Chapter 84 Hemoglobinopathies
Appendix materials for this chapter are available online at http://www.expertconsult.com.
Perspective
Hemoglobinopathies are plentiful as many provide a protective effect to malaria. Although 5% of humans have hemoglobin variants, an estimated 300,000 affected homozygotes are born annually.1 Contrary to popular beliefs, hemoglobinopathies do not provide resistance to infection, but rather diminish the chance of death once infected, allowing the development of partial immunity. Although sickle cell and thalassemia trait increase fitness, homozygotes in malaria endemic areas often do not survive into their teens. Although this strong protective effect resulted in a high incidence of hemoglobinopathies in the equatorial malaria zones, the slave trade and more recent voluntary migrations has led to the worldwide distribution of these conditions.
The Globin Gene Loci
The α- and β-like genes reside within multigene loci and are transcribed at unparalleled levels in both tightly tissue specific, and developmentally specific patterns.2 This, and their involvement in human disease, has made these loci paradigms for gene regulation and pathophysiology. The β-globin gene was the first sequenced, and sickle cell was the first molecularly defined and diagnosed disease. Most forms of mutation (e.g., point, splicing, deletion, gene rearrangement) are observed in thalassemia, making these the quintessential teaching loci. This high variety and incidence of mutations result in frequent compound heterozygotes with a wide spectrum of clinical manifestations.
The five genes of the β-globin locus reside in a cluster on chromosome 11. The genes are expressed in an erythroid, and developmentally stage-specific manor; the ε, Aγ and Gγ, and δ and β genes being expressed primarily during the embryonic, fetal, and postnatal periods, respectively. At birth 95% of β-like chains are γ, with the rest being β. This ratio gradually inverts during the first year of life, explaining why phenotypes limited to the β-globin gene such as sickle cell and most β-thalassemias do not manifest until several months of age. Expression of the chromosome 16 based α-like genes differs; the embryonic ζ-gene parallels the expression of ε, but the twin α-genes are expressed from the fetal period onward. Thus α abnormalities manifest in utero, potentially with devastating consequences (e.g., hydrops fetalis). The resultant hemoglobin α-, β-heterotetramers (HbS) are developmentally expressed; embryonic: Hb Gower1 (ζ2,ε2), Hb Gower2 (α2,ε2), and Hb Portland (ζ2,γ2); fetal: HbF (fetal) (α2,γ2) and adult: HbA2 (α2,δ2) and HbA (Adult) (α2, β2) (Appendix Figure 84-A). Although many suggest HbF, with its higher oxygen affinity, evolved to aid the fetus in “stealing” oxygen from the mother, this is not supported experimentally, the advantage of HbF may in fact lie in differential transport of NO or carbon dioxide. Unfortunately, despite the intensive study, multiple Nobel prizes and the clinical importance of the globin loci, little of the molecular understanding of the loci has been translated to clinical care. The holy grail of manipulating expression from the β-locus remains elusive. Inhibiting adult sickle or thalassemic genes, while concomitantly activating expression from the normal fetal genes would alleviate most disease, obviating the need for this chapter.
Sickle Cell Disease
Molecular Description and Epidemiology
Sickle cell disease (SCD) is a group of single gene, autosomal recessive disorders common in people originating from specific regions of Africa or India and in those of Hispanic descent. SCD encompasses a group of disorders characterized by the presence of a single nucleotide change in the adult β-globin gene, and a second abnormal allele allowing the sickle hemoglobin to polymerize. Substitution of an adenine (A) for a thymidine (T) in codon 6 results in replacement of a hydrophilic glutamic acid residue with a hydrophobic valine residue. The allosteric changes in hemoglobin structure occurring with deoxygenation leads to exposure of a destabilizing valine-containing pocket. The high concentration of hemoglobin in erythrocytes (MCHC) allows for the alignment of these pockets, leading to polymerization and transition to the classic sickle cell morphology (Figure 84-1) and the initiation of downstream events leading to pain and chronic end-organ damage. The presence of HbA in sickle trait, or HbF in the newborn, attenuate polymerization, diminishing the clinical consequences of HbS. In the United States, approximately 1 in 14 and 1 in 400 African Americans have sickle trait and SCD, respectively, with approximately 100,000 cases and approximately 1000 new births per year (in contrast to approximately 1 million births per year in Africa). With increased ethnic mixing, compound heterozygous forms of SCD are increasingly being observed (e.g., S/β-thalassemia or HbS/E). Sadly, though simple public health measures have dramatically decreased mortality rates in the United States, the countries with the highest incidence of SCD often lack such resources and children with SCD often do not reach their teens.
Sickle Cell Trait
Once the presence of HbS has been confirmed, the differential diagnosis consists of severe forms, less severe forms, and carrier states. Sickle cell trait (SCT) is distinguished from SCD by the presence of a normal β-globin allele and more HbA than HbS (typically 40% HbS) (Table 84-1). SCT does not result in the classic spectrum of sickle-related complications or alter red cell indices, but can lead to clinical abnormalities. Impaired renal concentrating abilities and intermittent microhematuria is common. Although of unclear clinical significance, this damage may interact with other pathophysiology in the African-American population (e.g., diabetes), explaining the high risk of chronic renal disease. In addition, there may be an increased risk of venous thromboembolism.3 Most controversial is the concern that extremes of physical exertion, dehydration, and/or altitude may induce sickle cell vaso-occlusive events in some individuals with sickle cell trait.4 This is particularly relevant to student athletes for whom no formal activity restrictions are recommended, but empirically, aggressive hydration during extremes of physical exertion is generally recommended.
Spectrum of Sickle Cell Disease Genotypes
Although homozygous sickle cell (Hb S/S, often called sickle cell anemia) is most common, it is essential to be aware of additional genotypes that result in a similar spectrum of disease (see Table 84-1). That said, once in the intensive care unit (ICU), the form of SCD plays little role in decision-making. In HbS/β+-thalassemia and HbSβ0-thalassemia, the lower the expression of β-like chains from the thalassemic allele, the lower the hemoglobin concentration (Hgb) and MCV, and the higher the percentage of HbS and polymerization, resulting in a more severe clinical phenotype. In contrast, when HbS is expressed in conjunction with HbC, HbD, or Hb O-Arab or several other variants, near normal amounts of hemoglobin are made, but these forms are less able to attenuate HbS polymerization than HbA and result in milder sickle syndromes.
Natural History
The clinical manifestations of SCD result from intermittent episodes of vascular occlusion leading to tissue ischemia/reperfusion injury and variable degrees of hemolysis, both of which contribute to multiorgan dysfunction. The severity of disease manifestations varies widely in individuals with the same genotype and is affected by (1) known genetic modifiers, e.g., α-thalassemia that lowers the MCHC; (2) genetic modifiers of additional pathways contributing to the pathophysiology of SCD (below); (3) behavioral and environmental factors, (e.g., family and community support, racial disparities and access to health care); and (4) psychological factors, (e.g., coping with chronic disease, recurrent pain, and a high rate of depression). The spectrum of manifestations changes with age, and is beautifully described in Gill et al.5 Previously, median survival in the United States for those with SCD was 42 years for men and age 48 years for women6; however, considerable improvements in longevity have been suggested.7,8 The main causes of death are infection, acute chest syndrome, pulmonary artery hypertension, and cerebrovascular events.9,10 What is not fully appreciated by pediatricians is that this increased lifespan is often associated with significant morbidity related to chronic organ damage.
Pathophysiology
Although unable to fully provide the elegant details of the rapidly evolving insights into the multifaceted pathophysiology of SCD, this chapter outlines key pathways with direct relevance to management basics. While the initial era of understanding pathophysiology focused on HbS polymerization and red cell “sickling,” this was followed by an awareness of the critical role of endothelial interactions and inflammation and later, of hemolysis and perturbations of NO metabolism (Figure 84-2).11–14
Red Cells, Inflammation, and the Endothelium
Crystallization leads to deformation of erythrocytes and oxidative damage to cell membrane proteins, leading to the activation of pathways contributing to the stimulation of white cells and inflammation, endothelial damage and the initiation of the clotting cascade and platelet aggregation. Membrane damage triggers movement of phosphatidylserine to the outer leaf of the red cell membrane where it acts as a substrate for factor V and VIII binding, promoting the clotting cascade. Reticulocytes and sickled cells expose increased adherence proteins, slowing flow and increasing CTT and enhancing binding to other cell types. Binding to platelets leads to platelet activation and aggregation, whereas binding to neutrophils stimulates the oxidative burst, damaging endothelium and exposing tissue factor, further promoting coagulation, platelet aggregation, and clot formation. In addition, bound white cells release cytokines, increasing inflammatory cell recruitment and division, perpetuating the process. Thus, sickle cell represents an activated, inflammatory state in which the CTT is easily compromised. This increases the likelihood of additional cells sickling and blood flow becoming further compromised, resulting in ischemic damage, inflammation, and propagation of the cycle. This activation and integration of multiple pathways explains the tremendous clinical heterogeneity observed in people with the same hemoglobin genotype, as well as why patients can show rapid clinical decline. The clinical importance of these paths in SCD is re-enforced by an elevated white blood cell (WBC) count being a risk factor for pain episodes, acute chest syndrome, and early death.6,15 This also explains why infection and trauma are frequent triggers for sickle related pain, and why granulocyte-colony stimulating factor (G-CSF) has led to death in SCD.16 Therapeutically, this points to the importance of nonsteroidal anti-inflammatory drugs (NSAIDs) in affecting the underlying pathophysiology as well as providing analgesia, and why clinical response to hydroxyurea is best correlated with a decrease in WBC.17,18 Finally the identification of these interacting pathways identifies potential targets for new therapies affecting inflammation and cell-endothelial interactions.
Hemolysis and Nitric Oxide Homeostasis
In contrast to the vaso-occlusive complications that correlate with a high WBC and diminished HbF, recently a subset of complications including pulmonary hypertension, skin ulcers, and priapism were found to correlate with increased lactate dehydrogenase and bilirubin and reticulocyte count.12–14 This defines a hemolytic phenotype arising from disturbances in NO hemostasis, and as described at the end of this section, this may be responsible for the inverse association of hemolysis and pain. Hemoglobin accelerates destruction of NO several thousand-fold. Normally laminar flow positions red cells centrally in vessels sequestering hemoglobin away from the endothelium where NO is synthesized and utilized. Hemolysis releases hemoglobin (Fe++) that is now free to diffuse and reduce NO to nitrate with resultant formation of methemoglobin (Fe+++). In addition, hemolysis releases erythrocyte arginase, resulting in arginine depletion, the precursor for NO synthesis. Thus chronic hemolysis leads to decreased NO synthesis and increased destruction, resulting in altered NO hemostasis and increased tone of small vessels, slowing flow and increasing sickling. Optimal management of hemolysis related pathophysiology remains uncertain. Hemolysis prevention with hydroxyurea is appropriate and well accepted, and agents to prevent cellular dehydration (e.g., Mg++ or ICA-17043) are being investigated, but neither is appropriate for acute management. NO metabolism is directly modified with class 5 phosphodiesterase inhibitors (e.g., sildenafil) or by administering NO or related metabolites, and both approaches are being actively investigated for both acute and chronic use. Endogenous sulfur compounds may be tightly integrated with NO pathways, thus a therapeutic role of sulfur compounds may emerge.
In an exciting new paradigm for understanding SCD pain suggested by Gladwin and colleagues,19 NO has been found to affect the processing of nociceptive signals, that may explain why patients with more severe hemolytic disease have fewer vaso-occlusive events (VOE). NO and resultant cGMP signaling increase the hyperexcitability of nociceptive neurons.20 Thus as hemolysis depletes NO, it potentially attenuates pain signaling. Conversely, this may explain why SCD patients with fewer markers of hemolysis have more VOE. The implications of this biochemical pathway on pain management in SCD and other states of high hemolysis such as cardiac bypass are not known (see “Pain” below).
Clinical Manifestations
Although a summary of clinical problems and management are listed in the following section, the reader is referred to the National Heart Lung and Blood Institute standard of care guidelines21 for more details: www.nhlbi.nih.gov/health/prof/blood/sickle/sc_mngt.pdf.
Pain
See Appendix Table 84-A for a detailed care plan, and Appendix Figures 84-B, C, and D for overviews of pain management.22 Vaso-occlusive events, defined as the acute onset of severe pain secondary to ischemic tissue injury, are a hallmark of SCD. The complexities of SCD pain are best summarized by Shapiro and Ballas: “Vaso-occlusion is a physiological process, but the resultant pain is a biopsychosocial phenomenon. Psychosocial issues such as coping skills, social context, personality, mood, and interactions with the health care system mingle with the biologic factors and contribute to the expression of the illness.”23 SCD pain can be acute, recurrent and/or chronic, and is complicated by co-existing chronic disease as well as social and racial overlays. The need for aggressive and rapid treatment is critical and well documented in guidelines of the American Pain Society and British Society of Hematology and should be consulted.24,25 Although aggressive intervention is essential for humane care and physiologic improvements (e.g., improving respiratory mechanics when having rib infarct pain), too often racial attitudes and concerns of drug seeking prevent sufficient delivery to patients in excruciating pain.26 Pain is the most common reason for hospitalization in SCD. The impact of pain is extremely heterogenous in SCD, likely because of differences in genetic background affecting the pathways described above, environment and psychosocial and behavioral variables. In one cooperative study, 80% of patients sought care once or less per year, whereas 5% of patients accounted for one third of hospitalizations.27 Recent daily pain diary assessments suggest these prior studies underestimate the routine pain many experience.28 Risk factors for pain include a high Hgb, high WBC, coexisting alpha thalassemia, and increased levels of cell free DNA, whereas HbF is protective.27,29,30
Pathophysiology, Diagnosis, and Presentation
Pain can start spontaneously but common precipitating factors relate to the pathophysiology and include weather changes (especially cold), dehydration, inflammation, and infections, all of which increase CTT. Occlusion results in ischemic/reperfusion injury and the release of multiple inflammatory mediators that activate nociceptors, evoking a pain response. Pain may involve any part of the body though most commonly affecting the back and long bones. The unpredictability of the pain contributes to the psychological component of pain, potentially interfering with coping mechanisms.31 Recurrent and chronic pain contribute to a decreased quality of life, decreased function, depression and suicidal ideations.28,32,33 Diagnosis is based on a qualitative description, typically biting or gnawing, throbbing, and fatiguing; and quantitative description, that must be trusted (Figure 84-E). The description is essential to help rule out other etiologies of pain, because no physical exam, laboratory, or radiographic studies can confirm or negate this subjective complaint. Physical findings can include swelling, warmth, erythema and tenderness, but exams can be normal and critically, it must be re-enforced that patients can appear without distress. The role of laboratory and radiologic studies is to rule out other etiologies of pain; particularly important for sickle mesenteric pain that must be distinguished from other causes of abdominal pain (e.g., ultrasound to rule out appendicitis or cholecystitis, or tapping a joint to rule out infectious bone or joint processes). Serial quantitative assessments with a developmentally stage specific pain scale is standard of care, though fraught with problems.
Management
See Appendix Table 84-A for a detailed care plan, and Appendix Figures 84-B to 84-D for overviews of pain management.22 An effective management strategy addresses the underlying tissue damage, nociception, history of pain episodes, and doses of medications required to achieve acceptable analgesia, baseline pain medications, history of tolerance, and how people process pain and coexisting depression. Although many are tempted to begin with analgesic medications, there are several other key components to management, such as the following: (1) environmental manipulation. A dark, calm, quiet environment with supportive interactions supports pain reduction. (2) Complementary methods. Many benefit from distraction, prayer, biofeedback, self-hypnosis and similar modalities. (3) Addressing pathophysiology and triggers. Fluids to maintain euvolemia, warmth and NSAIDs decrease CTT and promote conditions less likely to result in vaso-occlusion. (4) Adjuncts. Physical therapy, ambulation, and incentive spirometry should be encouraged to maintain blood flow and prevent atelectasis and acute chest syndrome (ACS). Additional adjuvants such as massage and acupuncture have been tried. After these have been addressed, providers may focus on analgesic medications.
Patients with SCD are the experts in their own pain management. Ideally patients and staff have agreed on individualized, predefined guidelines for pain management taking into account the patient’s degree of opiate tolerance, and side effect profile, and these are documented online or on a patient identification card. Early initiation of NSAIDs is essential, although the choice of NSAID is controversial (e.g., ibuprofen is a better anti-inflammatory, whereas ketoralac is a better analgesic). Notably, half of children will have resolution of pain with intravenous ketorolac.34 For severe episodes, rapid and repeated doses of opiates (dosed every 20 minutes), followed by transition to patient controlled analgesia (if developmentally appropriate) is the accepted standard.35 Although no consensus on the opiate of choice exists, many controversies remain. There are conflicting reports regarding an increased incidence of acute chest syndrome with morphine, with some suggesting decreased rates when nalbuphine is used.36,37 Many avoid meperidine due to concerns of increased seizures and neuroexcitation.38 The goal of opiate use is to balance providing sufficient pain relief to allow one to rest and perform incentive spirometry, while avoiding oversedation, which contributes to the development of pulmonary processes. Certain subsets of patients may benefit from regional anesthesia including epidural,39 dexmedetomidine,40 clonidine, gabapentin, ketamine, and intranasal diamorphine41; although few data exist evaluating their efficacy. One additional promising agent is low molecular weight heparin, which targets the pro-thrombotic and inflammatory state in SCD, Notably a recent trial reported fewer days of severe pain and length of stay with this intervention.42
As mentioned in the discussion of the pathophysiology of SCD, hemolysis leads to depletion of NO and this may attenuate nociceptive signaling through modulation of cGMP signaling.19 This has substantial implications for SCD where, as described in the following section, modulators of NO metabolism are being increasingly employed therapeutically. Expected outcomes are not obvious as additional signaling pathways modulate cGMP activity, and NO and cGMP production can decrease pain under some conditions.20 Some of this complexity is explained by the differential effects of modulating distinct sets of NO synthase genes and proteins.20 Thus, ultimately agents that specifically modulate distinct arms of these pathways in specific regions of the body (e.g., periphery versus central nervous system) will be required to extract the full clinical benefits of NO metabolism manipulation for SCD while maintaining the benefit of NO deletion on chronic pain.
Sepsis
See Appendix Figure 84-F for a detailed care plan.22 Sepsis is a major cause of morbidity and mortality in SCD. Strikingly, in the 1970s, 20% of SCD patients in the United States died before age 6 years, primarily from sepsis with encapsulated organisms, particularly Streptococcus pneumoniae.5 Many patients were diagnosed with SCD when presenting with sepsis, and diagnosed families often did not appreciate that fever in a toddler was an emergency. This led to newborn screening programs and the development of comprehensive care programs focused on education and immunizations. As expected, this did not affect the incidence of sepsis, but deaths from sepsis plummeted,43,44 and further decreased with the initiation of prophylactic penicillin in those younger than 6.45 Widespread use of pneumococcal and Hib vaccines further decreased severe pneumococcal disease to 2.3 events per 100 patient-years, with ages 6 to 36 months being the highest risk group.46,47
Pathophysiology and Etiology
The developmental timing of sepsis risk correlates with decreased splenic function because of infarcts leading to functional asplenia, which can be observed as early as 3 months. The resultant defects in cellular immunity, the alternate complement pathway and decrease in memory cells and opsonizing antibodies reduces clearance of encapsulated organisms. Together these lead to a lessened response to the 23-valent polysaccharide vaccine requiring routine reimmunization and results in a 100 to 400 times increased risk of bacteremia with encapsulated pathogens. SCD patients have shown a strong response to the conjugated PCV-7 vaccine, resulting in a two-thirds reduction in invasive pneumococcal disease.48 Serotypes not covered by the PCV-7 are increasing in prevalence, prompting the development of the PCV-13 vaccine. Although S. pneumoniae accounts for the majority of invasive infections other major pathogens include Haemophilus influenzae type b, Escherichia coli urosepsis, and Salmonella and Staphylococcus osteomyelitis.49
Management
See Figure 84-F for a detailed care plan.22 Although the pathophysiology, diagnosis, and management of sepsis are presented in Chapter 103, several aspects are unique to SCD, including (1) a focus on prevention with aggressive vaccinations with PCV-13, the conjugated 23-valent vaccine (and recurrent boosters from falling titers), Hib, and meningococcus50; (2) prophylactic penicillin given through age 6 years; (3) the critical role of family and provider education in seeking medical care and parenteral antibiotics emergently for fevers higher than 38.3° C. Pneumococcus has doubling time of 20 minutes; thus a 2-hour delay can result in a 50-fold increase in bacterial burden; (4) antibiotic choice should be driven by clinical presentation, but for empiric therapy ceftriaxone is preferred over cefotaxime and cefuroxime due to increasing resistance of pneumococcus; and (5) possible transfusion if cardiovascularly unstable. Evolving care practices will need to account for (1) the influence of hydroxyurea, (2) increasing antibiotic resistance, and (3) the recent release of the PCV-13 vaccine. (Hydroxyurea can result in regrowth of splenic tissue, and early use in young children may prevent autoinfarction, but how this correlates with preservation of immune function and resistance to encapsulated organisms is unknown.)
Acute Chest Syndrome
See Appendix Table 84-B for a detailed care plan.22 ACS is clinically heterogeneous and its definitions vary in the literature, making comparisons of studies difficult. The most general definition is a new nonatelectatic infiltrate on chest radiograph in a patient with SCD, though others add requirements for fever or respiratory symptoms (Figure 84-3). ACS is the second leading cause of hospitalization in SCD. ACS can progress in hours resulting in a mortality rate of 1% in children (vs. 4% in adults), with most deaths occurring in children younger than age 3 years.51 ACS accounts for 25% of deaths.51 Risk factors include a history of asthma, high baseline Hgb and low HbF. Factors associated with mortality include a prior episode of ACS, development of respiratory failure within 48 hours of presentation, sepsis, and simultaneous presentation with pain.51 Etiologies vary by age and included bacterial infections with typical and atypical organisms, viral infections, fat emboli, and pulmonary hemorrhage and multiple etiologic factors are often present (see also Appendix Figure 84-G).52
Presentation
ACS frequently occurs 2 to 3 days into a VOE. Consistent with the variety of etiologies, presenting signs and symptoms are variable and vary with age (e.g., young children are more likely to present with fever while older adolescents are more likely to present with chest pain).51 Notably, no single or pattern of signs and symptoms predicts ACS, and up to 35% of patients will have a normal pulmonary exam.51 Therefore there should always be a low threshold to obtain a chest radiograph. Infiltrates are most common in the lower lobes, and pleural effusions are more common in adults.51,52 Leukocytosis and significant drops in hemoglobin and platelets are common.51 Significant morbidity is associated with ACS including pneumothorax and empyema and 14% of patients developed respiratory failure in one study.52 Risk factors for respiratory failure include (1) extensive lobar involvement, (2) platelet count less than 199,000/µL and (3) history of cardiac disease.52
Management
See Appendix Table 84-B for a detailed care plan.22 Management of ACS can be addressed in stages; initial conservative interventions are followed by transfusion for clinical decline or if severely ill and finally biphasic positive airway pressure (BiPAP) or ventilatory support if needed. Initial care should consist of (1) antibiotics: a cephalosporin to cover encapsulated organisms, particularly S. pneumoniae, and a macrolide because Mycoplasma and Chlamydia are the most common infectious pathogens; (2) oxygen to maintain saturations more than 94%; (3) judicious fluid resuscitation to maintain euvolemia while avoiding overly aggressive fluid resuscitation that may worsen cardiac and respiratory status due to a combination of severe anemia and decreased cardiac function; and (4) prevention of atelectasis. Incentive spirometry (IS) has been shown to prevent progression, and chest physiotherapy and ambulation may be of benefit.53 Although IS is often limited by chest pain and patient compliance, use of a positive expiratory pressure (PEP) device has been shown to be as effective and better tolerated.54 The use of BiPAP or continuous positive airway pressure (CPAP) as a preventive measure is untested but appropriate as early intervention may prevent the need for intubation. (5) Opiates should be carefully titrated to minimize splinting and allow IS, while minimizing respiratory depression (small opiate boluses before IS may be helpful). Pain relief can improve respiratory mechanics, improving clinical status. (6) Bronchodilators are indicated as asthma is common in SCD and its presence increases the risk of ACS, and a subset of patients respond independent of documented wheezing.55 (7) The use of corticosteroids remains controversial. Although a randomized trial showed a decrease in hospital stay and transfusion, a subsequent retrospective review revealed a higher readmission rate, independent of a history of asthma.56,57
Transfusion has been shown to significantly improve oxygenation and clinical status in ACS.52 Although transfusion is usually effective at reversing ACS, because of the risks, including alloimmunization, transfusion not part of initial management unless severely ill. For most patients serial observations, with a low threshold for repeat chest radiographs is appropriate; reserving transfusion for progression despite conservative management. The decision of when to transfuse is contentious and data have been lacking. Promising studies suggest elevated secretory phospholipase A2 (sPLA2) levels predict the development of ACS in SCD.58 sPLA2 cleaves phospholipids, releasing free fatty acid, and is increasingly being associated with cardiovascular and pulmonary damage, including meconium aspiration. A randomized trial suggests early transfusion guided by sPLA2 levels, can prevent ACS.58 Though promising, sPLA2 assays are not routinely available and their role in management remains to be determined.
Although 20% to 70% of patients with ACS are transfused, views differ on performing a simple transfusion targeting a Hgb of approximately 10, or an exchange transfusion targeting the same Hgb while lowering the HbS to under 30%.51,52 Although both approaches improve oxygenation and are safe and effective, exchange transfusion requires exposure to more donors, increasing allo-immunization, is more time consuming, and may require central access in this population with increased thrombotic risk. Thus a simple transfusion is recommended for most situations.59,60 The decision between direct and exchange transfusion in worsening ACS patients with a high Hgb (>9 g/dL) remains unclear as a minimal amount of red cells can be transfused.
Although lacking formal studies, the use of BiPAP or CPAP in an attempt to stave off intubation makes sense pathophysiologically. There is no consensus as to when to intubate or the optimal ventilation strategy in ACS, thus patients are often treated as children with acute lung injury or ARDS. For those patients with refractory hypoxemia, both high-frequency oscillatory ventilation and venovenous extracorporeal membrane oxygenation have been successful.61–63 Several promising approaches to ACS including improving NO metabolism with inhaled NO or oral arginine, a precursor of NO; and inhibitors of sPLA2.64
Patients with ACS have developed plastic bronchitis. A review of 29 children hospitalized with ACS found 72% had plastic bronchitis, one requiring mechanical ventilation, suggesting there may be a role for intratracheal DNase.65,66
Stroke
See Appendix Table 84-C for a detailed care plan and Appendix Figure 84-H for a diagnostic approach.22 One of the most debilitating and under-appreciated complications of SCD is a cerebrovascular accident (CVA), or the acute disruption of cerebral blood supply resulting in loss of brain tissue function lasting longer than 24 hours.67 In contrast, transient ischemia attacks resolve within 24 hours, and usually less than 1 hour. This section will focus on CVA related to SCD; a detailed discussion of stroke can be found in Chapter 63. Before screening and prevention programs, 11% of children with HbS/S or HbS/β-thalassemia developed overt strokes (peak, 2 to 9 years), with another 17% having silent infarcts.68,69 Risk factors include anemia, moya moya, history of CVA, and high velocities upon transcranial Doppler (TCD) ultrasound.70 Additional risks for ischemic stroke include ACS and hypertension, whereas leukocytosis is a risk for hemorrhagic stroke. Patients with HbS/C disease and other compound heterozygotic states do not have such a significantly increased risk. Strokes are heterogeneous, being due to ischemia, hemorrhage, or thromboembolic events and involving large, medium, and small vessels. The type of CVA varies by age, with ischemic events more common in the first decade and hemorrhagic events more common after age 20 years. Pathophysiology varies tremendously depending on the type and site of stroke, for example anemia is associated with watershed infarcts, whereas thrombus formation and emboli affect more midsize to small vessels, and hemorrhagic strokes likely result from intimal hyperplasia and dilation at the site of previous insults.
Natural History
The natural history of overt ischemic infarcts has changed dramatically with screening TCDs and chronic transfusions. If untreated, 67% of patients have a recurrent infarct within 9 years, and this is reduced to 10% with chronic transfusions targeting a HbS of under 30%.71–73 Screening TCDs using a standardized approach identifies patients with high cerebral vessel flow, associated with an excessively high stroke risk within 3 years.74 This can now be decreased to 10% with chronic transfusions.75 Although physical manifestations often show significant improvement after a stroke, persistent neurocognitive abnormalities are common. A subgroup of patients have moya moya, multiple constrictions of large arteries leading to collaterals at high risk of hemorrhage, aneurysm and clot (see Appendix Video 84-1). This group may have particularly severe disease and may represent some of the 10% resistant to chronic transfusions. The natural history of hemorrhagic infarcts varies in large part with their severity at presentation and location, because these dictate possible neurosurgical or radiology-guided interventions. Silent infarcts can result in difficulties with academic attainment and achievement and cognitive dysfunction similar to that in overt strokes.76 Executive function deficits are most common, having a tremendous effect on a patient’s function in society, and ability to navigate the health care system.

Full access? Get Clinical Tree
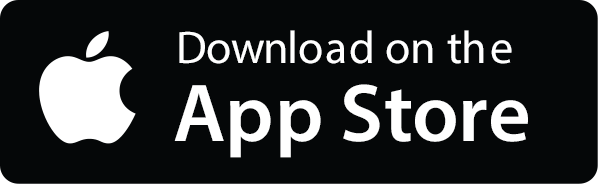
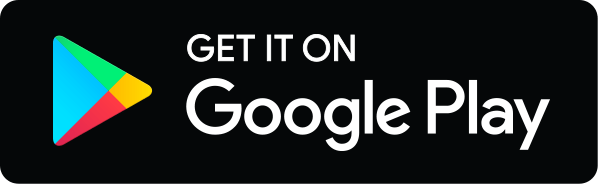