during which the heart–lung machine determines CO. Maintaining cerebral oxygenation, e.g. by increasing the pump speed of the machine, reduces postoperative complications and secures mental well-being.[46] Similarly, maintained cerebral oxygenation is important for reducing complications following other types of surgery, and maintained cerebral oxygenation may be taken as an index for whether handling of the circulation has been adequate.[47]
Titration to establish normovolemia
A problem with directing volume treatment by flow-related variables is their individual variability. For example, the trained athlete has a low resting HR and a compensating large SV that makes it difficult to evaluate whether a given filling of the heart is sufficient to secure a maximal SV.
For CO and SvO2 the inter-individual variation is smaller, but there remain significant differences among subjects/patients, and only some of the variation can be explained. Notably, there are inter-individual differences in CO according to beta-adrenergic polymorphism, with the “Gly–Gly” carrying about a half liter per minute greater CO than the “Arg–Arg” phenotype.[48] In other words, there is a genetic background for why accurate volume administration based on flow-related variables should be individualized.[31] More importantly, however, CO (and thereby SvO2) varies depending on circumstances including type of anesthesia, temperature, and notably disease. Alternatively the volume regime that maintains the plasma (pro)ANP level may be evaluated retrospectively; in that case, it seems to require a surplus of 2.5 liters for major surgery when volume treatment is carried out with lactate Ringer’s solution.

Full access? Get Clinical Tree
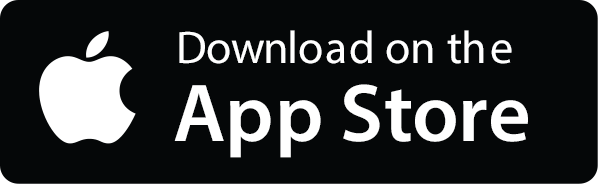
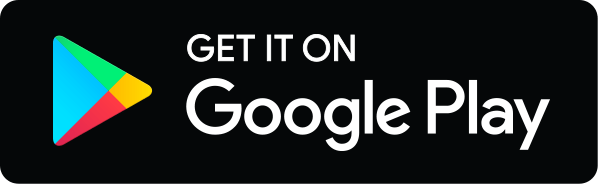