Hypoglycemia
John P. Mordes
Michael J. Thompson
David M. Harlan
Samir Malkani
Hypoglycemia occurs often in the intensive care unit (ICU) as both an admission diagnosis and a consequence of therapy. In a 2001 study of intensive insulin therapy in a surgical ICU population, hypoglycemia occurred in up to 5.1% of patients [1]. Another study of more than 2,200 ICU patients reported that nearly 7% experienced at least one episode of hypoglycemia [2]. Estimates of prevalence range as high as 25% [3]. Hypoglycemia is a marker of poor outcome in hospitalized patients in general [4,5,6], and among the critically ill in particular [7,8]. Severe, prolonged hypoglycemia can cause permanent neurological and cardiovascular damage.
Definition of Hypoglycemia
No specific blood glucose concentration defines hypoglycemia. Whipple proposed a symptom-based definition of hypoglycemia in the 1930s that remains valid [9]. “Whipple’s triad” defines hypoglycemia as (a) documentation of a low blood glucose concentration, (b) concurrent symptoms of hypoglycemia, and (c) resolution of symptoms after administration of glucose. Typically, this concentration in serum is less than 50 mg per dL (2.8 mM). The physiologic definition is a blood glucose concentration low enough to cause the release of counterregulatory hormones (e.g., catecholamines) and impair the function of the central nervous system (CNS).
Symptoms and Signs of Hypoglycemia
Counterregulatory hormones released in response to low glucose concentrations cause sympathoadrenal symptoms and signs. Prominent symptoms include hunger, tingling, shakiness, weakness, palpitations, and anxiety [10]. Corresponding signs include diaphoresis, tachycardia, peripheral vasoconstriction, and widening of the pulse pressure. These findings may be absent in patients receiving sympatholytic drugs (e.g., beta-blockers) and in patients with diabetes who have autonomic neuropathy.
Early symptoms and signs of neuroglycopenia include difficulty thinking and sensations of warmth, weakness, and fatigue. These may be followed by confusion, slurred speech, and other nonspecific behavioral changes [10]. Severe neuroglycopenia can cause disturbances of integrative function, obtundation, seizures, coma, and, rarely, a permanent vegetative state.
Hypoglycemia can be associated with acute pulmonary edema [11], supraventricular and ventricular tachycardias, atrial fibrillation, and junctional dysrhythmias [12,13,14]. Electrocardiographic abnormalities associated with hypoglycemia include T wave flattening, increased Q-T interval, ST segment depression, and repolarization abnormalities [15,16,17]. Bradycardias have also been attributed to hypoglycemia, but only rarely [18]. Prolonged hypoglycemia can be associated with hypothermia [19,20,21], respiratory failure [22], and hypokalemia and hypophosphatemia [23].
Normal Glucose Regulatory Physiology
Glucose Utilization
Plasma glucose concentration is maintained in a narrow range (∼ 60 to ∼ 120 mg per dL; ∼ 3.3 to ∼ 6.7 mmol). The organ most dependent on glucose is the brain, which consumes ∼ 150 g per day. Unlike fat or muscle, the CNS does not require insulin for glucose transport. During starvation, the brain uses ketone bodies as a substitute fuel (see Chapter 100), but acquisition of the capability to use this alternate substrate requires hours to days. Even during prolonged starvation, the brain still needs ∼ 44 g per day of glucose. Other tissues with obligate glucose needs include erythrocytes (∼ 36 g per day) and renal medulla (∼ 25 g per day).
Sources of Plasma Glucose
There are two sources of blood glucose: endogenous and ingested carbohydrate. In the postprandial state, the concentration of circulating insulin rises in response to the increase in glucose concentration. The insulin promotes (a) transport of glucose into skeletal muscle for immediate use or storage as muscle glycogen and (b) hepatic storage of glucose in the form of liver glycogen. When all other metabolic demands for glucose are being met, some excess glucose is used for synthesis of triglyceride.
During brief starvation, such as when asleep, the principal source of glucose is hepatic glycogen. During prolonged starvation, glucose is derived principally from conversion of muscle-derived amino acids. Smaller contributions to gluconeogenesis are made by (a) glycerol derived from fat and (b) lactate produced by anaerobic glycolysis. When euglycemia is maintained by glycogenolysis or gluconeogenesis, insulin levels are low but never zero.
The liver stores only 60 to 80 g of glycogen, a supply that is exhausted by an overnight fast. Muscle glycogen stores are ∼ 120 g, but this glycogen is not directly available for the maintenance of systemic glucose concentrations due to the lack of glucose-6-phosphatase in muscle. Muscle glycogen contributes to plasma glucose only via anaerobic glycolysis, leading to the production of lactate, which is transported to the liver and converted into glucose. The shuttling of glucose to muscle and lactate to the liver comprises the Cori cycle. Muscle can also amidate pyruvate to form alanine, which is then exported to the liver and converted to glucose. Gluconeogenesis occurs principally in the liver using amino acids, glycerol, and lactate as substrates. The enzymes and pathways required for gluconeogenesis are shown in Figure 106.1.
Hormonal Regulation of Plasma Glucose Concentration
Insulin is the most important regulator of glycemia, promoting glucose uptake and storage in the fed state, and regulating glycogenolysis, gluconeogenesis, and lipolysis in the fasted state. Glucagon, glucocorticoids, catecholamines, growth hormone, and, to a lesser degree, thyroxine promote the formation of glucose in the fasted state and in general counteract the actions of insulin. Foremost among these “counterregulatory” hormones is glucagon, which is secreted in response to low glucose. It promotes both glycogenolysis (an immediate effect) and gluconeogenesis (a delayed but more enduring effect). Glucocorticoids antagonize the action of insulin, stimulating gluconeogenesis and inhibiting extrahepatic glucose utilization. Catecholamines also promote glycogenolysis and gluconeogenesis. The mechanism by which growth hormone promotes blood glucose elevation is not fully understood. The influence of thyroxine on blood glucose concentration is probably indirect. Fasting glucose tends to be elevated and decreased in hyperthyroid and hypothyroid patients, respectively. Absence of liver glycogen has been observed in hyperthyroid animals [24].
Classification of Hypoglycemia
Hypoglycemia can be classified as fasting (or postabsorptive) or postprandial (or “reactive”). The former always represents a major health problem that requires further evaluation. Hypoglycemia that occurs only after eating can be more difficult to assess. It is usually minimally symptomatic and is attributed variously to exaggerated insulin response, renal glycosuria, defects in glucagon responses, and high insulin sensitivity [25,26]. Some cases of postprandial hypoglycemia, however, are severe and associated with health issues including mutations in the insulin receptor [27], autoantibodies against insulin [28], gastric “dumping” after bypass surgery, and nesidioblastosis, all of which are described later. Postprandial hypoglycemia generally does not require intensive care.
Differential Diagnosis of Hypoglycemia
Hypoglycemia that fulfills Whipple’s triad always implies a major disturbance in glucose homeostasis, which is dependent on
three factors: (a) the hormonal milieu, (b) the viability of organs responsible for gluconeogenesis, and (c) availability of substrate for conversion to glucose. The differential diagnosis of medically important hypoglycemia subsumes three corresponding categories: (a) states of hormonal imbalance, principally excess insulin, (b) states of impaired endogenous glucose production, and (c) states in which gluconeogenic substrates are unavailable.
three factors: (a) the hormonal milieu, (b) the viability of organs responsible for gluconeogenesis, and (c) availability of substrate for conversion to glucose. The differential diagnosis of medically important hypoglycemia subsumes three corresponding categories: (a) states of hormonal imbalance, principally excess insulin, (b) states of impaired endogenous glucose production, and (c) states in which gluconeogenic substrates are unavailable.
Hypoglycemia Due to Hormonal Imbalance
Insulin
Insulin Overdoses in Diabetic Patients
Insulin overdose is the most common cause of hypoglycemia. In an outpatient setting, it is frequently the result of a missed meal or an increased amount of exercise and less often the result of inadvertent injection of short acting in place of long-acting insulin. Occasionally, an overdose is intentional, particularly among adolescents. Rarely, overdoses represent attempts at suicide and homicide [29]. The rapidity of onset [30] and duration of hypoglycemia [31] depend on the type of insulin (see Table 106.1).
Hypoglycemia is often more severe among patients with long-standing type 1 diabetes due to the presence of a defective counterregulatory response and hypoglycemia unawareness. Impaired glucagon and epinephrine responses are common [32]. When counterregulation is impaired, adrenergic warning signals like tremor, diaphoresis, and tachycardia may not occur and neuroglycopenic symptoms can develop rapidly. The phenomenon is sometimes described as hypoglycemia-associated autonomic failure [33]. Inadequate counterregulatory responses can also delay recovery from hypoglycemia.
Hypoglycemia in ICU patients can result from rapid changes in clinical status, medications that impair counterregulation (e.g., high-dose β-adrenergic blockers), and the presence of intercurrent disease processes. Although insulin-induced hypoglycemia is easily diagnosed in the ICU, it is crucial to determine exactly why it occurred.
Table 106.1 Insulin Preparations | |||||||||||||||||||||||||||||||||||||||||||||||||||||||||||||||||||||||||||||||||||||
---|---|---|---|---|---|---|---|---|---|---|---|---|---|---|---|---|---|---|---|---|---|---|---|---|---|---|---|---|---|---|---|---|---|---|---|---|---|---|---|---|---|---|---|---|---|---|---|---|---|---|---|---|---|---|---|---|---|---|---|---|---|---|---|---|---|---|---|---|---|---|---|---|---|---|---|---|---|---|---|---|---|---|---|---|---|
|
Intensive insulin management is demanding [34]; occasionally, insulin is administered either in the wrong dose or in response to a factitiously elevated blood glucose measurement or to the wrong patient. Even the most careful attempts to lower blood glucose in the ICU to levels less than 110 mg per dL can frequently be associated with hypoglycemia [35,36,37,38,39]. Failure to adjust insulin infusion rate after decreasing nutritional support is common [2]. Total parenteral nutrition solutions containing insulin can produce hypoglycemia [3], particularly if improvement in a patient’s underlying condition leads to reduced peripheral insulin resistance. Insulin-induced hypoglycemia in the ICU can be related to adrenal insufficiency, renal failure, sepsis or drug toxicity, continuous venovenous hemofiltration with bicarbonate replacement fluid, and need for inotropic support [3].
Intentional Insulin and Oral Hypoglycemic Agent Overdoses in Nondiabetic Patients
“Factitious” hypoglycemia should be suspected in anyone with access to insulin or oral hypoglycemic agents, including healthcare workers and relatives of persons with diabetes. The diagnosis can be difficult. These patients are trying to frustrate rather than facilitate a diagnosis, and they may devise ingenious methods to conceal their actions. Insulin intended for surreptitious injection has been found hidden in electronic devices and body cavities. Surreptitious use of oral hypoglycemic agents, which act by increasing endogenous insulin secretion, can be particularly problematic to diagnose [40,41]. Factitious hypoglycemia can represent malingering, attempted suicide or homicide, Münchausen syndrome, and Münchausen-by-proxy syndrome [29]. It has also been reported to result from adulteration of herbal and counterfeit prescription drugs with oral agents [42,43].
Insulinoma
Insulinomas are insulin-secreting pancreatic islet tumors. They are uncommon but do represent the most common form of pancreatic islet neoplasm. Most (> 90%) are nonmalignant. They are typically small and difficult to visualize radiographically. To evaluate a patient with suspected
insulinoma, fasting immunoreactive insulin (IRI; measured in microunits [μU] per milliliter) and glucose (milligrams per deciliter) should be obtained. Insulinoma is usually associated with an IRI/glucose ratio > 0.3. Elevated levels of C-peptide and proinsulin help confirm the diagnosis by documenting that the source of insulin is endogenous. Proinsulin is typically elevated up to 25% of the insulin concentration in cases of insulinoma [44].
insulinoma, fasting immunoreactive insulin (IRI; measured in microunits [μU] per milliliter) and glucose (milligrams per deciliter) should be obtained. Insulinoma is usually associated with an IRI/glucose ratio > 0.3. Elevated levels of C-peptide and proinsulin help confirm the diagnosis by documenting that the source of insulin is endogenous. Proinsulin is typically elevated up to 25% of the insulin concentration in cases of insulinoma [44].
It can be difficult to differentiate among insulinoma, factitious hypoglycemia due to self-administration of insulin, and abuse of oral hypoglycemic agents. When intentional insulin overdose is suspected, insulin measurements are of limited value. Patients with factitious hypoglycemia may have circulating anti-insulin antibodies that interfere with the radioimmunoassay for insulin. This is true even if human insulin is injected. These patients may appear to have elevated levels of insulin, just as would patients with an insulinoma. In this circumstance, simultaneous glucose, insulin, and C-peptide concentrations are helpful. Insulin and C-peptide are normally cosecreted by the pancreas in equimolar quantities, but the latter is not present in insulin for injection. Absence of C-peptide in a patient with unexplained fasting hypoglycemia strengthens the possibility of surreptitious use of insulin. If oral hypoglycemic agent abuse is suspected, both serum and urine should be screened.
Nonislet Tumors that Secrete Insulin
Nesidioblastosis or Persistent Hyperinsulinemic Hypoglycemia (PHH)
Nesidioblastosis (nonmalignant islet cell hyperplasia) is a rare form of nonmalignant islet cell adenomatosis that leads to insulin-mediated hypoglycemia. In infants, nesidioblastosis is typically characterized by islet hyperplasia, β-cell hypertrophy, and increased β-cell mass. It can be either diffuse or focal and is sometimes termed persistent hyperinsulinemic hypoglycemia of infancy (PHHI) [50,51,52,53]. Rapid diagnosis of the childhood form is crucial to avoid hypoglycemic damage to the maturing CNS. Several different genetic mutations have been associated with the disorder cases [51,52,54,55,56,57]. Special cases of hyperinsulinemic hypoglycemia in infancy include Costello syndrome [58] and the Beckwith–Wiedemann syndrome, which is due to defects in pancreatic β-cell potassium channels [59].
A small number of cases of adult nesidioblastosis have been reported [51,60,61]. The pathological findings are reportedly less consistent than in infants [62], and the cause is unknown. Additional cases of PHH have been diagnosed in adults who had previously undergone Roux-en-Y gastric bypass surgery for weight reduction [63]. Whether these cases represent nesidioblastosis [63,64], “dumping syndrome,” or a reactive process leading to or unmasking a defect in β-cell function [65] is unclear, perhaps in part because the pathological diagnosis of nesidioblastosis is difficult [62]. Treatment options include diazoxide, streptozocin, calcium channel blockade [66], octreotide (discussed later), percutaneous gastrostomy into the remnant stomach [67], as well as partial pancreatectomy [64].
Antibody-Mediated (“Autoimmune”) Hypoglycemia
Autoimmune hypoglycemia can result from autoantibodies directed against insulin itself or autoantibodies directed against the insulin receptor [68]. Both can occur in either insulin-treated [69] or nondiabetic individuals, and both types of autoantibodies can be found in the same patient [70]. About 90% of cases have been reported from Japan [71]. Serum insulin concentrations typically are extremely high, usually higher than those produced by insulinomas. It is assumed that in these cases, glucose administration causes excessive insulin response because the antibodies buffer most of the insulin secreted [72].
Endogenous antibodies that bind to and activate the insulin receptor can also cause hypoglycemia [73]. Some, but not all cases, are associated with other autoimmune disorders [74,75,76], and a few have occurred in patients with myeloma [77]. Previous exposure to exogenous insulin is not necessary, but some patients may have an abnormal insulin molecule [78]. Metformin may be helpful in the management of some cases [79]. Some cases respond to immunosuppressive therapy [73]. The natural history of this syndrome is that the antireceptor antibodies disappear and the syndrome resolves over a time course of months to years [28].
Pancreas and Islet Transplantation
Hypoglycemia can occur after pancreas and islet transplantation [80], but it is generally not a significant clinical problem [81,82]. Islet transplantation has been reported to resolve hypoglycemia unawareness [83]. There is a report of nesidioblastosis-like transformation of a successful pancreas allograft [84].
Nonislet Tumors with Insulin-Like Activity
Certain tumors not of pancreatic islet origin are associated with fasting hypoglycemia clinically indistinguishable from that caused by islet cell neoplasms. Whereas insulinomas are typically quite small, nonpancreatic neoplasms associated with hypoglycemia tend to be large mesenchymal tumors. The large size initially suggested that excessive glucose utilization by the tumor was the cause of the hypoglycemia, but subsequent studies suggest that most cases result from secretion of high-molecular-weight insulin-like growth factor II (pro-IGF-II or “big IGF-II”) [85,86]. Pro-IGF-II levels decrease significantly and hypoglycemia resolves after successful tumor resection [87,88,89,90,91,92]. Abnormal processing of the IGF-II molecule may play a role in some cases [88,90,93]. Nonislet cell neoplasms associated with hypoglycemia include gastrointestinal stromal cell tumors [94], hemangiopericytoma [95,96,97], hepatoma [98,99], uterine tumors [100], renal tumors [101], mesenteric sarcomas [102], colorectal cancer [103], gastric cancer [104], adrenocortical carcinoma, lymphoma, poorly differentiated thyroid cancer [105], somatostatinoma [106], phylloides tumor [107], and leukemia [108]. Multiple myeloma may also cause hypoglycemia via an antibody-mediated mechanism described later [76].
The diagnosis of hypoglycemia due to production of pro-IGF-II by nonislet cell tumors requires the exclusion of insulinoma. As noted earlier, this can be done by obtaining simultaneous insulin and glucose measurements during hypoglycemia. The observation of increased blood glucose concentration after intravenous administration of glucagon, an index of adequate glycogen stores, may also help discriminate between insulin and IGF-secreting tumors [109]. Methods have been developed for measuring serum pro-insulin-like growth factor-II directly [86]. Hypoglycemia associated with high levels of IGF-I has rarely been reported [110].
Hypoglycemia Due to Noninsulin Hypoglycemic Agents
Pharmaceuticals other than insulin that are used to treat type 2 diabetes fall into two classes. “Hypoglycemic agents” enhance insulin secretion and can cause hyperinsulinemic hypoglycemia. The sulfonylureas and meglitinides belong to this class; all are taken orally. “Antidiabetic agents” promote normoglycemia through mechanisms other than enhancement of insulin secretion; they include both oral and injectable agents. When given as monotherapy they do not cause hypoglycemia, but they can amplify the glucose-lowering activity of insulin
and oral hypoglycemic agents. The thiazolidinediones (TZDs), biguanides, α-glucosidase inhibitors, glistens, and bromocriptine mesylate (Cycloset™) are oral antidiabetic agents. Exenatide, liraglutide, and pramlintide are injectable antidiabetic agents. As discussed later, many drugs not used in the treatment of diabetes can also amplify the glucose-lowering activity of oral hypoglycemic agents, and a complete medication history can be critical in the diagnosis of hypoglycemia.
and oral hypoglycemic agents. The thiazolidinediones (TZDs), biguanides, α-glucosidase inhibitors, glistens, and bromocriptine mesylate (Cycloset™) are oral antidiabetic agents. Exenatide, liraglutide, and pramlintide are injectable antidiabetic agents. As discussed later, many drugs not used in the treatment of diabetes can also amplify the glucose-lowering activity of oral hypoglycemic agents, and a complete medication history can be critical in the diagnosis of hypoglycemia.
Table 106.2 Oral Hypoglycemic Agents | ||||||||||||||||||||||||||||||||||||||||||||||||||||||||
---|---|---|---|---|---|---|---|---|---|---|---|---|---|---|---|---|---|---|---|---|---|---|---|---|---|---|---|---|---|---|---|---|---|---|---|---|---|---|---|---|---|---|---|---|---|---|---|---|---|---|---|---|---|---|---|---|
|
Sulfonylureas
After insulin, these are the most common cause of hypoglycemia [111] (Tables 106.2 and 106.3). Sulfonylureas reduce serum glucose by increasing insulin secretion, inhibiting glycogenolysis and gluconeogenesis, and enhancing the response of target tissues to the effects of insulin [112]. Severe hypoglycemia is not common with appropriate administration of these drugs [113], but it can be observed in several contexts [114]. In all age groups, the condition is most often observed in the context of decreased carbohydrate intake. Maternal treatment of diabetes with glyburide can lead to postpartum hypoglycemia in neonates [115]. In patients between the ages of 11 and 30 years, perhaps two-thirds of hypoglycemic comas are due to sulfonylurea agents [41]. Half of these cases are suicide attempts [111]. In patients with type 2 diabetes older than 60 years, sulfonylurea-induced hypoglycemia is a frequent complication [114,116].
Liver disease decreases the clearance of tolbutamide, acetohexamide, tolazamide, glipizide, glyburide, and glimepiride (Table 106.2). Metabolites of sulfonylureas are excreted in urine with one exception; 50% of glyburide metabolites are excreted in bile. Accordingly, sulfonylurea-induced hypoglycemia is often observed in older individuals in the setting of acute or chronic starvation superimposed on mild to moderate liver or renal failure.
The half-life of some sulfonylureas is > 24 hours and the duration of action is often even longer (Table 106.1). Hepatic and renal insufficiency can extend their half-lives. Patients with sulfonylurea-induced hypoglycemia should therefore be hospitalized after initial resuscitation with glucose. They require continued treatment with oral and intravenous glucose for a minimum of 18 to 24 hours.
When oral agent overdosage is suspected, serum and urine should be screened for sulfonylurea compounds. Sulfonylurea drugs circulate bound to proteins, and drugs of several classes can displace sulfonylureas and enhance their hypoglycemic effect (Table 106.3). The constellation of insulin concentration ≥ 3.9 μU per mL, C-peptide ≥ 1.4 ng per mL, and glucose < 49 mg per dL may be helpful in diagnosing sulfonylurea-induced hypoglycemia [40].

Full access? Get Clinical Tree
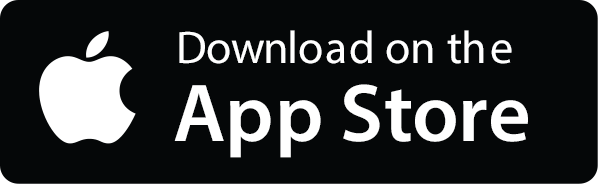
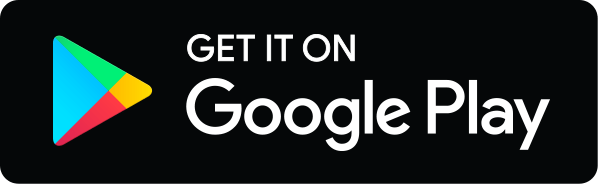
