- •
Proactive treatment measures for tumor lysis syndrome include hydration with hypotonic or isotonic saline solution; alkalinization; use of allopurinol or urate oxidase; avoidance of exogenous potassium; close monitoring of fluid status; and frequent monitoring of serum potassium, sodium, chloride, bicarbonate, calcium, phosphorus, uric acid, blood urea nitrogen, and creatinine concentrations.
- •
Clinically significant hyperleukocytosis occurs with a white blood cell count greater than 200,000/µL in acute myeloid leukemia and greater than 300,000/µL in acute lymphocytic leukemia.
- •
Concurrent thrombocytopenia and hyperleukocytosis increase risk of adverse outcomes, including intracranial hemorrhage and death. Aggressive therapy to correct coagulopathy with fresh frozen plasma and vitamin K and to maintain platelet count higher than 20,000/µL is critical.
- •
Childhood mediastinal masses produce few symptoms until they have occluded a substantial portion of the cross-sectional area of the trachea or mainstem bronchi or the superior vena cava. Diagnosis should be established with the least invasive means available because these patients are at significant risk for anesthetic complications.
- •
Spinal cord compression most frequently occurs as metastatic disease and requires emergent treatment with 1 mg/kg of dexamethasone over 30 minutes, as well as neurosurgical consultation.
- •
Hemophagocytic lymphohistiocytosis produces severe illnesses that may be confused with other forms of systemic inflammatory response syndrome, such as sepsis. Appropriate diagnosis is tantamount; if the condition is left untreated, it is almost always fatal.
Hematologic emergencies
Anemia
Anemia results from a deficiency in the oxygen-carrying capacity of the blood. The deficit may be in the number of red blood cells (RBCs), the RBC hemoglobin (Hb) concentration, or both. Because Hb serves as the primary transport molecule for oxygen (O 2 ), anemia may affect the delivery of O 2 to the tissues, with wide-ranging potential complications. Under steady-state conditions, O 2 consumption remains constant and is independent of O 2 delivery until it falls below a critical level, which varies for each organ system. When the Hb concentration decreases to approximately 5 g/dL or less, O 2 delivery and consumption may be compromised. Below this level, O 2 consumption becomes delivery dependent. The body responds to acute, normovolemic anemia by increasing cardiac output through increases in stroke volume, heart rate, or both. As the hematocrit falls, blood viscosity diminishes, increasing venous return and augmenting preload. In the patient with chronic anemia, increases in cardiac output are supplemented by increased levels of 2,3-diphosphoglycerate shifting the oxyhemoglobin curve to the right and augmenting O 2 delivery at the tissue level. Anemias generally are classified either by the mechanism resulting in the Hb deficit—decreased production, accelerated destruction, or loss of erythrocytes—or by the morphologic appearance of the erythrocyte, including size, Hb concentrations, and shape variation ( Table 92.1 ). The normal values for mean corpuscular volume and mean corpuscular Hb concentration vary with the child’s age. A wide variety of conditions, some intrinsic to the erythrocyte and others related to extrinsic factors, may result in abnormal RBC morphology. The more common causes of profound anemia encountered in the pediatric intensive care setting are reviewed here. Regardless of cause, transfusion of pediatric critical care patients has received intensive investigation, and recommendations for transfusion have emerged balancing the benefit of increased RBC O 2 -carrying capacity with potential risks. Current recommendations for packed red blood cell (PRBC) transfusion to subgroups of pediatric patients share a goal of reducing the frequency and volume of PRBC transfusions; in most cases, transfusion is indicated for Hb 5 g/dL or less and not indicated for Hb 7 g/dL or greater, with use of clinical judgment for values between these thresholds (see also Chapter 91 ).
Mechanism and Examples | Changes in Laboratory Data |
---|---|
Loss or Destruction | |
Bleeding | ↑ Reticulocyte count |
Autoimmune hemolytic anemia | ↑ Reticulocyte count |
Hereditary spherocytosis | ↑ LDH |
Sickle cell anemia | ↑ Indirect bilirubin ↓ Haptoglobin |
Poor Production | |
Anemia of chronic inflammation | Normal or ↓ MCV ↓ Reticulocyte count |
Pure red cell aplasia | ↑ MCV ↓ Reticulocyte count |
Aplastic anemia | ↑ MCV ↓ Reticulocyte count ↓↑ Neutropenia ↑↓ Thrombocytopenia |
Nutritional Deficiency | |
Iron deficiency | ↑ MCV ↓ Reticulocyte count ↓ Ferritin ↓ Iron saturation ↑ TIBC |
Folate deficiency | ↑ MCV |
Vitamin B 12 deficiency | ↑ Reticulocyte count |
Hemorrhagic anemia
Bleeding may be either acute and normocytic or chronic, which becomes microcytic due to depletion of iron stores. In response, physiologic mechanisms to increase O 2 delivery and tolerate Hb levels well below the normal range include tachycardia, increased stroke volume, and augmented contractility. Signs of significant cardiovascular compromise may not become evident until the child has lost at least 25% of total blood volume, when patients usually manifest age-appropriate systolic hypotension. Signs and symptoms of acute hemorrhage result from poor end-organ perfusion, with consequent diminished O 2 delivery. However, signs of impending shock—such as pallor, anxiety, and tachypnea—may be subtle. Initial management should include achieving hemostasis, establishing a secure airway, maintaining ventilation, and initiating volume replacement via an adequate intravenous catheter.
RBC transfusion should be given if O 2 delivery to the end organs is inadequate. Either whole blood or PRBCs can be used, but the former has many difficulties related to storage and transport (see also Chapter 91 ). If PRBCs or plasma-poor red cells are used to correct O 2 -carrying capacity during massive blood loss, deficits of coagulation factors develop earlier than during transfusion of whole blood. Hypofibrinogenemia generally develops first, followed by deficits in other clotting factors and later by thrombocytopenia. Fresh frozen plasma, platelets, and cryoprecipitate should be used to treat coagulopathy that develops during replacement of massive blood loss with PRBCs. Other clinical abnormalities associated with replacement of large volumes of RBCs include hyperkalemia, hypocalcemia, and transfusion reactions. Transfusion of platelets should be guided by serial platelet counts. Central venous pressure should be monitored to allow for rapid administration of RBCs and volume replacement while decreasing the risks of hypervolemia. Blood and other fluids may be administered rapidly until central venous pressure rises to 6 to 7 mm Hg.
Anemia secondary to decreased production
Screening laboratory tests are invaluable in distinguishing the types of anemia associated with diminished blood cell production: reticulocyte count, red cell size, and evaluation of other cell lines in addition to a careful history direct further evaluation. Nutritional deficiencies typically involve either microcytic (iron) or macrocytic (B 12 , folate) isolated RBC anemia. Anemia of chronic disease, microcytic or normocytic, is extremely common and is the end product of several processes. Inflammation increases expression of hepcidin, a key regulator of iron metabolism, and interleukin-6 (IL-6), a proinflammatory cytokine. Through its effect on ferroportin, hepcidin activity leads to decreased gastrointestinal iron absorption and increased iron sequestration in macrophages, reducing its availability for erythropoiesis. IL-6 promotes iron sequestration by upregulation of hepcidin via the JAK/Stat pathway. Other proinflammatory cytokines—such as IL-1, tumor necrosis factor-α, and interferon-γ, seem to inhibit erythropoiesis by impairing erythropoietin sensitivity and erythroid lineage differentiation. Currently, the most effective intervention for anemia of chronic inflammation is to treat the underlying disease.
Anemia associated with thrombocytopenia represents a broad array of clinical entities ( Box 92.1 ), most commonly acquired aplastic anemia, defined by at least two of the three following factors: granulocytes less than 500/µL, platelets less than 20,000/µL, and anemia with a corrected reticulocyte count less than 1% in conjunction with markedly hypocellular bone marrow. The majority of cases have no definable cause but the pathophysiology appears immune mediated, with destruction of blood-forming cells by lymphocytes. Irrespective of the etiology of bone marrow failure, life-threatening complications may arise from blood cytopenias. The most common causes of death are bacterial sepsis and fungal infection secondary to refractory granulocytopenia. Broad-spectrum antibiotics should be used to treat suspected infection in the granulocytopenic patient. Historically, Gram-negative organisms were the most frequent cause of fulminant infection in this patient population. With the increased use of central venous catheters, Gram-positive organisms now predominate. Antifungal therapy should be instituted in patients who fail to defervesce within 3 to 5 days of treatment with antibiotics.
A. Isolated anemia
- 1.
Anemia of chronic inflammation
- 2.
Congenital sideroblastic anemia
- 3.
Transient erythroblastopenia of childhood
- 4.
Paraneoplastic
- 5.
Autoimmune
- 6.
Infections
B. Complex anemia
- 1.
Diamond-Blackfan anemia
- 2.
Fanconi anemia
- 3.
Dyskeratosis congenital
- 4.
Shwachman-Diamond syndrome
- 5.
Acquired aplastic anemia
Platelet transfusions should be used judiciously in an effort to avoid alloimmunization to platelet antigens and are generally reserved for episodes of active bleeding. Similarly, RBC transfusions should be reserved for patients whose O 2 delivery may be compromised as a result of profound anemia. The patient should not receive blood products donated by family members to avoid sensitization to leukocyte and platelet antigens of potential bone marrow donors. All blood products should be irradiated and leukodepleted to decrease the risk of graft-versus-host disease, with preference given to single-donor apheresis units. Treatment of severe acquired aplastic anemia involves either the use of immunosuppressive therapy or replacement of bone marrow through stem cell transplantation. The treatment of choice, bone marrow or peripheral blood stem cell transplantation from a histocompatible sibling, produces long-term survival rates of 75% to 80%. Unfortunately, up to 70% of patients may lack a suitably matched sibling donor. Stem cells harvested from a matched unrelated donor, or umbilical cord blood, produce poorer outcomes because of the higher rate of graft-versus-host disease. For these patients, immunomodulation, which usually includes a combination of antithymocyte globulin and cyclosporin, often with use of hematopoietic growth factors, has resulted in response rates of 70% to 80%.
Hemolytic anemia
Hemolysis, the destruction of RBCs with liberation of Hb, may occur within either the blood vessels (intravascular hemolysis) or the reticuloendothelial system (extravascular hemolysis). Anemia results when the rate of RBC destruction exceeds new RBC production in the bone marrow. Laboratory findings in patients with hemolytic anemia usually include increased reticulocyte count and elevated serum concentrations of unconjugated bilirubin and lactate dehydrogenase. Intravascular hemolysis usually results in decreased serum haptoglobin concentrations.
Premature destruction of RBCs may result from intrinsic RBC abnormalities, such as hemoglobinopathies from red cell membrane defects or from a variety of extrinsic factors (see Table 92.1 ). Numerous Hb variants resulting in shortened RBC survival have been identified. Individuals with sickling hemoglobinopathies may suffer a variety of complications that require treatment in an ICU (see Chapter 89 ). Abnormalities in the structure of the RBC membrane, as in hereditary spherocytosis, or decreased quantities of RBC enzymes, as in glucose-6-phosphate dehydrogenase (G6PD) deficiency, also decrease red cell survival. Hemolysis in these settings occurs primarily extravascularly. Mechanical disruption of the red cell membrane secondary to factors extrinsic to the RBC may lead to macroangiopathic hemolytic anemia, as with turbulent flow around a prosthetic heart valve, or microangiopathic hemolytic anemia, caused by fibrin deposition in the microvasculature. The latter process is seen in consumptive disorders, including disseminated intravascular coagulation (DIC), hemolytic uremic syndrome (HUS), and thrombotic thrombocytopenic purpura (TTP). In these entities, hemolysis is primarily intravascular, with characteristic schistocytes seen on the blood smear.
The hemolytic processes that result from abnormal interactions between erythrocytes and the immune system are known collectively as autoimmune hemolytic anemia (AIHA). AIHA can be classified as either primary, in which there is no identifiable systemic illness except possibly a history of a recent viral-like illness, or secondary, in which the hemolytic anemia is present in the context of another illness. AIHA has been reported as a manifestation of autoimmune disorders (e.g., lupus erythematosus), immunodeficiency disorders, malignancies, specific infections, or as a drug reaction ( Box 92.2 ). AIHA also may be classified by the thermal sensitivity of the autoantibodies. The most common form is the result of warm-reactive immunoglobulin G (IgG) autoantibodies directed against RBC membrane proteins. Extravascular hemolysis occurs, with sensitized erythrocytes cleared primarily in the spleen. Cold-agglutinin disease, the second most common form of AIHA, most frequently occurs with Mycoplasma pneumoniae infections, but it also is associated with other infectious agents, including Epstein-Barr virus, cytomegalovirus, and mumps virus. In this disorder, IgM autoantibody binds to the RBC and fixes complement. The erythrocytes may undergo intravascular hemolysis, or they may be cleared by the reticuloendothelial system, primarily in the liver. Paroxysmal cold hemoglobinuria is a rare variant of AIHA that usually occurs following a viral illness and in which an IgG autoantibody binds at cold temperature to the P-antigen of the erythrocyte, fixing complement (the Donath-Landsteiner antibody) and producing intravascular hemolysis. Although drug-induced autoantibodies occur uncommonly in children, they may follow exposure to some antibiotics, including penicillins and cephalosporins. Mechanisms of drug-induced hemolysis may include autoantibody formation and adsorption of the drug onto the red cell membrane, with immune complex formation with IgG or IgM.
A. Primary AIHA
- 1.
Warm-reactive autoantibodies, usually IgG
- 2.
Paroxysmal cold hemoglobinuria, usually IgG
- 3.
Cold agglutinin disease, usually IgM
B. Secondary AIHA
- 1.
Systemic autoimmune disease (e.g., lupus)
- 2.
Malignancy (Hodgkin and non-Hodgkin lymphoma)
- 3.
Immunodeficiency
- 4.
Infection ( Mycoplasma , viruses)
- 5.
Drug induced
Patients with AIHA usually present with pallor, jaundice, and splenomegaly on physical examination. The reticulocyte count is generally elevated, although initially it may be low or normal. The direct antiglobulin test (Coombs test) demonstrates the presence of antibodies or complement on the red cells. The indirect antiglobulin test measures the presence of unbound antierythrocyte antibodies in the patient’s serum.
Therapy depends on the type of AIHA and the severity of clinical symptoms. Profound anemia, usually with a Hb level of less than 5 g/dL, may result in cardiovascular compromise and requires erythrocyte transfusion to increase O 2 -carrying capacity. The presence of autoantibodies often makes cross-matching blood difficult, and the patient may require transfusion with “least incompatible” blood. Significant hemolytic transfusion reactions are infrequent. However, severe hemolysis occurs on rare occasions, with hemoglobinemia and hemoglobinuria resulting in renal failure. Therefore, transfusions should be started at a slow rate, and both plasma and urine samples should be checked regularly for free Hb. Patients with cold-reactive antibodies should be kept warm, and a blood warmer should be used for the transfused blood. Even in the absence of transfusion, significant intravascular hemolysis may occur in patients with cold-reactive antibodies. Maintaining good renal blood flow and careful monitoring of urine output in this setting may help obviate renal injury. Corticosteroids appear to slow the hemolytic process, particularly in patients with IgG autoantibodies, in whom this drug class appears to inhibit Fc receptor-mediated clearance of sensitized erythrocytes. The usual dosage is 1 to 2 mg/kg methylprednisolone administered intravenously every 6 hours until the patient is clinically stable. The patient then can be switched to oral prednisone (2 mg/kg per day for 2 to 4 weeks, followed by a slow taper over 1 to 3 months).
Thrombocytopenia related to decreased platelet production
Decreased platelet production may result from primary bone marrow failure states or from bone marrow infiltration by malignant cells, as in leukemia, lymphoma, and metastatic solid tumors. Bone marrow suppression—a common side effect of antineoplastic therapy, including both chemotherapy and radiotherapy—frequently leads to periods of thrombocytopenia with duration dependent on the regimen. Patients with hematologic malignancy, on average, receive 1.5 platelet transfusions (range, 0–3) during each chemotherapy cycle. In patients who have undergone hematopoietic stem cell transplantation, the time period until platelet recovery ranges from 10 to 45 days, and they receive 5 to 17 platelet transfusions, on average, depending on whether they received an autologous or an allogeneic unrelated donor source. Despite supportive platelet transfusion therapy, bleeding continues frequently in this population, with the most common sites being minor bleeding of skin and mucous membranes. Clinical manifestations include petechiae and purpura, epistaxis, gastrointestinal bleeding, hematuria, and menorrhagia. Intracranial hemorrhage is an infrequent manifestation of thrombocytopenia. The importance of residual bleeding despite prophylactic platelet transfusion was demonstrated in the Platelet Dose Study. An analysis of the 200 patients ages 18 years or younger in the study found that bleeding (≥2 World Health Organization [WHO] grade) was higher in children compared with adults. In fact, the rate of bleeding was highest in the youngest patient cohorts; 86% in 0- to 5-year-olds, 88% in 6- to 12-year-olds, and 77% in 13- to 18-year-olds, compared with 67% in adults. Pediatric patients also had more days with grade 2 or higher WHO bleeding compared with adults. There is early support for the use of antifibrinolytic therapy with either tranexamic acid or ε amino caproic acid (EACA) infusion, which results in clinical improvement in ongoing bleeding and allows reduction in platelet usage. Guidelines for platelet transfusion vary with the underlying cause of thrombocytopenia and the patient’s clinical status. Patients with primary bone marrow failure, who likely will experience prolonged thrombocytopenia, generally receive transfusions only for active bleeding because of the risk of alloimmunization. In addition, exposure to multiple platelet donors may jeopardize the success of bone marrow transplantation by increasing the risk of graft rejection. The threshold for transfusion may need to be higher in patients with sepsis, decreased humoral coagulants, or other risk factors. In the perioperative setting, platelet counts should be maintained at greater than 50,000/dL and greater than 100,000/dL for neurologic or ophthalmologic surgery. Use of ABO-compatible donors and leukoreduction diminishes the risk of platelet alloimmunization. Single-donor apheresis units reduce donor exposure compared with pooled platelet concentrates, but whether such usage reduces the incidence of platelet alloimmunization remains unclear.
Thrombocytopenia-related immune-mediated consumption
Immune-mediated platelet destruction may be caused by autoantibodies, drug-induced antibodies, or alloantibodies. Alloantibodies result from exposure to polymorphic epitopes expressed on foreign platelets to which the patient has been exposed (see the previous section). Drug-induced thrombocytopenia may be suggested by the patient’s medication history, most commonly associated with heparin, which may be confirmed by laboratory tests for specific drug-associated antiplatelet antibodies.
In immune thrombocytopenia purpura, now renamed with the same acronym for continuity to immune thrombocytopenia (ITP), autoantibodies to platelets and megakaryocyte antigens are generated with other autoimmune disorders, immunodeficiency states, or after viral illness or immunization or without predisposing condition identified. The incidence of childhood ITP ranges from 5 to 7 per 100,000 per year, with 25% of affected children subsequently developing chronic ITP. The reticuloendothelial system removes antibody-coated platelets, with the bulk of the destruction occurring in the spleen. These children typically present with petechiae, purpura, bleeding from mucous membranes, and isolated thrombocytopenia. There is also impaired platelet production, in which immature, large platelets enter the blood, thought to prevent serious bleeding due to the increased effectiveness of platelets. The primary goal of therapy in children with ITP is to limit bleeding, especially from the central nervous system, and await spontaneous resolution, with no intervention in cases of mild or absent bleeding regardless of platelet count. There is no evidence that pharmacologic intervention prevents hemorrhage, and the majority of children will undergo spontaneous remission. Consensus guidelines for the definition and treatment of ITP, directed to slow clearance of sensitized thrombocytes in the spleen and reduce antibody production, include first-line therapy for high-risk patients or those with more than mild bleeding of a single dose or short course of corticosteroids or intravenous immunoglobulin (IVIG). Infusion of anti-Rh(D) IG (50–75 µg/kg) for individuals who have Rh(D)-positive RBCs prolongs survival of antibody-coated platelets in patients with ITP but should be avoided in patients who present with anemia. As with IVIG, the major mechanism appears to include blockage of Fc receptors on reticuloendothelial cells. Use of these agents usually halts bleeding and raises platelet counts to safe levels within a few days, although evidence indicating their influence on the course of the disease remains lacking. Second-line agents include rituximab, splenectomy, and high-dose dexamethasone. Intracranial hemorrhage remains rare, and there are no data that treatment actually reduces the incidence of intracranial hemorrhage. Bone marrow evaluation, routine screening for antiplatelet antiphospholipid and antinuclear antibodies, Ig levels, and other platelet parameters are no longer considered necessary if careful history, physical examination, and review of blood count and smear are consistent with ITP. Intracranial hemorrhage, a devastating but rare complication of ITP, requires immediate intervention. Consequently, patients presenting with headaches, persistent vomiting, or neurologic symptoms require an emergent computed tomography (CT) scan of the head. Therapy for intracranial hemorrhage in the setting of ITP includes IVIG, corticosteroids, and intermittent (2–4 IU/m 2 every 6–8 hours) or continuous (0.5–1.0 IU/m 2 per hour) platelet transfusions administered for life-threatening hemorrhage, with decreases in bleeding reported. Plasmapheresis, splenectomy, and romiplostim, a thrombopoietin receptor agonist, may be beneficial in patients who do not respond to these interventions.
Thrombocytopenia related to nonimmune consumption
Reduced numbers of platelets may be concomitant with cellular injury from a broad array of causes, resulting in tissue or endothelial injury. Systemic response patterns involve the balance of procoagulant and anticoagulant factors, with cross-activation among coagulation, innate immunity, and inflammatory responses. DIC is the result of an excess of thrombin generation by inciting factors to overwhelm the hemostatic process, which then disseminates. Generalized activation of the plasma coagulation pathways occurs within small blood vessels with formation of fibrin and depletion of circulating levels of clotting factors and platelets. DIC usually follows a systemic insult—most often sepsis, trauma, or shock; treatment should be directed to the underlying cause. Hemorrhage frequently occurs at platelet counts higher than 10,000/µL because of concomitant depletion of clotting factors, requiring platelet and plasma transfusions. DIC is suspected to contribute to the development of multiorgan system failure in intensive care unit (ICU) patients, with formation of a large number of microthrombotic foci leading to organ microcirculation failure and subsequent failure of the organ itself (see Chapter 111 ). However, thrombocytopenia may be a marker of poor prognosis rather than a cause of ICU mortality.
Many common disorders are associated with development of microangiopathic hemolytic anemia and thrombocytopenia, including cancer, sepsis, organ transplantation, autoimmune disorders and eclampsia syndromes. The thrombotic microangiopathy (TMA) syndromes are diverse, hereditary or acquired, and acute or chronic, but all share microangiopathic hemolytic anemia, thrombocytopenia, and organ injury. The four hereditary TMA disorders, Shiga toxin-mediated TMA (ST-HUS) and TMA associated with transplant, are the most common disorders in pediatric patients. Most TMAs (with the exception of TTP, which is uncommon in children) share hallmarks of kidney injury, hemolytic anemia, and thrombocytopenia. Increasing recognition of these syndromes has led to an awareness of specific interventions depending on the form of TMA present. Depending on the type of TMA, therapeutic approach varies but may include plasma exchange, removal of inciting drug, immunosuppression, vitamin supplementation, and supportive care ( Table 92.2 ). Specific management of HUS is described in Chapter 74 .
Defect | Treatment | |
---|---|---|
Hereditary Disorders | ||
ADAMTS13 deficiency-mediated TMA (also called TTP) | Homozygous or compound heterozygous ADAMTS13 | Plasma infusion |
Complement-mediated TMA | Mutations in CFH , CFI , CFB , C3 , CD46 , and other complement | Plasma infusion or exchange, anticomplement agent |
Metabolism-mediated TMA | Homozygous mutations in MMACHC (encoding methylmalonic aciduria and homocystinuria type C protein) | Vitamin B 12 , betaine folinic acid |
Coagulation-mediated TMA | Homozygous mutations in DGKE ; mutations in PLG , THBD (?) | Plasma infusion |
Acquired Disorders | ||
ADAMTS13 deficiency–mediated TMA (also called TTP) | Autoantibody inhibition of ADAMTS13 activity | Plasma exchange, immunosuppression |
Shiga toxin–mediated TMA (also called ST-HUS) | Enteric infection with Shiga-toxin strain of E. coli or Shigella | Supportive care |
Drug-mediated TMA | Quinine and possibly other drugs | Removal of drug, supportive care |
Drug-mediated TMA (toxic dose–related reaction) | VEGF inhibition, other mechanism | Removal of drug, supportive care |
Complement-mediated TMA | Antibody inhibition of complement factor H activity | Plasma exchange, immunosuppression, anticomplement agent |

Full access? Get Clinical Tree
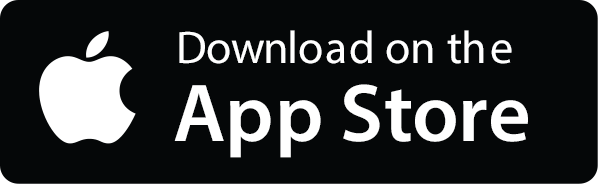
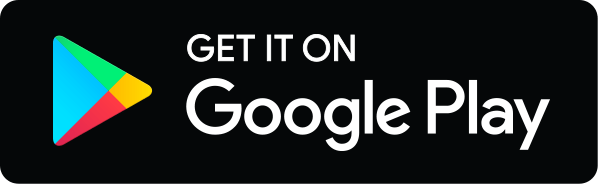
