Genetic Preconception and Prenatal Counseling
Wendy C. McKinnon MS
Elizabeth Dorsey Smith EdD, RN
William T. Seed MD, FAAP
PART 1 Genetic Counseling
Wendy C. McKinnon MS
INTRODUCTION
The financial and societal costs resulting from genetic conditions are significant. Last estimates of the incidence of genetic disease in newborns were between 5% and 6% (Baird, Anderson, Newcombe, & Lowry, 1988). Although current estimates are unavailable, past studies show that 52% of pediatric hospitalizations (Hall, Powers, McIlvaine, & Ean, 1978), 42% of pediatric deaths (Roberts, Chavez, & Court, 1970), and 11.5% of adult inpatient admissions (Emery & Rimoin, 1990) were attributable to genetic conditions. Preconception genetic risk assessment may direct screening and testing for unsuspected genetic disorders in individuals of childbearing age, helping to provide a more accurate assessment of risks for future offspring (Cohn, Miller, Gould, Macri, & Gimovsky, 1999).
Historically, the primary goal of genetic counseling was prevention of birth defects. In this era of increasing genetic information, however, expanded knowledge and enhanced reproductive choice should be the goals. The value of preconception genetic counseling must not be overlooked. With regard to congenital malformations, birth defects, and many inherited conditions, education and counseling before conception and subsequent organogenesis allow couples to make informed decisions regarding possible risks and to assess the possibilities that best fit their individual circumstances. They often will miss such opportunities if they wait until the first prenatal visit (Hogge & Hogge, 1996).
Individuals and couples will likely have different reactions to genetic information depending on the specific condition, its severity, prenatal testing options, disease treatment options, and personal experience with the condition. Reproductive options include the following:
Not bearing children
No intervention during pregnancy
Prenatal diagnosis, with the option of terminating an affected fetus
Alternative reproductive options, such as ovum or sperm donation or preimplantation diagnosis
FAMILY HISTORY
A thorough and appropriately detailed family history is an important part of genetic counseling. A three-generation pedigree significantly influences risk assessment for future offspring and reveals an individual’s risk for significant heritable medical problems. The provider should inquire about a family history of birth defects, neural tube defects, recurrent miscarriages, stillbirths, mental retardation or developmental delay, consanguinity, early deaths, and specific genetic disorders (eg, cystic fibrosis [CF], fragile X syndrome, neurofibromatosis). If a family history of an apparent heritable disorder exists, the preconception period allows time to collect medical records, document the reported history, and refer the woman or couple to a clinical geneticist or other specialist. If a specific diagnosis is made, then the parties can discuss risks of recurrence, options for possible prenatal diagnosis, and available treatment options.
The provider should ask about late-onset disorders in the family, such as Huntington’s disease, Alzheimer’s disease, hereditary breast or ovarian cancer syndrome, and hereditary nonpolyposis colon cancer syndrome. Individuals may want to consider predisposition genetic testing before starting a family to determine whether they are at risk. Genetic counseling before and after the test is extremely important for individuals interested in predisposition genetic testing. The National Society of Genetic Counselors has outlined the important elements of such counseling in its position statement (McKinnon et al., 1997).
ETHNICITY AND GENETIC CONDITIONS
Couples from certain ethnic populations may be at increased risk for having offspring with specific autosomal recessively inherited conditions. Common conditions are discussed in the following sections.
Thalassemias
The thalassemias are a heterogeneous group of genetic disorders characterized by absent or decreased production of normally functioning hemoglobins. Normal red blood cells contain a combination of three hemoglobins: hemoglobin A (Hb A), hemoglobin A2 (Hb A2), and hemoglobin F (Hb F). Each of these hemoglobins is composed of two α- and two non-α (β-, δ-, or τ-) -globin chains. Two α-globin genes are on chromosome 16, providing four copies in normal individuals; the β-, δ-, and τ-globin genes are on chromosome 11.
Normally, production of α- and non–α-chains is balanced: Hb A is α2β2, Hb A2 is α2δ2, and Hb F is α2τ2.
Normally, production of α- and non–α-chains is balanced: Hb A is α2β2, Hb A2 is α2δ2, and Hb F is α2τ2.
In both α- and β-thalassemias, a decreased number of globin chains results in microcytosis and ineffective erythropoiesis and hemolysis. Varying degrees of microcytic hypochromic anemia ensue (Dumars et al., 1996). If Hb A2, Hb F, or both are increased, then the diagnosis is β-thalassemia. If Hb A2 and Hb F are normal and ferritin is normal or high, then α-thalassemia is the likely diagnosis. Providers must be aware that unusual structural variants also can occur (Dumars et al., 1996).
Couples of Mediterranean (eg, Southern Italy, Greece) descent are at increased risk for having a child with β-thalassemia (Olivieri, 1999). Couples of Southeast Asian (eg, Laos, Thai, Cambodia, Hmong) descent are at increased risk for having a child with α-thalassemia. Couples of East Indian and Middle Eastern ancestry are at increased risk for having a child with either α- or β-thalassemia or sickle cell disease (discussed below). A simple first-line screen for both types of thalassemia is a complete blood count with red blood cell indices.
• Clinical Pearl
Carriers of thalassemia have a mild anemia and a low mean corpuscular volume (MCV). An MCV less than 80 should prompt further evaluation. Providers should offer individuals with a low MCV hemoglobin electrophoresis for quantitative Hb A2 and Hb F. Measurement of ferritin in such individuals also is important to rule out iron-deficiency anemia.
Sickle Cell Anemia
Sickle cell anemia (SCA) is a hemoglobinopathy in which an inherited structural abnormality occurs in one of the globin chains. A change of one amino acid from glutamic to valine in the hemoglobin molecule alters the molecule’s configuration, causing the cells to sickle and obstruct blood flow in small vessels. Episodes of vaso-oclusive crisis and chronic anemia characterize SCA because the red blood cells tend to become deformed under conditions of decreased oxygen tension.
• Clinical Pearl
People of African American ancestry are at increased risk for having a child with SCA. The carrier state (sickle cell trait) is found in approximately 1 out of every 10 African Americans, and incidence of SCA in this population is 1 in 400. To identify carriers of sickle cell trait, providers should obtain a hemoglobin electrophoresis.
Cystic Fibrosis
Cystic fibrosis is an autosomal recessive, chronic illness characterized primarily by pulmonary insufficiency and pancreatic dysfunction. One of every 25 individuals of northern European ancestry is a carrier for CF, resulting in 1 in 2500 affected births in this population. CF results from an abnormality in the CF transmembrane receptor gene. Normally, this gene produces a protein that is crucial to the proper transport of salt and chloride across cell membranes. When this transport is disrupted, the body produces excessive amounts of thick mucus, which leads to breathing problems and lung infections. Diarrhea and poor digestion also occur. Individuals with CF have an increased risk for infertility. Male infertility primarily results from congenital bilateral absence of the vas deferens (CBAVD). Up to 82% of males with isolated CBAVD have been found to have an identifiable CF mutation in one or both chromosomes (de Braekeleer & Ferec, 1996).
Severity of CF varies greatly. Some affected individuals manifest symptoms at birth, others later in childhood, and still others in young adulthood. Carrier screening for CF involves DNA mutation analysis. The detection rate is approximately 90% among individuals of northern European ancestry and 97% among Ashkenazi Jews, but it is lower among individuals of other ethnic backgrounds. This detection rate varies depending on the number of mutations that a laboratory screens. Therefore, even with a negative carrier screen, a chance still exists that the individual is a carrier.
In April 1997, the National Institutes of Health (NIH) convened a consensus conference regarding carrier genetic testing for CF. The panel concluded that the following people should be offered testing:
Adults with a positive family history of CF
Partners of individuals with CF
Couples planning a pregnancy
Couples during early pregnancy as a screening test for CF in the fetus
The American College of Medical Genetics (ACMG), however, disagrees with the NIH’s recommendations to offer CF genetic testing to all couples who are planning a pregnancy or are pregnant. They believe this recommendation to be premature until two requirements are readily available:
Adequate educational, genetic counseling, and other support systems
Adequate experience with the sensitivity and specificity of the testing for CF mutations in the ethnic and racial groups being served
The ACMG emphasizes that widespread genetic testing for CF is not simple and should be offered only by a provider who is familiar with the tests and their interpretation (www.faseb.org/genetics/acmg/pol-32.htm, 1997).
Lysosomal Storage Diseases
Couples of Ashkenazi Jewish ancestry are at increased risk for having offspring with autosomal recessive lysosomal storage diseases, such as Tay Sachs disease (TSD), Canavan’s disease (CD), Gaucher’s disease (GD), and Niemann-Pick disease (NPD). In addition, approximately 1 in 40 individuals of Ashkenazi Jewish ancestry carries an abnormality in either the BRCA1 or BRCA2 gene. These mutations are associated with autosomal dominantly inherited breast and ovarian cancers (Tonin et al., 1996). Several laboratories offer carrier testing, often as a panel, for these diseases.
Tay-Sachs Disease
Tay-Sachs disease is a progressive, neurologic disease that begins in infancy. Among Ashkenazi Jews, approximately 1 in every 30 individuals is a carrier for TSD, and the incidence of TSD in this population is 1 in 3600. TSD results from a deficiency of the enzyme hexosaminidase A (Hex A), which is crucial to the normal functioning of the central nervous system (CNS). Deficiencies of Hex A cause fatty substances to accumulate in the nerve cells and other organs. Typically, a baby with TSD develops normally for several months, but a progressive deterioration then begins, causing blindness, deafness, seizures, and paralysis. Children with TSD usually die by age 5 years, but some survive longer. Unfortunately, no treatment is available for TSD. Carrier screening is done through an enzyme assay that
measures Hex A activity in the blood; however, this test is not reliable in pregnant women. Hex A in the leukocytes or platelets should be measured in pregnant women. TSD carrier testing also can be done with DNA mutation analysis; however, this technology has limitations. Therefore, the most reliable method for routine TSD carrier testing is Hex A level enzyme analysis, rather than DNA testing (Natowicz & Prence, 1996).
measures Hex A activity in the blood; however, this test is not reliable in pregnant women. Hex A in the leukocytes or platelets should be measured in pregnant women. TSD carrier testing also can be done with DNA mutation analysis; however, this technology has limitations. Therefore, the most reliable method for routine TSD carrier testing is Hex A level enzyme analysis, rather than DNA testing (Natowicz & Prence, 1996).
Couples of French Canadian ancestry, specifically from the Bas-St. Laurent and Gaspesie regions of Quebec, appear to be at increased risk for having a child with TSD, owing to a founder effect. Population studies have suggested that individuals of French Canadian ancestry living in southern New England (eg, Connecticut, Rhode Island, Massachusetts) are likely to have migrated from northern Quebec. The carrier frequencies for TSD among such individuals appear to be similar to those of the Ashkenazi Jewish population, suggesting that carrier testing is appropriate in this region (Prence, Jerome, Triggs-Raine, & Natowicz, 1997). Individuals of French Canadian ancestry living in northern New England (eg, Vermont, New Hampshire, Maine) are less likely to be from northern Quebec, and an increased carrier frequency for TSD has not been found in this population. Thus, routine carrier testing is not indicated for this region (Palomaki, Williams, Haddow, & Natowicz, 1995).
Canavan’s Disease
Canavan’s disease is a progressive, neurologic disease that usually results in death before age 10 years, although some children with CD survive until their teens or early 20s. Three variants of CD exist: congenital, infantile, and juvenile. The infantile form is the most common, with onset beginning at age 3 to 6 months. One in 40 individuals of Ashkenazi Jewish ancestry is a carrier for CD, and the incidence of CD in this population is 1 in 6400.
A deficiency of the enzyme aspartoacylase, which is crucial to normal functioning of the CNS, results in CD. Typically, babies with CD appear normal at birth, but a progressive deterioration begins after several months, resulting in mental retardation and hypotonia, which progresses to spasticity, blindness, seizures, and feeding problems. No effective treatment is available. Carrier testing for CD involves DNA mutation analysis. The detection rate is 98% in Ashkenazi Jews but is lower in individuals of partly Jewish or non-Jewish ancestry (Matalon, 1997).
Gaucher’s Disease
Gaucher disease is a condition that occurs in three clinically distinct forms, classified by age of onset, severity, and presence of neurologic involvement. Type I, the most common and mildest form of GD, usually has onset in adulthood and does not have neurologic manifestations. One of 12 Ashkenazi Jews is a carrier for type 1 GD, which translates to a 1 in 576 incidence in this population. Types 2 and 3 are much rarer, have earlier onset, and include neurologic involvement. They occur in all ethnic groups, and incidences are not increased among Jews.
All three types of GD result from deficiency of the enzyme glucocerebrosidase, which is necessary for the breakdown of glucocerebroside. In type 1 GD, glucocerebroside accumulates in the bone marrow, spleen, and liver, causing these organs to enlarge, with subsequent bone and joint pain, anemia, and impaired blood clotting. Type 1 is treatable through enzyme replacement. DNA testing for type 1 detects 95% of carriers in the Jewish population. Providers must remember that type 1 GD can be mild with late onset, and carrier screening may identify affected individuals who are asymptomatic (Grabowski, 1997).
Niemann-Pick Disease
Niemann-Pick disease consists of a group of clinically distinct inherited disorders. Type A, progressive and neurogenerative with onset in infancy, is most common among Ashkenazi Jews, with a carrier frequency of 1 in 90 and an incidence of 1 in 32,400 in this population. Type B has delayed onset, usually is not neuronopathic, and is found in all populations. Types C, D, and E also exist.
All forms of NPD result from deficiency of sphingomyelinase, which is necessary for the metabolism of sphingomyelin. In type A, accumulation of sphingomyelin in the bone marrow, CNS, and other organs leads to organ enlargement, failure to thrive, rapid neurologic deterioration, and death by age 4 years. Patients with type B may survive into adolescence or adulthood, depending on the degree of neurologic involvement. No effective treatment is available for NPD. DNA-based carrier testing in the Ashkenazi Jewish population can identify approximately 95% of carriers of type A (Schuchman & Miranda, 1997).
Hereditary Breast and Ovarian Cancer
Approximately 5% to 10% of all cases of breast and ovarian cancer are inherited, with two genes, BRCA1 and BRCA2, primarily responsible. Three specific mutations in these two genes account for most cases of hereditary breast and ovarian cancer in the Ashkenazi Jewish population. Approximately 1 in 40 individuals of Ashkenazi Jewish heritage carries one of these three mutations. When a personal or family history of breast or ovarian cancer is present, the probability that one of these three mutations also exists increases (Tonin et al., 1996).
While these three mutations account for most hereditary breast and ovarian cancer in the Jewish population, some families that clearly have inherited breast and ovarian cancer by pedigree analysis do not have one of these mutations. These families may have mutations in other parts of BRCA1 or BRCA2 or in another gene that has yet to be identified (Schubert et al., 1997). Genetic testing for inherited breast and ovarian cancer should occur only after pretest genetic counseling and informed consent, followed by post-test genetic counseling and psychosocial support (McKinnon et al., 1997).
GENETIC CONDITIONS AND PRIOR PREGNANCY HISTORY
In the following situations, the provider should refer the couple for genetic counseling to discuss recurrence of risks and options for prenatal diagnosis:
For women who have had complications in previous pregnancies, the provider should consider screening for mutations in the thrombophilia genes. One study (Kupferminc et al., 1999) identified mutations in the factor V gene (factor V Leiden), which encodes methylenetetrahydrofolate reductase, and the prothrombin gene. This study also found deficiencies in proteins S and C, antithrombin III, and anticardiolipin antibodies. Another study showed that carriers of factor V Leiden have a higher risk for fetal loss, particularly miscarriage, than noncarriers (Meinardi et al., 1999).
For couples who have had a child with a neural tube defect, the woman must increase folic acid supplementation (see later discussion).
For couples who have had a child with a chromosomal trisomy, the recurrence risk in a subsequent pregnancy is 1% or the woman’s age-related risk, whichever is higher.
For couples who have had a child with one or more congenital anomalies, the recurrence risk depends on the etiology. The couple should consult a clinical geneticist to determine the correct diagnosis.
For couples who have had multiple recurrent miscarriages, karyotyping of both partners can help rule out a translocation or other chromosome rearrangement, which is the etiology in approximately 5% of cases (Bick, Madden, Heller, & Toofanian, 1998).
For couples who have had a fetal demise or stillbirth, the provider should review the autopsy (if available) and consult a clinical geneticist for the possible diagnosis.
• Clinical Pearl
Kupferminc et al. (1999) found that women with serious obstetric complications (eg, preeclampsia, abruptio placentae, fetal growth retardation, and stillbirth) have an increased incidence of mutations, predisposing them to thrombosis.
GENETIC CONCERNS RELATED TO AGE
Providers should educate women and their partners about age-related risk factors and available tests during the preconception period. Such tests include screening (eg, triple marker screening test and ultrasound) and diagnostic tests (eg, chorionic villus sampling and amniocentesis). Please see the Appendix on Prenatal Screening.
Maternal Age
Providers should offer genetic counseling to women age 35 and older because of the increased risk for chromosomal abnormalities associated with older maternal age. Down syndrome is the most common chromosomal trisomy, but couples should realize that other chromosomal trisomies are associated with increasing maternal age, some of which are more life-threatening than Down syndrome (trisomy 13 and 18) and some less (sex chromosome abnormalities).
• Clinical Pearl
Although all women are at risk for having a child with a chromosomal anomaly, women over 35 years routinely are offered prenatal diagnosis for such anomalies. After a woman is 35, her risk for losing a pregnancy from an amniocentesis is less than the risk of identifying a chromosomal abnormality (Hect & Hook, 1996).
Paternal Age
Increased paternal age has been linked with autosomal dominant genetic diseases (eg, neurofibromatosis, achondroplasia, Apert’s syndrome, Marfan syndrome) appearing as new mutations in their offspring. Estimates are that the absolute frequency of such diseases resulting from new mutations among offspring of fathers age 40 years or older is between 0.3% and 0.5%. This risk is similar in magnitude to the risk of Down syndrome in offspring of mothers who are 35 years or older. No specific prenatal tests screen for new dominant mutations. Genetic counseling is recommended for couples in whom advanced paternal age is a concern (McIntosh, Olshan, & Baird, 1995; American College of Obstetricians and Gynecologists, 1997).
MATERNAL HEALTH
Certain medical conditions in women, including diabetes, epilepsy, and phenylketonuria (PKU), may increase the risk for congenital malformations in their offspring. Women with specific genetic conditions, such as CF, Marfan syndrome, myotonic dystrophy, neurofibromatosis, and hereditary hemorrhagic telangiectasia, will likely benefit from preconception genetic counseling.
Infants of mothers with poor glucose control during organogenesis have a twofold to threefold higher risk of birth defects than offspring of nondiabetic mothers. Good glucose control preconceptionally can reduce the risk of birth defects to a level comparable to that of the general population (Janz et al., 1995; Casele & Laifer, 1998; Herman, Janz, Becker, & Charron-Prochownik, 1999).
Women with epilepsy of unknown etiology, even with the factored risk of many anticonvulsant medications, have a twofold increased risk for congenital malformations in their offspring. Specific defects related to maternal epilepsy include cleft lip or palate, congenital heart defects, and neural tube defects.
A less commonly recognized situation is the risks posed by maternal PKU. Over the past decades, successful screening and institution of a low phenylalanine diet in newborns with PKU have resulted in an increased number of women with PKU who are now of childbearing age. In the past, dietary restriction was discontinued when children with PKU reached adolescence. During pregnancy, however, phenylalanine easily crosses the placenta, causing intrauterine growth retardation and subsequently severe mental retardation and microcephaly. Data suggest that normalizing serum phenylalanine levels before conception results in children with normal intelligence and no increased rate of birth defects (Rouse et al., 1997; Kirby, 1999).

Full access? Get Clinical Tree
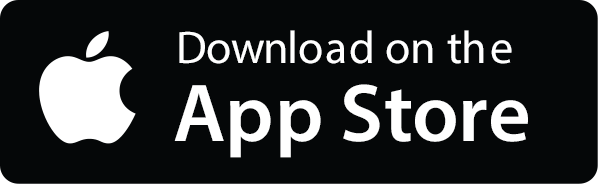
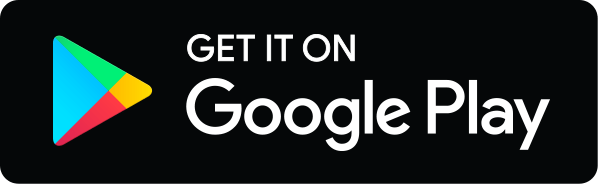