Anatomy
The liver is divided into four lobes consisting of 50,000 to 100,000 individual hepatic lobules (Fig. 32-1). Blood flows past hepatocytes via sinusoids from branches of the portal vein and hepatic artery to a central vein. There is usually only one layer of hepatocytes between sinusoids so the total area of contact with plasma is great. Central veins join to form hepatic veins, which drain into the inferior vena cava. Each hepatocyte is also located adjacent to bile canaliculi, which coalesce to form the common hepatic duct. This duct and the cystic duct from the gallbladder join to form the common bile duct, which enters the duodenum at a site surrounded by the sphincter of Oddi (Fig. 32-2).3 The main pancreatic duct also unites with the common bile duct just before it enters the duodenum.


Hepatic lobules are lined by macrophages (derived from circulating monocytes) known as Kupffer cells, which phagocytize 99% or more of bacteria in the portal venous blood. This is crucial because the portal venous blood drains the gastrointestinal tract and usually contains colon bacteria.
Endothelial cells that line the hepatic lobules contain large pores, permitting easy diffusion of certain substances, including plasma proteins, into extravascular spaces of the liver that connect with terminal lymphatics. The extreme permeability of the lining of endothelial cells allows large quantities of lymph to form, which contain protein concentrations that are only slightly less than the protein concentration of plasma. Indeed, approximately one-third to one-half of all the lymph is formed in the liver.
Hepatic Blood Flow
The liver receives a dual afferent blood supply from the hepatic artery and portal veins (Fig. 32-3). Total hepatic blood flow is approximately 1,450 mL per minute or approximately 29% of the cardiac output. Of this amount, the portal vein provides 75% of the total flow but only 50% to 55% of the hepatic oxygen supply because this blood is partially deoxygenated in the preportal organs and tissues (gastrointestinal tract, spleen, pancreas). The hepatic artery provides only 25% of total hepatic blood flow but provides 45% to 50% of the hepatic oxygen requirements. Hepatic artery blood flow maintains nutrition of connective tissues and walls of bile ducts. For this reason, loss of hepatic artery blood flow can be fatal because of ensuing necrosis of vital liver structures. An increase in hepatic oxygen requirements is met by an increase in oxygen extraction rather than a further increase in the already high hepatic blood flow.

Control of Hepatic Blood Flow
Portal vein blood flow is controlled primarily by the arterioles in the preportal splanchnic organs. This flow, combined with the resistance to portal vein blood flow within the liver, determines portal venous pressure (normally 7 to 10 mm Hg) (see the section “Portal Venous Pressure”). Sympathetic nervous system innervation is from T3 to T11 and is mediated via α-adrenergic receptors. This innervation is principally responsible for resistance and compliance of hepatic venules. Changes in hepatic venous compliance play an essential role in overall regulation of cardiac output and the reservoir function of the liver (see the section “Reservoir Function”).
Fibrotic constriction characteristic of hepatic cirrhosis (most often due to chronic alcohol abuse and hepatitis C) can increase resistance to portal vein blood flow, as evidenced by portal venous pressures of 20 to 30 mm Hg (portal hypertension). The resulting increased resistance to portal vein blood flow may result in development of shunts (varices) to allow blood flow to bypass the hepatocytes. Conversely, congestive heart failure and positive pressure ventilation of the lungs impair outflow of blood from the liver because of increased central venous pressure, which is transmitted to hepatic veins. Ascites results when increased portal venous pressures cause transudation of protein-rich fluid through the outer surface of the liver capsule and gastrointestinal tract into the abdominal cavity. Hepatic artery blood flow is influenced by arteriolar tone that reflects local and intrinsic mechanisms (autoregulation). For example, a decrease in portal vein blood flow is accompanied by an increase in hepatic artery blood flow by as much as 100%. Presumably, a vasodilating substance such as adenosine accumulates in the liver when portal vein blood flow decreases, leading to subsequent hepatic arterial vasodilation and washout of the vasodilating material.
Halothane decreases hepatic oxygen supply more than isoflurane, enflurane, desflurane, or sevoflurane when administered in equal potent doses.4 In contrast to the other volatile anesthetics, halothane preserves autoregulation of hepatic blood flow only to a limited extent and only when used in doses that do not decrease systemic blood pressure >20%. Surgical stimulation may further decrease hepatic blood flow, independent of the anesthetic drug administered. The greatest decreases in hepatic blood flow occur during intraabdominal operations, presumably due to mechanical interference of blood flow produced by retraction in the operative area, as well as the release of vasoconstricting substances such as catecholamines.
Reservoir Function
The liver normally contains approximately 500 mL of blood or approximately 10% of the total blood volume. An increase in central venous pressure causes back pressure, and the liver, being a distendible organ, may accommodate as much as 1 L of extra blood. As such, the liver acts as a storage site when blood volume is excessive, as in congestive heart failure, and is capable of supplying extra blood when hypovolemia occurs. Indeed, the large hepatic veins and sinuses are constricted by stimulation from the sympathetic nervous system, discharging up to 350 mL of blood into the circulation. Therefore, the liver is the single most important source of additional blood during strenuous exercise or acute hemorrhage.
Bile Secretion
Hepatocytes continually form bile (500 mL daily) and then secrete it into bile canaliculi, which empty into progressively larger ducts, ultimately reaching the common bile duct (see Fig. 32-2).3 Between meals, the tone of the sphincter of Oddi, which guards the entrance of the common bile duct into the duodenum, is high. As a result, bile flow is diverted into the gallbladder, which has a capacity of 35 to 50 mL. The most potent stimulus for emptying the gallbladder is the presence of fat in the duodenum, which evokes the release of the hormone cholecystokinin by the duodenal mucosa. This hormone enters the circulation and passes to the gallbladder, where it causes selective contraction of the gallbladder smooth muscle. As a result, bile is forced from the gallbladder into the duodenum. When adequate amounts of fat are present, the gallbladder empties in approximately 1 hour.
The principal components of bile are bile salts, bilirubin, and cholesterol.
Bile Salts
Bile salts combine with lipids in the duodenum to form water-soluble complexes (micelles) that facilitate gastrointestinal absorption of fats (triglycerides) and fat-soluble vitamins. Once absorbed, bile salts return to the liver via the portal vein, where they enter hepatocytes (enterohepatic circulation). In the absence of bile secretion, steatorrhea and a deficiency of vitamin K develop in a few days. Vitamin K is necessary for activation of several of the clotting factors that contain glutamic acid residues.
Bilirubin
After approximately 120 days, the cell membranes of erythrocytes rupture, and the released hemoglobin is converted to bilirubin in reticuloendothelial cells (Fig. 32-4).5 The resulting bilirubin is released into the circulation and transported in combination with albumin to the liver. In hepatocytes, bilirubin dissociates from albumin and conjugates principally with glucuronic acid. Unlike conjugated bilirubin, unconjugated bilirubin may be neurotoxic and may even cause a rapidly fatal encephalopathy. In the gastrointestinal tract, bilirubin is converted by bacterial action mainly into urobilinogen.

Jaundice
Jaundice is the yellowish tint of body tissues that accompanies accumulation of bilirubin in extracellular fluid. Skin color usually begins to change when the plasma concentration of bilirubin increases to approximately three times normal. The most common types of jaundice are hemolytic jaundice, due to increased destruction of erythrocytes, and obstructive jaundice, due to obstruction of bile ducts.
Cholesterol
Cholesterol is an important component of cell walls (synthesized in tissues from acetate in a reaction catalyzed by β-hydroxy β-methylglutaryl coenzyme A) and is transported from the periphery to the liver as high-density lipoproteins (HDL). Once cholesterol has reached the liver, it can be excreted in the bile in association with bile acids. Cholesterol in the bile may precipitate as gallstones if there is excess absorption of water in the gallbladder or the diet contains too much cholesterol. Gallstones occur in 10% to 20% of individuals; 85% are cholesterol stones.
Metabolic Functions
Metabolism of carbohydrates, lipids, and proteins depends on normal hepatic function (see Chapter 33). Furthermore, the liver is an important storage site for vitamins and iron. Degradation of certain hormones (catecholamines and corticosteroids), as well as drugs, is an important function of the liver. Hepatocytes are the principal site for synthesis of all the coagulation factors, with the exception of von Willebrand factor and factor VIIIC. Because the half-life of clotting factors produced in the liver is short, coagulation is particularly sensitive to acute hepatocellular damage.
Carbohydrates
Regulation of blood glucose concentration is an important metabolic function of the liver. When hyperglycemia is present, glycogen is deposited in the liver, and when hypoglycemia occurs, glycogenolysis provides glucose. Amino acids can be converted to glucose by gluconeogenesis when the blood glucose concentration is decreased.
Lipids
The liver is responsible for β-oxidation of fatty acids and formation of acetoacetic acid. Triglycerides are formed from the esterification of glycerol with three molecules of fatty acid. Pancreatic lipases and esterases are important in facilitating the absorption of dietary fats. After absorption, fat may be stored as triglycerides (reserve energy) or metabolized to energy. Lipoproteins, cholesterol, and phospholipids, such as lecithin, are formed in the liver. Synthesis of fats from carbohydrates and proteins also occurs in the liver.
Proteins
The most important liver functions in protein metabolism are oxidative deamination of amino acids, formation of urea for removal of ammonia, formation of plasma proteins and coagulation factors, and interconversions (transfer of one amino group to another amino acid) among different amino acids. Albumin formed in the liver is critically important for maintaining plasma oncotic pressure as well as providing an essential transport role. The half-life for albumin is about 21 days; therefore, plasma albumin concentrations are unlikely to be significantly altered in acute hepatic failure. Deamination of amino acids is required before these substances can be used for energy or converted into carbohydrates or fats. Decreases in portal vein blood flow, as may occur with the surgical creation of a portocaval shunt to treat esophageal varices, can result in fatal hepatic coma because of accumulation of ammonia.
Gastrointestinal Tract
The primary function of the gastrointestinal tract is to provide the body with a continual supply of water, electrolytes, and nutrients. To achieve this goal, the contents of the gastrointestinal tract must move through the entire system at an appropriate rate for digestive and absorptive functions to occur. Each part of the gastrointestinal tract is adapted for specific functions such as (a) passage of food in the esophagus, (b) storage of food in the stomach or fecal matter in the colon, (c) digestion of food in the stomach and small intestine, and (d) absorption of the digestive end products and fluids in the small intestine and proximal parts of the colon. Overall, approximately 9 L of fluid and secretions enters the gastrointestinal tract daily, and all but approximately 100 mL is absorbed by the small intestine and colon (Fig. 32-5).6 The pH of gastrointestinal secretions varies widely (Table 32-2).


Anatomy
The smooth muscle of the gastrointestinal tract is a syncytium such that electrical signals originating in one smooth muscle fiber are easily propagated from fiber to fiber. Mechanical activity of the gastrointestinal tract is enhanced by stretch and parasympathetic nervous system stimulation, whereas sympathetic nervous system stimulation decreases mechanical activity to almost zero.
Tonic contraction of gastrointestinal smooth muscle at the pylorus, ileocecal valve, and anal sphincter helps regulate the rate at which materials move through the gastrointestinal tract. In these parts of the gastrointestinal tract, rhythmic movements (peristalsis) occur 3 to 12 times per minute to facilitate mixing and movement of food.
Blood Flow
Most of the blood flow to the gastrointestinal tract is to the mucosa to supply energy needed for producing intestinal secretions and absorbing digested materials. Blood flow parallels digestive activity of the gastrointestinal tract. Approximately 80% of portal vein blood flow originates from the stomach and gastrointestinal tract, with the remainder coming from the spleen and pancreas.
Stimulation of the parasympathetic nervous system increases local blood flow at the same time it increases glandular secretions. Conversely, stimulation of the sympathetic nervous system causes vasoconstriction of the arterial supply to the gastrointestinal tract. The decrease in blood flow, however, is transient because local metabolic vasodilator mechanisms elicited by ischemia return blood flow toward normal. The importance of this transient sympathetic nervous system–induced vasoconstriction is that it permits shunting of blood from the gastrointestinal tract for brief periods during exercise, or when increased blood flow is needed by skeletal muscles or the heart.
Portal Venous Pressure
The liver offers modest resistance to blood flow from the portal venous system. As a result, the pressure in the portal vein averages 7 to 10 mm Hg, which is considerably higher than the almost zero pressure in the inferior vena cava. Cirrhosis of the liver, most frequently caused by alcoholism, is characterized by increased resistance to portal vein blood flow due to replacement of hepatic cells with fibrous tissue that contracts around the blood vessels. The gradual increase in resistance to portal vein blood flow produced by cirrhosis of the liver causes large collateral vessels to develop between the portal veins and the systemic veins. The most important of these collaterals are from the splenic veins to the esophageal veins. These collaterals may become so large that they protrude into the lumen of the esophagus, producing esophageal varicosities. The esophageal mucosa overlying these varicosities may become eroded, leading to life-threatening hemorrhage.
In the absence of the development of adequate collaterals, sustained increases in portal vein pressure may cause protein-containing fluid to escape from the surface of the mesentery, gastrointestinal tract, and liver into the peritoneal cavity. This fluid, known as ascites, is similar to plasma, and its high protein content causes an increased colloid osmotic pressure in the abdominal fluid. This high colloid osmotic pressure draws additional fluid from the surfaces of the gastrointestinal tract and mesentery into the peritoneal cavity.
Splenic Circulation
The splenic capsule in humans, in contrast to that in many lower animals, is nonmuscular, which limits the ability of the spleen to release stored blood in response to sympathetic nervous system stimulation. A small amount (150 to 200 mL) of blood is stored in the splenic venous sinuses and can be released by sympathetic nervous system–induced vasoconstriction of the splenic vessels. Release of this amount of blood into the systemic circulation is sufficient to increase the hematocrit 1% to 2%.
The spleen functions to remove erythrocytes from the circulation. This occurs when erythrocytes reenter the venous sinuses from the splenic pulp by passing through pores that may be smaller than the erythrocyte. Fragile cells do not withstand this trauma, and the released hemoglobin that results from their rupture is ingested by the reticuloendothelial cells of the spleen. These same reticuloendothelial cells also function, much like lymph nodes, to remove bacteria and parasites from the circulation. Indeed, asplenic patients are more prone to developing bacterial infections.
During fetal life, the splenic pulp produces erythrocytes in the same manner as does the bone marrow in the adult. As the fetus reaches maturity, however, this function of the spleen is lost.
Innervation
The gastrointestinal tract receives innervation from both divisions of the autonomic nervous system as well as from an intrinsic nervous system consisting of the myenteric plexus, or Auerbach plexus, and the submucous plexus, or Meissner plexus. In the absence of sympathetic nervous system or parasympathetic nervous system innervation, the motor and secretory activities of the gastrointestinal tract continue, reflecting the function of the intrinsic nervous system. Signals from the autonomic nervous system influence the activity of the intrinsic nervous system. For example, impulses from the parasympathetic nervous system increase intrinsic activity, whereas signals from the sympathetic nervous system decrease intrinsic activity. A large number of neuromodulatory substances act in the gastrointestinal tract.
The cranial component of parasympathetic nervous system innervation to the gastrointestinal tract (esophagus, stomach, pancreas, small intestine, colon to the level of the transverse colon) is by way of the vagus nerves. The distal portion of the colon is richly supplied by the sacral parasympathetics via the pelvic nerves from the hypogastric plexus. Fibers of the sympathetic nervous system destined for the gastrointestinal tract pass through ganglia such as the celiac ganglia.
Motility
The two types of gastrointestinal motility are mixing contractions and propulsive movements characterized as peristalsis. The usual stimulus for peristalsis is distension. Peristalsis occurs only weakly in portions of the gastrointestinal tract that have congenital absence of the myenteric plexus. Peristalsis is also decreased by increased parasympathetic nervous system activity and anticholinergic drugs.
Ileus
Trauma to the intestine or irritation of the peritoneum as follows abdominal operations causes adynamic (paralytic) ileus. Peristalsis returns to the small intestine in 6 to 8 hours, but colonic activity may take 2 to 3 days. Adynamic ileus can be relieved by a tube placed into the small intestine and aspiration of fluid and gas until the time when peristalsis returns.
Salivary Glands
The principal salivary glands (parotid and submaxillary) produce 0.5 to 1.0 mL per minute of saliva (pH 6 to 7), largely in response to parasympathetic nervous system stimulation. Saliva washes away pathogenic bacteria in the oral cavity as well as food particles that provide nutrition for bacteria. In the absence of saliva, oral tissues are likely to become ulcerated and infected. The bicarbonate ion concentration in saliva is two to four times that in plasma, and the high potassium content of saliva can result in hypokalemia and skeletal muscle weakness if excess salivation persists.
Esophagus
The esophagus serves as a conduit for passage of food from the pharynx to the stomach. The swallowing or deglutition center located in the medulla and lower pons inhibits the medullary ventilatory center, halting breathing at any point to allow swallowing to proceed. The upper and lower ends of the esophagus function as sphincters to prevent entry of air and acidic gastric contents, respectively, into the esophagus. The sphincters are known as the upper esophageal (pharyngoesophageal) sphincter and lower esophageal (gastroesophageal) sphincter.
Lower Esophageal Sphincter
The lower esophageal sphincter regulates the flow of food between the esophagus and the stomach. The sphincter mechanism at the lower end of the esophagus consists of the intrinsic smooth muscle of the distal esophagus and the skeletal muscle of the crural diaphragm.7 Under normal circumstances, the lower esophageal sphincter is approximately 4 cm long. The crural diaphragm, which forms the esophageal hiatus, encircles the proximal 2 cm of the sphincter. The intraluminal pressure of the esophagogastric junction is a measure of the strength of the antireflux barrier and is typically quantified with reference to the intragastric pressure (normal <7 mm Hg). Both the lower esophageal sphincter and the crural diaphragm contribute to the intragastric pressure. Muscle tone in the lower esophageal sphincter is the result of neurogenic and myogenic mechanisms. A substantial part of the neurogenic tone in humans is due to cholinergic innervation via the vagus nerves. The presynaptic neurotransmitter is acetylcholine, and the postsynaptic neurotransmitter is nitric oxide.
The normal lower esophageal sphincter pressure is 10 to 30 mm Hg at end-exhalation.7 Transient relaxation of the lower esophageal sphincter is a neural reflex mediated through the brainstem. Gastric barrier pressure is calculated as lower esophageal sphincter pressure minus intragastric pressure. This barrier pressure is considered the major mechanism in preventing reflux of gastric contents into the esophagus. Gastric distension, meals high in fat, and pharyngeal stimulation are two possible mechanisms by which the afferent stimulus that initiates transient relaxation of the lower esophageal sphincter may originate.8 Cricoid pressure decreases lower esophageal sphincter pressure, presumably reflecting stimulation of mechanoreceptors in the pharynx created by the external pressure on the cricoid cartilage (Fig. 32-6).9–11 General anesthesia decreases lower esophageal sphincter pressure 7 to 14 mm Hg, depending on the degree of skeletal muscle relaxation.12 Normally, upper esophageal sphincter pressure prevents regurgitation into the pharynx in the awake state. The administration of anesthetic drugs may decrease upper esophageal sphincter pressure even before the loss of consciousness.12


Full access? Get Clinical Tree
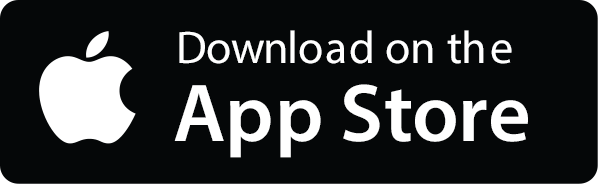
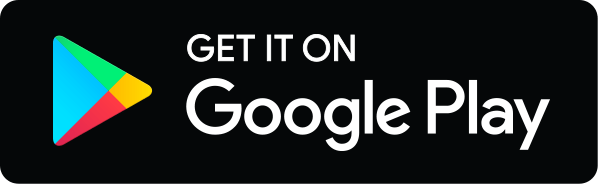