Head Injury
Chris C. Lee
Susan A. Ironstone
Marie A. Theard
A 52-year-old woman with an unknown past medical history was involved in a highspeed motorcycle collision and was airlifted to the trauma center. On the scene, she was combative, unresponsive, and intubated. At the hospital, she was found to have an open tibial/fibular fracture that was reduced and fixed with regaining of pulses after an initial pulseless left leg, a left small pneumothorax, rib fractures, and a skull fracture extending to the left parietal bone, occipital bone, clivus sphenoid sinus, and temporal bone. On admission, the patient had a Glasgow Coma Scale (GCS) of 7T, and her vital signs were a blood pressure of 69/40 mm Hg, heart rate 150 beats per minute, respiratory rate 20 breaths per minute, and an oxygen saturation 100% on 60% FIO2. The patient was resuscitated by transfusing packed red blood cells, infusing 0.9% normal saline (NS), and a norepinephrine infusion. Fresh frozen plasma (FFP) and prothrombin complex concentrate were given for correction of her coagulopathy. Her head computed tomography (CT) scans showed bilateral traumatic subarachnoid hemorrhages and subdural hematomas (Fig. 20.1). The patient was GCS 7T; therefore, a right frontal external ventriculostomy drain (EVD) was emergently placed for intracranial pressure (ICP) monitoring and cerebral spinal fluid (CSF) drainage, given that her CT scan showed traumatic subarachnoid hemorrhage and early signs of cerebral swelling. The patient was managed conservatively for her ICPs but required increasing medical treatments, including maximal hyperosmolar therapy, adequate pain control, sedation, and paralysis. Her ICP readings spiked into the 30 mm Hg after initially low ICP readings. The patient was taken to the operating room for a bifrontal craniectomy for emergency decompression secondary to severe bifrontal contusions with malignant cerebral edema (Fig. 20.2).
A. Pathophysiology and Differential Diagnosis
What types of intracranial injuries are most likely to have occurred in this patient?
What is the difference between primary and secondary injury? What factors contribute to secondary injury?
What are the benefits and risks of administering mannitol?
What is the effect of hyperglycemia on neurologic outcome following head trauma?
What alterations in sodium and potassium balance can occur in patients with head injury?
In addition to sodium and potassium, what other electrolyte abnormalities can be present after head trauma?
What are the neuroprotective effects of administering magnesium (Mg)?
What roles do gender and female sex hormones have in the pathophysiology of traumatic brain injury (TBI)?
What is the role of decompressive craniectomy (DC) as a treatment option for ICP control after head injury?
Do genetic factors play a role in the outcome after head injury?
B. Preoperative Evaluation and Preparation
What is the GCS? What is the significance of a GCS of 7T in this patient?
In addition to the GCS, what other assessments can be done to evaluate neurologic function?
What is the role of CT scanning in the initial evaluation of the patient with head injury? What management options can be done in patients whose neurologic condition is deteriorating before obtaining a CT scan?
What is the role of ICP monitoring in the management of head injury?
How can you clear this patient’s cervical spine?
What are the advantages of early endotracheal intubation in this patient?
What is your plan for airway management in this patient? How would it change if the patient were combative? How would it change if the patient had facial fractures with significant swelling of the head and neck?
What are the effects of succinylcholine on ICP? What is the significance of the effect, if any?
What coagulation abnormalities are present after TBI? What modalities can be used to provide hemostasis and reduce intracranial hematoma expansion?
C. Intraoperative Management
What is appropriate hemodynamic monitoring during CT scanning and during craniotomy for evacuation of a subdural hematoma?
Should this patient be hyperventilated?
What are the implications of arterial hypertension in patients with head injury? How should blood pressure be managed?
What should be done about intravenous (IV) fluid replacement? Should corticosteroids be given empirically?
Should hypertonic saline (HTS) be administered to this patient? How is HTS administered?
The patient underwent a craniectomy for evacuation of a frontal intracerebral hematoma. Which anesthetic agents should be avoided and what agents might be preferred in this situation?
Should hypothermia be employed in this patient?
D. Postoperative Management
What are the postoperative ventilation concerns in this patient?
What specific measures should be used to control the patient’s ICP? What type of monitoring devices can be used to measure ICP?
What is neurogenic pulmonary edema? Would you avoid positive end-expiratory pressure (PEEP) in a patient with increased ICP?
What is the role of antiseizure prophylaxis in the perioperative management of head trauma?
How can cerebral oxygenation monitoring be used in the clinical management of TBI?
What methods can be used in the neurointensive care unit to prevent hyperthermia?
A. Pathophysiology and Differential Diagnosis
A.1. What types of intracranial injuries are most likely to have occurred in this patient?
The common causes of TBI include falls, motor vehicle accidents, assaults, and gunshot wounds. TBI is a leading cause of death and disability after injury in the United States of America. The following types of intracranial injuries are most likely to have occurred in this patient:
Concussion is the most common type of TBI and the least apparent on imaging. It is often completely invisible to any type of imaging and must be assessed and diagnosed clinically. It is also the most common type of TBI to result in ongoing morbidity, such as decreased job or school performance, and produces lasting cognitive symptoms. This is a very complex type of injury and is a big focus of professional sports team injury experts. Currently, there is a social movement to raise awareness of these injuries, particularly in young athletes.
Diffuse axonal injury (DAI) is difficult to see on CT of the brain (although small punctate hemorrhages in white matter tracts can be seen). Magnetic resonance imaging (MRI), particularly susceptibility weighted imaging, is a more sensitive way to detect it. DAI can produce severe neurologic impairment with a poor prognosis for improvement.
Traumatic subarachnoid hemorrhage (tSAH) is the most common type of posttraumatic intracranial hemorrhage and the least severe indicator of injury. Although this finding often signals the presence of a significant concussion injury, it does not require surgical intervention. tSAH resolves within days and carries far lower risk of the secondary consequences associated with spontaneous aneurysmal subarachnoid hemorrhage (i.e., hydrocephalus and stroke from intracerebral vasospasm). Follow-up CT scans are usually not necessary due to this low risk of secondary neurologic injury.
Epidural hematoma (located between the skull and dura mater) is classically associated with displaced temporal bone skull fractures that cause a laceration of the middle meningeal artery. This arterial hemorrhage results in a rapidly expanding hematoma lateral to the temporal lobe which can lead to death from herniation if not emergently surgically evacuated. Injury to large venous structures including the dural sinuses or venous bleeding from fractured bone can lead to a so-called venous epidural hematoma. Because these hemorrhages occur at venous pressure, they are thought to be less likely to expand to a dangerous volume, although sometimes surgical treatment is required. Patients with an acute epidural hematoma often exhibit a lucid interval after the initial impact before deteriorating. The signs of this deterioration can include depressed mental status, an ipsilateral fixed and dilated pupil, contralateral hemiplegia, and imaging demonstrating uncal herniation due to a rapidly expanding hematoma. Arterial epidural hematomas are almost always a true neurosurgical emergency; rapid intervention makes the difference between death and a good prognosis.
Subdural hematomas (located between the brain and dura) arise from a tear in the dural sinuses or bridging veins and may accumulate more insidiously than an epidural hematoma. They are classified as acute (up to 48 hours), subacute (48 hours to 12 days), and chronic (more than 12 days) according to the interval of time between the TBI and the onset of symptoms. Subdural hematomas greater than 1 cm in thickness or causing more than 1 cm of midline shift are generally associated with significant lateralizing neurologic symptoms and often constitute a surgical emergency. Small subdural hematomas can expand in volume as they grow more chronic and can produce delayed onset of significant neurologic signs and symptoms.
Intracerebral hematomas and hemorrhages arise from the tearing of small vessels in the white matter and are due to penetrating head injury or caused by accelerationdeceleration injuries that involve laceration of cortical arteries. These hemorrhages and hematomas can often be managed medically with serial follow-up head CT scans. Surgical evacuation of intracerebral hematomas is rarely indicated due to the deep location of many of these hemorrhages. Although they can be associated with trauma, hypertension, amyloid angiopathy, and/or profound coagulopathy are more common causes.
Cerebral contusions are superficial hemorrhages that occur mainly when the anterior temporal and frontal lobes strike the rough edges of the tentorium (contrecoup contusion). In a patient without focal neurologic deficits, the most probable intracranial injury is a cerebral contusion after ruling out other focal brain injuries. This type of injury is often associated with cerebral edema and can require aggressive management with hyperosmolar therapies or surgical craniectomy for decompression.
Brambrink AM, Kirsch JR, eds. Essentials of Neurosurgical Anesthesia & Critical Care. New York: Springer; 2012:93-105.
Faul M, Xu L, Wald MM, et al. Traumatic Brain Injury in the United States: Emergency Department Visits, Hospitalizations and Deaths, 2002-2006. Atlanta, GA: U.S. Department of Health and Human Services, Centers for Disease Control and Prevention, National Center for Injury Prevention and Control; 2010.
Ghajar J. Traumatic brain injury. Lancet. 2000;356:923-929.
Jaffe RA, Schmiesing CA, Golianu B. Anesthesiologist’s Manual of Surgical Procedures. 5th ed. Philadelphia, PA: Wolters Kluwer Health; 2014:37-44.
A.2. What is the difference between primary and secondary injury? What factors contribute to secondary injury?
Primary injury refers to the direct injury of tissue at the moment of head trauma.
Secondary injury occurs minutes, hours, and even days after the initial head trauma and is the consequence of ischemic insults to the brain. The factors contributing to secondary injury include systemic causes (i.e., hypoxia, hypercapnia, and hypotension) and intracranial causes (i.e., intracranial hypertension, or elevated ICP, and herniation). Systemic hypotension and intracranial hypertension are factors related to increased morbidity and mortality in patients with severe TBI. Mental status changes suggest inadequate cerebral perfusion, either from decreased systemic pressure or elevated ICP. Cerebral perfusion pressure equals mean arterial pressure minus either ICP or central venous pressure, whichever is greater (CPP = MAP – ICP or CVP). Therefore, it is critical to maintain adequate oxygenation, cerebral perfusion, and a normal PaCO2 to prevent or decrease the chance of mortality or any deleterious sequelae caused by secondary injury. A general goal is to maintain CPPs greater than 60 mm Hg to ensure adequate brain perfusion and avoid secondary injury.
Algattas H, Huang JH. Traumatic brain injury pathophysiology and treatments: early, intermediate, and late phases post-injury. Int J Mol Sci. 2013;15:309-341.
Miller RD, Cohen NH, Eriksson LI, et al, eds. Miller’s Anesthesia. 8th ed. Philadelphia, PA: Elsevier Saunders; 2015:2158-2198.
A.3. What are the benefits and risks of administering mannitol?
Benefits
Mannitol (20%) 0.25 to 1.0 mg per kg is effective in reducing ICP by increasing serum osmolarity to 300 to 315 mOsm per L to improve CPP, which facilitates surgical exposure and reduces tissue trauma from surgical retraction. The use of hyperosmolar agents is theoretically limited by an upper acceptable osmolarity limit of about 320 mOsm per L. Its osmotic diuretic effect begins in about 5 to 10 minutes and achieves a peak effect in about 30 minutes. Mannitol has been commonly accepted as an acute treatment for cerebral edema for decades.
Risks
After TBI, the possibility of loss of the blood-brain barrier (BBB) integrity leading to leak of mannitol into cerebral interstitial tissue may actually worsen cerebral edema.
Osmotic diuresis can cause hypovolemia and consequent hypotension aggravating cerebral ischemia.
It can transiently cause hypervolemia and result in congestive heart failure in those patients who have borderline cardiac function.
Electrolyte abnormalities (e.g., hypernatremia) due to diuresis can occur.
A serum osmolarity above 320 mOsm per L can cause renal failure.
It could cause rebleeding in patients with expanding hematoma by removing the tamponading effect of the hematoma if mannitol is administered before the dura is opened.
Colton K, Yang S, Hu PF, et al. Responsiveness to therapy for increased intracranial pressure in traumatic brain injury is associated with neurological outcome. Injury. 2014;45(12):2084-2088.
Miller RD, Cohen NH, Eriksson LI, et al, eds. Miller’s Anesthesia. 8th ed. Philadelphia, PA: Elsevier Saunders; 2015:2158-2198.
A.4. What is the effect of hyperglycemia on neurologic outcome following head trauma?
Hyperglycemia resulting from the nervous systems response to injury following moderate to severe head trauma has been associated with increased odds ratio for unfavorable neurologic outcome and increased risk for mortality. Additionally, persistent hyperglycemia has been found to be an independent predictor of an unfavorable outcome in patients with severe TBI.
Nevertheless, tight glucose control has been related to elevations in biomarkers for cerebral cellular metabolic distress and to reduced survival at 21 days. This may be a result
of both adverse effects of episodes of severe hypoglycemia as well as increased metabolic requirement of the injured brain.
of both adverse effects of episodes of severe hypoglycemia as well as increased metabolic requirement of the injured brain.
Currently, moderate glucose control, with a range of 140 to 180 mg per dL is recommended. Glucose levels of less than 100 should be avoided, and hypoglycemia should be treated immediately.
Miller RD, Cohen NH, Eriksson LI, et al, eds. Miller’s Anesthesia. 8th ed. Philadelphia, PA: Elsevier Saunders; 2015:3103.
Muehlschlegel S, Carandang R, Ouillette C, et al. Frequency and impact of intensive care unit complications on moderate-severe traumatic brain injury: early results of the Outcome Prognostication in Traumatic Brain Injury (OPTIMISM) Study. Neurocrit Care. 2013;18:318-331.
A.5. What alterations in sodium and potassium balance can occur in patients with head injury?
Electrolyte abnormalities such as hyponatremia and hypokalemia are commonly seen following head trauma. Hyponatremia may result via several mechanisms, including hypothalamus-pituitary dysfunction, cerebral salt wasting syndrome, and syndrome of inappropriate antidiuretic hormone. Additionally, sodium loss may be exacerbated by medications used to treat cerebral edema. Severe hypokalemia has been found to be an independent mortality risk factor in patients with TBI and is likely related to trauma and stress-related catecholamine surge. Hypernatremia, resulting from mannitol use, HTS, or diabetes insipidus, is also frequently seen in association with severe TBI.
Wu X, Lu X, Lu X, et al. Prevalence of severe hypokalaemia in patients with traumatic brain injury. Injury. 2015;46(1):35-41.
Zhang W, Li S, Visocchi M, et al. Clinical analysis of hyponatremia in acute craniocerebral injury. J Emerg Med. 2010;39:151-157.
A.6. In addition to sodium and potassium, what other electrolyte abnormalities can be present after head trauma?
Multiple electrolyte abnormalities, including hypomagnesemia and hypophosphatemia, are often associated with TBI and can be challenging to treat.
Symptoms of hypomagnesemia include ventricular arrhythmias, widened QRS, prolonged PR interval, weakness, and seizures. Symptomatic hypomagnesemia, or Mg level of less than 0.8 mEq per L, should be treated with IV Mg, although oral Mg should be used for asymptomatic patients because most of the IV Mg dose is likely to be renally excreted. Mg repletion may also be required for successful correction of hypokalemia or hypocalcemia.
Hypophosphatemia can present with hypotension, seizures, as well as cardiac or respiratory failure. Treatment of hypophosphatemia can result in severe hypocalcemia. Phosphate replenishment should be considered only for symptomatic patients.
Miller RD, Cohen NH, Eriksson LI, et al, eds. Miller’s Anesthesia. 8th ed. Philadelphia, PA: Elsevier Saunders; 2015:1801-1802.
Wu X, Lu X, Lu X, et al. Prevalence of severe hypokalaemia in patients with traumatic brain injury. Injury. 2015;46(1):35-41.
A.7. What are the neuroprotective effects of administering magnesium (Mg)?
N-methyl-D-aspartate (NMDA) receptors are stimulated by excitatory amino acids in the central nervous system, the most abundant of which is glutamate. Once stimulated, the NMDA receptor ion channels (blocked by Mg2+ which is relieved by postsynaptic membrane depolarization) will facilitate calcium entry into the cell. In the face of ischemia (TBI), excess glutamate causing excitotoxicity leads to increased intracellular calcium and cell death. Mg, a noncompetitive inhibitor of the NMDA receptor ion channel, will limit the influx of calcium, thereby decreasing cell death. Other effects of Mg include a downregulation of the membrane protein, aquaporin 4, which is known to increase cerebral edema and a decrease in upregulation of the tumor suppressor gene TP53 which causes apoptosis. The benefits of Mg have been translated into decreased cortical damage noted in the rat brain after brain injury. Furthermore, ionized Mg is decreased in humans after TBI.
Despite encouraging preclinical data of the benefits of Mg as well as established safety studies, human outcome studies on the impact of Mg after TBI are varied. Mg administered 12 hours after TBI led to a significant decrease in mortality at 3 months: 13% mortality for patients treated with Mg versus 47% in the placebo group. Of those who survived, good recovery (Glasgow outcome score) was observed in 54% of the patients treated with Mg and 38% of patients treated with placebo. In another study, however, Mg administered to patients within 8 hours of brain injury had no beneficial effect based on mortality, seizures, functional status, or neuropsychological testing at 6 months postinjury. Study design problems, such as missing baseline vital signs, glucose values, concurrent injuries, and neurologic scores at discharge, contributed to the mixed results. Another challenge of translating the benefit of Mg seen in preclinical studies to human research is the notably small, possibly inadequate, increase in ionized Mg in CSF affected by increasing serum Mg levels. This finding may relate to the integrity of the BBB. Finally, the multifactorial etiology of TBI may necessitate research examining combination therapy (e.g., combinations of hypothermia, hyperoxia which restores mitochondrial function, and/or Mg administration) in order to achieve more consistent and favorable outcomes.
Dhandapani SS, Gupta A, Vivekanandhan S, et al. Randomized control trial of magnesium sulphate in severe closed traumatic brain injury. Indian J Neurotrauma. 2008;5(1):27-33.
McKee JA, Brewer RP, Macy GE, et al. Analysis of the brain bioavailability of peripherally administered magnesium sulfate: a study in humans with acute brain injury undergoing prolonged induced hypermagnesemia. Crit Care Med. 2005;33:661-666.
Sen AP, Gulati A. Use of magnesium in traumatic brain injury. Neurotherapeutics. 2010;7(1):91-99.
Temkin NR, Anderson GD, Winn HR, et al. Magnesium sulfate for neuroprotection after traumatic brain injury: a randomised controlled trial. Lancet Neurol. 2007;6:29-38.
A.8. What roles do gender and female sex hormones have in the pathophysiology of traumatic brain injury (TBI)?
Approximately 52,000 people in the United States die from TBI per year. Gender is an important consideration in understanding how to improve outcome in TBI as the death rate from this disease is significantly reduced in women. This together with preclinical data suggesting less severe sequelae from TBI in younger women has served as the impetus for exploring how sex hormones may reduce mortality and improve outcome after TBI. Attella et al. demonstrated improved functional outcome in pseudopregnant rats (higher progesterone levels) after TBI compared to normal cycling female rats with similar injuries. Other mechanisms by which progesterone may improve outcome after TBI include an anti-inflammatory action by reducing microglia activation and proinflammatory cytokines and a postinjury remyelination and repair. Estrogen may also be beneficial in cerebral ischemia by stabilizing the BBB and increasing blood flow during and after ischemia as well as improving regeneration and plasticity of new neurons.
Small clinical studies that demonstrated a more favorable outcome with administration of progesterone 8 hours after injury while encouraging did not translate into positive findings in two recently published major progesterone trials. In these two separate randomized controlled trials (SYNAPSE and PROTECT III trial), progesterone administered to TBI patients did not produce a favorable outcome at 6 months as assessed by Glasgow Outcome Score-Extended (includes an assessment of ability to participate in activities of daily living) or other secondary outcomes. At this time, the role of progesterone in TBI is unclear.
Attella MJ, Nattinville A, Stein DG. Hormonal state affects recovery from frontal cortex lesions in adult female rats. Behav Neural Biol. 1987;48:352-367.
Coronado VG, Xu L, Basavaraju SV, et al. Surveillance for traumatic brain injury-related deaths—United States, 1997-2007. MMWR Surveill Summ. 2011;60(5):1-32.
Herson PS, Koerner IP, Hurn PD. Sex, sex steroids, and brain injury. Semin Reprod Med. 2009;27(3):229-239.
Skolnick BE, Maas AI, Narayan RK, et al. A clinical trial of progesterone for severe traumatic brain injury. N Engl J Med. 2014;371(26):2467-2476.
Wright DW, Yeatts SD, Silbergleit R, et al. Very early administration of progesterone for acute traumatic brain injury. N Engl J Med. 2014;371(26):2457-2466.

Full access? Get Clinical Tree
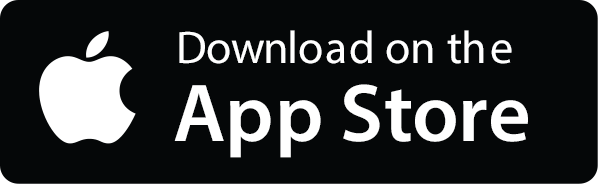
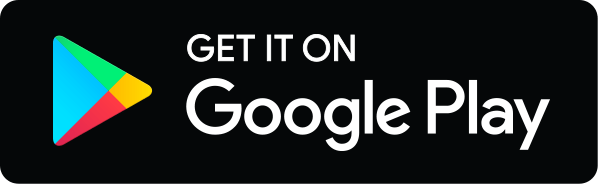
