Chapter 34
Hematology and Anesthesia
The Normal Vessel Wall
The normal blood vessel acts as a conduit to maintain a state of fluidity within the vascular system. Blood vessels are cylindrical and consist of three distinct layers—the intima, the media, and the adventitia (Figure 34-1).
The intima (the inner layer) is the lining separating the flowing blood from the vessel; it is made up primarily of endothelial cells. These endothelial cells play an important role in the modulation of hemostasis by synthesizing and secreting many procoagulants (initiators of coagulation), anticoagulants (inhibitors of coagulation), and fibrinolytics (to dissolve the clot) (Table 34-1). One of the important mediators, von Willebrand factor (vWF), is a necessary cofactor for the adherence of platelets to the subendothelial layer. Tissue factor (a cofactor from the coagulation cascade) activates the clotting cascade pathway when injury to the vessel occurs. Some mediators (e.g., thromboxane A2, adenosine diphosphate [ADP]) control blood flow by influencing vasoconstriction. Other mediators (e.g., nitric oxide, prostacyclin) control blood flow by vasodilation of blood vessels. Endothelial cells also can suppress activation of the coagulation system by their expression of many coagulation inhibitors such as tissue factor pathway inhibitor.1
TABLE 34-1
Mediators Responsible for Procoagulant, Anticoagulant, and Fibrinolytic Activities
Property | Mediator | Function |
Procoagulant | Coagulation factors Collagen vWF Protein C Protein S Fibronectin Thrombomodulin | Coagulation Tensile strength Adhesion Degrades V and VII Cofactor for protein C Mediates cell adhesion Regulates anticoagulant pathway |
Anticoagulant | Antithrombin III | Degrades factors XII, XI, X, IX, & II |
Tissue pathway factor inhibitor | Inhibits tissue factor | |
Vasodilation | Nitric oxide Prostacyclin | Vasodilates Vasodilates, inhibits aggregation Both promote smooth-muscle relaxation |
Vasoconstriction | Thromboxane A2 ADP Serotonin | Vasoconstriction Vasoconstriction Vasoconstriction |
Fibrinolytic | Plasminogen tPA Urokinase | Converts to plasmin Activates plasmin Activates plasmin |
Antifibrinolytic | Plasminogen activator inhibitor | Inactivates tPa, urokinase |
α-Antiplasmin | Inhibits plasmin |
ADP, Adenosine diphosphate; tPA, tissue plasminogen activator; vWF, vonWillebrand factor.
The third layer, the adventitia, participates in the control of blood flow by influencing the vessel’s degree of contraction. The endothelial cells produce nitric oxide and prostacyclin, which influence the adventitia. Nitric oxide affects platelet function by inhibiting platelet adhesion, aggregation, and the binding of fibrinogen between glycoprotein IIb/IIIa complex (GpIIb-IIIa) pseudopods. The ability of nitric oxide to influence and promote smooth-muscle relaxation results in vascular vasodilation. Once the vessel vasodilates, the increase in blood flow limits the activity of procoagulant mediators by simply washing the procoagulant mediators away. This metabolic reaction occurs within the endothelial lining (Figure 34-2). Under the influence of nitric oxide synthetase (NOS), l-arginine is converted to nitric oxide (NO). Nitric oxide then diffuses into the muscle cells and activates soluble guanylate cyclase, subsequently producing a second messenger, cyclic guanosine monophosphate, causing muscle relaxation.1
Platelets are an essential component of the thrombogenic response to bleeding. Platelets are round and disklike and circulate freely within the blood. They are formed in the bone marrow from megakaryocytes, maintain a concentration count of approximately 150,000 to 300,000/mm3, and survive approximately 8 to 12 days.2 Platelets are constantly working to “patch” thousands of minute vascular injuries that occur in perpetuity. Approximately 7.1 × 103 are used each day.3
The platelets flow along the vessel surface. Because they are smaller than some other constituents in fluid blood (e.g., red blood cells [RBCs], white blood cells [WBCs]), they tend to be pushed aside, strategically positioned near the vessel-wall surface where they can then “react” in the event of injury (Figure 34-3).
The membrane surface of the platelet serves as a physical barrier between platelet cytoplasm and the surrounding plasma. Platelets contain mitochondria in their cytoplasm, enabling them to participate in aerobic metabolism, and have glycogen stores that allow for anaerobic metabolism.4 Platelets also contain contractile proteins, store large amounts of calcium and various enzymes, and require the use of their phospholipids’ surface to promote cellular activity. Platelets contain alpha (α) granules that store proteins (e.g., vWF, fibrinogen, fibronectin, platelet factor 4, and platelet growth factor) and dense granules that store nonproteins (e.g., serotonin, ADP, adenosine triphosphate [ATP], histamine, and epinephrine).2 Many of these granules synthesize prostaglandins that enable the platelets to promote vascular and local tissue reactions.2,4 Platelets also produce thrombin. In the platelet, thrombin’s role is to activate some of the coagulation factors and to influence recruitment of platelets to the site of injury. All the contents in the cytoplasm of the platelet participate in regulating hemostasis. Platelets do not contain a nucleus, RNA (ribonucleic acid), or DNA (deoxyribonucleic acid), so they do not reproduce.2
Vessel Injury
The vessel wall immediately contracts to cause a tamponade, decreasing blood flow. This contraction is a result of autonomic nervous system reflexes and the expression of thromboxane A2 and ADP.2,5 The area adjacent to the injury vasodilates and distributes blood to the surrounding organs and tissues. Contraction is followed by three separate stages in the formation of a primary plug: adhesion, activation, and aggregation.
In the adhesion stage, vWF mobilizes from within the endothelial cells and emerges from the endothelial lining. Glycoprotein Ib (GpIb) receptors emerge from the surface of the platelet (Figure 34-4). The purpose of GpIb is to attach to vWF and attract platelets to the endothelial lining; vWF makes platelets “sticky” and allows them to adhere to the site of injury.
Under the influence of tissue factor (a cofactor of the extrinsic clotting pathway), the platelet then undergoes a conformational transformation as it becomes activated (Figure 34-5). The once disklike structure swells and becomes oval and irregular. From the platelet surface, two other major glycoproteins, IIb and IIIa, project themselves outward. The purpose of the GpIIb-IIIa receptor complex is to link other activated platelets together in an effort to form a primary platelet plug. When this action is complete, the platelets form a mound whose only goal is to seal and heal the site of injury within the blood vessel.
As platelets undergo this metamorphosis, they release the alpha and dense granules, the contractile granules, thrombin, and many important mediators into the blood in an effort to promote procoagulant activity. All these mediators are responsible for platelet aggregation to form a primary unstable clot. When injury is minute and less threatening, this primary plug is enough to maintain hemostasis. When the injury is large, activation of the coagulation clotting cascade is required for permanent repair to create and stabilize a secondary clot to cease bleeding.2
The coagulation cascade illustrates the activation of cofactors (also referred to as zymogens) and their role in this process of hemostasis. Most cofactors are enzymes, with some exceptions (e.g., factors V and VIII). The coagulation factors circulate as inactive cofactors until they are activated to assist in the process of coagulation (Table 34-2). Activation of cofactors results from either tissue or organ damage and sets in motion a process that terminates in stabilization of hemorrhagic conditions in the absence of pathology. The factors are identified with Roman numerals for ease of interpretation.
The clotting pathways are thought to be two separate and distinct pathways (extrinsic and intrinsic) that worked independently of each other but in conjunction with platelet activity and the common coagulation pathway (Figure 34-6). The extrinsic pathway (tissue factor pathway) became activated by the release of tissue factor when injury occurred outside the vessel wall (with organ trauma or crushing injuries). This section of the coagulation cascade consisted of factor III (tissue factor or thromboplastin) and factor VII (proconvertin).
FIGURE 34-6 Schematic of the coagulation cascade (extrinsic, intrinsic, and common pathways). a, Active form.
Thrombin also behaves as an anticoagulant. It (1) prevents runaway clot formation by releasing tissue plasminogen activator (tPA) from endothelial cells, (2) stimulates protein C and protein S to inhibit clot formation, and (3) forms a relationship with antithrombin III to interfere with coagulation.1 The common pathway is the terminal pathway of the coagulation cascade. In the common pathway, factor X has been activated by the intrinsic and extrinsic pathways. Factor X requires the help of factor V (proaccelerin) and calcium to convert factor II (prothrombin) to its active-state thrombin (IIa). Thrombin then activates factor I (fibrinogen) to its active form, Ia (fibrin). Factor XIII (fibrin-stabilizing factor) is required to ensure the platelet plug will hold. Factor XIII helps form a cross-linked mesh within the platelet plug, increasing its strength. Fibrin (factor Ia) in conjunction with factor XIII finally secures a stable secondary plug, and bleeding stops. Once a clot is made, it retracts, eliminating its serum. As it retracts, it weaves the edges of the vessel together, healing the site of injury.2
Cell-Based Theory of Coagulation
The cell-based theory is a newer concept for explaining the involvement of the platelet and clotting cascades in hemostasis. It hypothesizes why platelets and the extrinsic and intrinsic pathways of coagulation cascade do not work independently of one another but form a very interdependent relationship.6 The theory posits that coagulation takes place on different “cell surfaces” that bear tissue factor (TF). These surfaces play a pivotal role in factor expression leading to hemostasis. The cell-based theory describes hemostasis as taking place in three phases: initiation, amplification, and propagation.
The initiation phase is triggered by injury to the endothelial surface (Figure 34-7). When injury occurs, TF is exposed at the site of injury. In its presence, the endothelial surface of the blood vessel changes, becoming acidic and making its phospholipid surface less repellent to platelets. TF down-regulates anticoagulants that reside in the subendothelial layer (e.g., antithrombin III, thrombomodulin) in an effort to promote coagulation.7 This new medium enhances the many enzymatic processes that work to maintain hemostasis by encouraging aggregation and the activation of clotting factors to the site of injury. TF recruits platelets and activates factor VII.
In the cell-based theory, TF/VII reaction results in the activation of factors X (common pathway) and IX (intrinsic pathway). Factor X forms a complex with factor V, and together these two activated factors are able to generate a small amount of thrombin for clot formation.8 Only a small amount of thrombin is created because this reaction terminates almost immediately when tissue factor pathway inhibitor (TFPI) limits the amount of TF expressed. The activation of factor IX from the TF/VII complex does not participate in this initiation stage because IX does not act on TF-bearing cell surfaces.7
As injury perpetuates and TF is expressed, platelets mobilize to the site of injury.7 It is during the amplification phase that thrombin generation gains momentum, and acceleration and activation of clotting factors persists. Thrombin activates factors V, VIII, and IX.6 Activated factor XI assists in generating even more factor IX on the platelet surface.9 Von Willebrand factor promotes platelet aggregation through its adhesive properties with GpIb, and the expression of the GpIIb-IIIa pseudopods from the surface of platelets facilitates aggregation of additional platelets.
The cell-based theory is a means of providing a more thorough understanding and an innovative interpretation of coagulation. It explains how cell surfaces do not just express coagulation factors; these surfaces participate in conjunction with platelets and the coagulation cascade pathways to maintain hemostasis. This theory also explains why certain deficiencies fail to cause bleeding, despite changes in laboratory values such as the PT prothrombin time (PT) or activated partial thromboplastin time (aPTT), indicative of coagulation problems.6
Fibrinloytic System
Once a disrupted vessel is sealed, there is no longer a need for a hemostatic plug. A counterbalance mechanism, the fibrinolytic system, exists to degrade fibrin. Initially there is an increase in blood flow at the site of injury. This increase in blood flow washes away ADP and thromboxane A2 and other procoagulant mediators, which were initially present to encourage hemostasis and limit the size of the clot. Thrombin, which initially behaved as a procoagulant, now acts as an anticoagulant and activates additional anticoagulant mediators. TFPI stops the action of TF. Protein C and protein S inhibit coagulation factors III, V, and VIII. Antithrombin III inhibits thrombin activity by sequestering factors XII, XI, IX, and X. Antithrombin III is a mediator that corrals some of the factors present in the clotting cascade and takes them out of the clotting equation (Figure 34-8). The clot manufactured is disrupted.
The process of fibrinolysis is highly regulated by plasma proteins (Figure 34-9). A clot is composed primarily of plasminogen, plasmin, fibrin, and fibrin degradation products. Plasminogen is an enzyme synthesized in the liver. It is stored like the clotting factors in an inactive form. While the clot is forming, plasminogen incorporates itself into the clot. With the assistance of the body’s own tPA and urokinase, plasminogen is activated to plasmin. Plasmin then acts on the fibrin, causing fibrin to degrade into fibrin degradation products. The circulatory system removes the waste products of the clot. α-Antiplasmin and tissue plasminogen activator inhibitor are important fibrinolytic mediators that stop the process of fibrinolysis when the clot has been digested.
Anesthetic Implications
The preoperative interview is the ideal time for the anesthesia provider to gather detailed information regarding the patient’s health status. A thorough history and physical is the best way to identify patients at risk for surgical bleeding or those patients with thrombopoietic tendencies.10 It is also during the interview that additional laboratory tests can be ordered if necessary to identify potential defects in hemostasis and to guide the decision regarding whether to order and/or administer blood products.
During the preoperative interview, it is important to ask questions directly related to bleeding: (1) Does the patient experience unusual bleeding or bruising (e.g., bleeding gums, epistaxis, mucous membrane bleeding, bloody stools)? (2) Is there a history of previous bleeding with dental procedures? (3) Are there repeated spontaneous bleeding episodes or a history of excess bleeding that may have occurred after a minor procedure or childhood trauma? (4) Do familial bleeding tendencies exist? (5) Has there been a time when expected bleeding from a surgical procedure was more than anticipated?4,11 These questions can reveal an undiagnosed inherited disorder of coagulation. Patients who have undiagnosed inherited coagulopathies may complain of hematomas, runaway bruising, and oozing, even after the most minor injuries. An undetected preoperative bleeding tendency can lead to life-threatening blood loss during surgery.4 Laboratory evaluation of platelets, coagulation, and fibrinolytic components can be screened with the commonly available coagulation tests.
The preoperative use of many medications—prescribed, over–the-counter, and herbal remedies—can interfere with normal platelet function and coagulation (Box 34-1). The anesthesia provider must ascertain whether the patient regularly ingests medications that might interfere with normal coagulation and when were the medications or herbals last taken. For example, many patients take aspirin for a number of reasons. Historically it has been recommended that aspirin be held for 7 to 10 days prior to surgery. Current recommendations are discussed in detail later in this chapter. Aspirin directly affects the life of the platelet by irreversibly inhibiting cyclooxygenase, resulting in decreased platelet function.12 Nonsteroidal antiinflammatory drugs (NSAIDs) also inhibit cyclooxygenase, albeit reversibly, and the recommendation is to withhold NSAIDs for approximately 24 to 48 hours to avoid any bleeding effects in surgery13,14 (Figure 34-10).
Most anesthesia providers are familiar with the Jehovah Witness (JW’s) population and their refusal of blood transfusion or derived components. However, it is important to consider that some JW’s will accept certain fractions of primary blood components (e.g., albumin, leukocyte-depleted red cells, platelets, solvent-treated fresh frozen plasma [FFP], and recombinant products) or alternatives to allogenic transfusions, as long as blood remains continuous with the body.15 Advice regarding available management options should be discussed, especially with high-risk blood-loss procedures.16
Laboratory Tests
Routine laboratory tests must be evaluated preoperatively. They serve to guide the clinician in determining whether a coagulation disorder exists (Table 34-3). Laboratory tests should be ordered on an individual basis, considering the patient’s history and planned surgical procedure.
The most frequently assessed tests are the bleeding time, platelet count, PT, and aPTT (Table 34-4). Together, these tests evaluate vascular contraction, platelet function, coagulation, and the fibrinolytic system. Results of these routine tests must fall within the normal range. If they are outside normal range, there must be a reasonable explanation, and adequate measures must be taken to correct or control hemostasis before bringing the patient into the operating room. For example, if the patient requires surgery but not emergently, vitamin K can be administered 4 to 6 hours prior to surgery. If the risk of bleeding is moderate, a type and crossmatch may be preferred to a type and screen. If the patient requires surgery emergently, ordering blood components such as packed red blood cells, FFP, platelets, and cryoprecipitate may be advisable.
TABLE 34-4
DIC, Disseminated intravascular coagulation; NSAIDs, nonsteroidal antiinflammatory drugs.
The bleeding time was once thought to be the best indicator of bleeding risk. The use of a bleeding time test, however, is open to much scrutiny, and there are many reasons to question its use and interpretation.17 In the absence of drug ingestion, a prolonged bleeding time suggests primary hemostasis abnormality, and further investigation is recommended. Although the bleeding time is a means of evaluating vascular integrity and platelet function, it is important to appreciate that a prolonged bleeding time is not a good predictor of bleeding or a sign that an abnormality is present.18 In addition, an isolated prolonged value is not a reason to cancel or delay a surgical procedure.

Full access? Get Clinical Tree
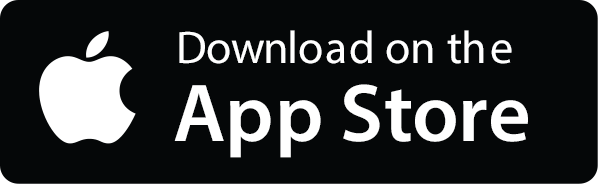
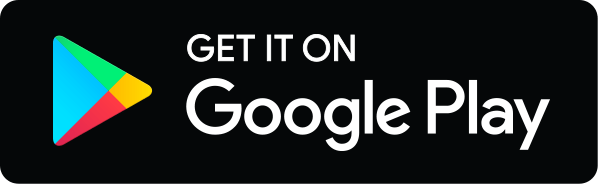