The morbidity and mortality resulting from traumatic brain injury (TBI) stem not only from the primary brain damage caused by the initial impact but also from the secondary insults that follow. Although the primary injury occurs nearly immediately and is largely irreversible, secondary insults include a variety of ischemic, metabolic, and inflammatory disturbances that occur in the vulnerable brain tissue. These insults develop over hours to days after injury. Given the delayed presentation, these secondary insults represent an opportunity for clinical intervention. Recognition and treatment of these evolving processes are critical to ensuring optimal outcome following serious brain injury.
In the following discussion, we outline the evidence supporting various intensive care unit (ICU) practices in the management of the severely brain injured to prevent secondary brain damage. We follow and summarize the general format of the Brain Trauma Foundation (BTF) management guidelines. The specific studies that are included were evaluated with GRADE (Grading of Recommendations Assessment, Development and Evaluation) criteria, as detailed in Table 62-1 .
Underlying method
|
Factors that may decrease the strength of evidence
|
Main factors that may increase the strength of evidence
|
Systemic Blood Pressure and Oxygenation
Background
Both hypotension and hypoxemia contribute to secondary brain injury and influence outcomes after trauma. Because of ethical constraints, it is not possible to perform randomized studies to establish absolute thresholds for oxygenation and blood pressure support. In the following section, we discuss the available evidence that can provide some guidance to these thresholds.
Evidence
Hypoxemia
Analysis of the prospectively collected Traumatic Coma Data Bank showed that episodes of hypoxemia (partial pressure of oxygen in arterial blood [Pa o 2 ] <60 mm Hg or apnea/cyanosis in the field) in the acute period after injury were associated with increased mortality. Studying prehospital trauma patients, Stocchetti et al. found that hypoxemia (defined as a peripheral oxygen saturation <60%) at the accident scene was associated with a universally poor prognosis (death or severe disability), as was severe hypotension (defined as systolic blood pressure <60 mm Hg). In 3240 patients included in the San Diego County trauma registry, both hypoxemia (Pa o 2 <110 mm Hg) and extreme hyperoxemia were associated with increased mortality and poorer clinical outcomes in survivors of TBI. Hypoxemia in head-injured patients cared for in the ICU also increases mortality.
Hypotension
Similar results have been reported with prehospital and in-hospital hypotension. A single episode of hypotension with a systolic blood pressure lower than 90 mm Hg was associated with increased morbidity and doubled mortality. Similarly, Marmarou and colleagues used the Traumatic Coma Data Bank to study 428 patients with severe TBI and found that as the proportion of systolic blood pressure measurements <80 mm Hg or intracranial pressure (ICP) >20 mm Hg increased, patient outcome worsened. A series of prospective studies by Vassar and colleagues evaluating resuscitative fluids given before hospital admission in hypotensive trauma patients demonstrated that hyperosmolar fluid resuscitation (7.5% hypertonic saline [HTS] ± dextran) more effectively raised blood pressure than isotonic solutions, and patients who received hyperosmolar resuscitation had better outcomes than predicted. In the subset of trauma patients with a Glasgow Coma Scale (GCS) score of 8 or less, the hyperosmolar treatment group fared significantly better than the isotonic group. A follow-up prospective study looking specifically at prehospital resuscitation of hypotensive patients with severe TBI showed no difference in neurologic outcome at 6 months in patients resuscitated with hypertonic fluids versus conventional fluid resuscitation.
Recommendations
Hypoxemia and hypotension are associated with poor outcomes from TBI and should be avoided. GRADE B evidence supports a threshold value of 90 mm Hg systolic and Pa o 2 mm Hg less than 60 with O 2 saturation less than 90%, respectively.
Cerebral Perfusion Thresholds
Background
On the basis of Pouiselle’s law describing laminar flow, cerebral blood flow (CBF) is directly related to the cerebral perfusion pressure (CPP), which is defined as mean arterial pressure (MAP) – ICP and is inversely related to the cerebral vascular resistance (CVR) (CBF = CPP/CVR). Under normal conditions, CBF remains constant despite changes in CPP by constriction and dilation of the cerebral blood vessels, termed cerebral autoregulation. After brain injury, cerebral autoregulation is often disrupted such that blood vessel radius either remains constant, despite changes in MAP and CPP, or varies directly (inversely) with CPP. In both cases, even small changes in CPP can trigger alterations in CBF, blood flow velocity, and ICP, which, in turn, compromises oxygen and glucose delivery to the brain tissue. Given the lack of tools to directly and continuously measure CBF, CPP has been used as a monitoring parameter reflective of cerebral perfusion.
Evidence
Low CPP (generally defined as less than 50 mm Hg), typically caused by systemic hypotension (low MAP) or intracranial hypertension (high ICP), is associated with poor clinical outcome as well as poor physiologic variables, including jugular venous oxygen saturation, brain oxygen saturation, and cerebral microdialysis parameters.
Rosner and Daughton originally reported management on head-injured patients based on CPP thresholds. They studied 34 patients and used a variety of interventions to achieve CPP greater than 70 mm Hg and concluded that this was associated with a decreased mortality and an improvement in functional outcome over prior cohorts, although it was unclear whether this was just due to a lower incidence of systemic hypotension. Follow-up studies, however, suggested that there were risks of CPP augmentation. Robertson et al. performed a randomized controlled trial (RCT) of CPP-guided versus ICP-guided management and found a fivefold increase in the development of acute respiratory distress syndrome (ARDS) in patients managed by CPP, presumably a consequence of overenthusiastic fluid resuscitation. Contant et al. found a similar incidence of ARDS in their CPP-managed TBI patients. This complication was associated with an increased risk of mortality and poor neurologic outcome.
TBI is, by its very nature, a heterogeneous disease process with varying cerebral physiologies not only between patients but also between brain regions in a single patient. Thus, it is likely overly simplistic to believe that every individual (or, perhaps, every region of the brain) requires the same CPP to maintain adequate perfusion and avoid hyperperfusion or ICP changes. To this end, methods to measure individual cerebral autoregulation have been developed. Steiner et al. retrospectively calculated a correlation coefficient defining the relationship between CPP and ICP to determine the CPP at which autoregulation was best preserved. When the mean CPP was maintained near the level of autoregulation, there was an association with better outcomes; patients whose CPPs were either higher or lower than the optimal CPP faired more poorly. These data suggest that CPP can be optimized on an individual basis with adjunctive physiologic monitoring. However, the efficacy of this approach remains to be tested in large, prospective RCTs.
Another consideration in optimizing CPP is how the CPP is measured. Lassen originally defined CPP based on “arterial blood pressure measured at the level of the head,” using the tragus of the ear as an external landmark. However, there is much heterogeneity in current practice, and the MAP is often measured at the level of the right atrium. When the head of the bed is elevated, as it should be for the management of head-injured patients, MAP measurements at the level of the right atrium may be higher than those measured at the tragus by up to 18 mm Hg, thereby overestimating CPP. This variability in practice may contribute to difficulty in defining optimal CPP and MAP targets after head injury. In this regard, the Neuroanesthesia Society of Great Britain and Ireland and the Society of British Neurologic Surgeons recently issued a joint position statement advocating for measurement of CPP at the level of the tragus in patients with TBI ( http://www.nasgbi.org.uk/media/uploads/NASGBI_and_SBNS_CPP_statement_final.pdf ).
Recommendation
We recommend measuring CPP at the level of the tragus. Low CPP, generally defined as less than 50 mm Hg, should be prevented (GRADE B). Augmentation of CPP with fluids should be avoided because of the risk of iatrogenic complications based on GRADE B evidence. Adjunctive physiologic monitors to determine the CPP at which autoregulation is optimized may be helpful to individualize care, but there is currently no evidence to support a recommendation regarding their use.
Intracranial Pressure Thresholds
Background
The bony, rigid skull and vertebral canal contain a fixed volume including the brain parenchyma, cerebrospinal fluid (CSF), and blood, creating a nearly incompressible system. Any increase in the volume of one of these compartments is met first with a compensatory shift in another compartment (e.g., CSF shifting down the less rigid spinal column). When compensatory changes are exhausted, however, the ICP increases dramatically and can cause secondary brain injury due to brain compression, herniation, and cerebral perfusion compromise. However, treatment of ICP must be balanced with the potential side effects of medical and pharmacologic ICP management.
Evidence
There are no prospective randomized studies designed to determine the threshold for initiation of ICP-reducing therapy. Several prospective observational studies reviewed suggest that outcomes improve when ICP is maintained at less than 20 to 25 mm Hg and that brain herniation is more likely above these thresholds. However, in situations without intracranial mass lesions, ICP greater than 20 mm Hg may be tolerated.
Chesnut et al. performed the first study to compare outcomes in brain-injured patients who had their management guided by ICP monitoring with those who did not. In this study, the focus was on monitoring ICP, not its treatment. Thus, both arms underwent ICP-reducing therapy. In this population, there was no difference in ICU length of stay or 14-day or 6-month mortality in those who had aggressive management to maintain ICP less than 20 mm Hg compared with patients who were managed on the basis of clinical examination and radiographic imaging alone. The generalizability of these findings has been questioned, but this study raises the important point that ICP monitoring in and of itself may not influence clinical outcomes.
Recommendations
Despite an absence of high-quality evidence, in most cases, an ICP higher than 20 to 25 mm Hg should be an indication to increase ICP-reducing therapy (GRADE C). This must be tempered with the iatrogenic risks of ICP-lowering therapy. When ICP monitoring is unavailable, management based on clinical examination and imaging is reasonable based on the available evidence.
Seizure Prophylaxis
Background
Posttraumatic seizures (PTSs) are classified as early (<7 days after TBI) or late (>7 days after TBI). The incidence of PTS varies from 4% to 25% early and 9% to 42% late in untreated TBI patients. Early PTS may contribute to secondary brain injury by inducing cerebral hypermetabolism, elevated ICP, and hypertension. It has been proposed that early PTSs beget late PTSs and epilepsy through neuronal kindling. Epilepsy can have a significant impact on quality of life in TBI survivors.
Evidence
Temkin et al. performed a randomized, placebo-controlled trial in 404 patients with severe head trauma who received phenytoin or placebo for 1 year after head injury. They found that patients receiving phenytoin had a decrease in the incidence of seizures in the acute period (<7 days) but no difference in late seizures. The same group found that both valproic acid and phenytoin also decreased seizures in the acute period. The BTF meta-analysis concluded that anticonvulsant prophylaxis with phenytoin, carbamazapine, or valproic acid is indicated in the first 7 days after TBI but longer treatment is not warranted. The Cochrane meta-analysis of 11 RCTs and the American Academy of Neurology meta-analysis of 8 RCTs arrived at similar conclusions.
Given the potential toxicities and need for drug level monitoring with both phenytoin and valproic acid, neurointensivists have begun to use levetiracetam for seizure prophylaxis after TBI. In a retrospective review, Caballero et al. found that rates of seizure activity were not different between patients treated with levetiracetam and those treated with phenytoin. Levetiracetam therapy was slightly cheaper. Larger, prospective studies are needed to determine if levetiracetam is efficacious and cost-effective in preventing acute seizures after TBI.
Recommendations
Seizure prophylaxis is indicated in the first 7 days after TBI for seizure prophylaxis (GRADE A). Phenytoin, valproic acid, and carbamazapine are all reasonable antiseizure medications for prophylaxis. In the absence of PTS, treatment should be stopped after 7 days. Levetiracetam may be a reasonable option for seizure prophylaxis in patients in whom phenytoin is contraindicated, but further studies are needed. There is no evidence to support antiepileptic administration for longer than 7 days after TBI in the absence of clinical or electrophysiologic seizure activity.
Management of Elevated Intracranial Pressure: Hyperventilation
Background
During hyperventilation, carbon dioxide concentrations fall, resulting in constriction of cerebral blood vessels. Vasoconstriction decreases CBF to reduce intravascular volume in the cranial cavity and lower ICP. In this regard, voluntary hyperventilation may be one of the first clinical signs of elevated ICP as consequent tissue acidosis from high ICP produces compensatory hyperventilation. Similarly, hyperventilation and maintenance of a modestly lower partial pressure of carbon dioxide in arterial blood (Pa co 2 ) (30 to 35 mm Hg) are the first measures that can be used to lower ICP in the mechanically ventilated patient. Given that hypocarbia triggers intracranial vasoconstriction, the risk of decreased CBF and resultant cerebral ischemia as a consequence of hyperventilation must be considered, although hyperventilation has not been reported to cause brain injury in the absence of TBI.
Evidence
Several studies of CBF suggest that CBF is dangerously low in the acute period after TBI, leading the BTF to advise against aggressive hyperventilation (Pa co 2 <25 mm Hg) after TBI. Muizelaar et al. performed a prospective, randomized study of hyperventilation in TBI and found a worse functional outcome, though no difference in mortality, at 3 and 6 months, but not at 12 months, in the hyperventilated patients.
Recommendations
Prolonged prophylactic hyperventilation to Pa co 2 of 25 to 30 mm Hg should be avoided in TBI patients (GRADE B) because of risks of cerebral ischemia. It may be justified if accompanied by a monitoring of CBF adequacy. It also may be justified as a temporary intervention in emergency and temporary sudden increases in ICP such as with life-threatening herniation syndromes. Hypercarbia (Pa co 2 > 45) should be avoided as this can trigger hyperemia, decreased CPP, and potentially trigger a sudden ICP elevation. There is insufficient evidence to determine the effect of hyperventilation on patient outcome after TBI.
Management of Elevated Intracranial Pressure: Hyperosmolar Therapy
Background
Osmotic agents are thought to lower ICP primarily by drawing free water out of the brain parenchyma and into the systemic vasculature. The simplest way to maintain a modest osmotic gradient is to maintain the serum sodium on the upper range of normal (∼145) in the euvolemic patient. When a stronger gradient is needed, mannitol and HTS are the two most commonly used agents.
Evidence
Although RCTs are lacking, mannitol and HTS are both effective agents for lowering ICP after TBI. Mannitol was superior to barbiturates in ICP control, maintenance of CPP, and mortality when given as a 20% bolus at 1 g/kg and repeated to maintain ICP greater than 20.
Early studies focused on the use of HTS for resuscitation in trauma patients. Shackford and colleagues were the first to specifically look at HTS infusion and ICP, finding that HTS significantly lowered ICP. Several studies report efficacy of HTS in decreasing ICP, notably being effective in cases where ICP is refractory to mannitol therapy. A trial evaluating equiosmolar doses of mannitol and 3% HTS in the operating room revealed an equivalent effect on “brain relaxation,” suggesting equivalent ICP reduction with equiosmolar therapy.
Another prospective, randomized study of 20 TBI patients looked at the effect of equivalent volumes of 7.5% HTS and 20% mannitol (2400 mOsm/kg HTS, 1160 mOsm/kg mannitol), showing a lower incidence of treatment failure with HTS. A recent study using brain tissue oxygen monitoring in TBI found that, compared with 0.75 g/kg 20% mannitol, 7.5% HTS boluses were associated with lower ICP and higher CPP and cardiac output. They concluded that in patients with severe TBI and elevated ICP refractory to previous mannitol treatment, 7.5% HTS administered as second-tier therapy is associated with a significant increase of brain oxygenation and improved cerebral and systemic hemodynamics.
Recommendations
Both mannitol and HTS are effective in the treatment of elevated ICP based on GRADE B evidence. Although small studies suggest that HTS may result in better ICP control in patients who no longer respond to mannitol therapy, no RCTs have directly compared the two treatments. As such, it is not possible to recommend one agent over the other agent. Other factors, such as volume status or kidney function, may favor use of one agent over another but should be assessed on a case-by-case basis.
Management of Elevated Intracranial Pressure: Surgical Decompressive Therapy
Background
When severe cerebral edema causes elevated ICP, decompressive craniectomy, a surgical procedure in which a portion of the skull is removed and the dura is opened, can provide definitive management. With the rigid cranium opened, brain swelling can occur outside of the cranial cavity without compromising surrounding structures. The surgical approach varies depending on the site and extent of injury. Hemispheric, bifrontal, or bihemispheric bone flaps may be removed. A durotomy is also performed to provide additional space into which the swelling brain can expand. The use of decompressive craniectomy to control elevated ICP has a long history dating to the early twentieth century. Only recently has the procedure started to undergo scrutiny for impact on outcome.
Evidence
In 26 patients who underwent bifrontal craniectomy for the treatment of refractory elevated ICP after severe TBI, surgical intervention was associated with significant reductions in ICP, and outcomes were favorable in 69% of patients, a significant improvement over similarly described cohorts. In this regard, Aarabi et al. found that decompressive craniectomy significantly lowered ICP in 85% of patients and was associated with a favorable outcome in 51% of patients at 3 months.
On the basis of these initial observations, 155 patients with severe, diffuse TBI who had elevations in ICP refractory to standard first-line therapies were enrolled in the first RCT of craniectomy after TBI. Subjects were randomized to undergo bifrontotemperoparietal craniectomy or standard medical therapy. Although decompressive craniectomy did control ICP and result in fewer ICU days, surgical intervention was associated with more unfavorable outcomes (death, vegetative state, or severe disability) than standard medical therapy. There remain questions, though, regarding the generalizability of these data, particularly given that a large proportion (27%) of patients in the surgical arm had bilateral unreactive pupils at randomization (as compared with 12% in the medical management arm), a clinical finding historically associated with very poor outcomes.
Recommendations
Surgical decompressive therapy is an effective measure for the management of persistently elevated ICP. However, there is no evidence that decompression improves clinical outcomes. We recommend that surgical decompressive therapy be considered as a lifesaving measure in patients in whom other measures to control ICP have failed and who may have a chance of functional outcome. It is important that families consenting for this procedure be counseled that the surgical procedure may be lifesaving but does not guarantee a satisfactory functional outcome.

Full access? Get Clinical Tree
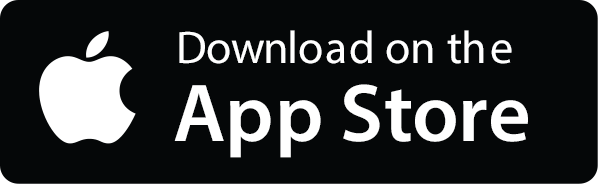
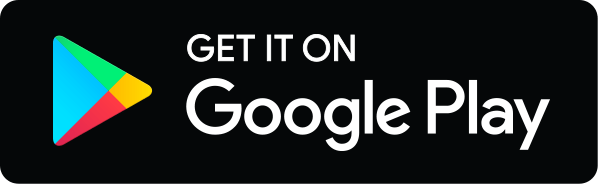