Gas Embolism Syndromes: Venous Gas Emboli, Arterial Gas Emboli, and Decompression Sickness
Mark M. Wilson
The gas embolism syndromes are known to occur in many different settings and may result in life-threatening emergencies. The clinical manifestations of these disorders are varied and the final pathophysiologic consequences depend on where the gas bubbles obstruct the circulation and how they impact the surrounding tissue. The nervous system, heart, lungs, and skin are the primary organ systems involved. The diagnosis of a gas embolism syndrome can be very difficult to establish. Clinicians must depend on a high level of suspicion in the appropriate settings to rapidly identify the problem, prevent further gas entry into the circulation, and begin effective treatment. Each of these entities is discussed in more detail based on the predominant location of the gas collections, although they are not always separate and distinct.
Venous Gas Embolism
Although the actual incidence of venous gas embolism (VGE) in the United States is unknown, it has been estimated conservatively that at least 20,000 cases of “air” embolism occur annually [1]. The consequences of VGE range from clinically undetectable to being rapidly fatal.
Etiology
Clinical reports emphasize the high incidence of VGE in association with traumatic injuries and invasive procedures involving the head, neck, and chest (Table 61.1) [1]. Only the most common causes are discussed in detail here.
Surgical
Virtually any surgical procedure that transiently exposes an open vein to a relative negative pressure may be associated with VGE. The best-studied surgical procedure known to be commonly associated with VGE is craniotomy performed in the Fowler’s (sitting) position. When monitors for VGE are prospectively used [1], VGE has been documented in 21% to 32% of all craniotomies and up to 58% of occipital craniotomies. Air may also enter the venous system via the occipital emissary veins, the dural sinuses, the diploic veins, the veins of tumors, or through burr holes.
Childbirth, hysterectomy, and abortion have been associated with an increased incidence of VGE [1]. It has been estimated that VGE causes 1% of maternal deaths. The incidence of VGE during cesarean section has been reported to be on the order of 39% to 71% overall, and the majority of episodes occur during uterine repair and placenta removal. During pregnancy, the veins of the uterus are exposed and fixed; when traumatized, they remain open and may serve as a portal of entry for gaseous emboli.
Prospective Doppler monitoring studies have documented a 31% to 83% incidence of VGE during total hip replacement [1]. The presumptive mechanism of embolization involves the forcible entry of air into the venous circulation through vascular openings in the bony medulla of the femur as a result of the high pressures generated in the distal shaft when the prosthesis is inserted.
Sinus lavage and dental surgical procedures have resulted in fatal cases of VGE [1]. Emboli are the result of intraosseous irrigation with water or air under pressure (at least 80 cm H2O). There are no data available in the literature to suggest just how often VGE occurs during these procedures.
Trauma
Open or penetrating wounds—especially of the chest, neck, head, heart, spine, abdomen, and pelvis—may result in VGE due to the exposure of an open vein to a relative positive pressure gradient (i.e., atmospheric pressure as compared with
central venous pressure) [1]. Pneumothorax or pneumoperitoneum may result in VGE by the inadvertent puncture of intraabdominal or intrathoracic blood vessels during the mechanism of injury.
central venous pressure) [1]. Pneumothorax or pneumoperitoneum may result in VGE by the inadvertent puncture of intraabdominal or intrathoracic blood vessels during the mechanism of injury.
Table 61.1 Causes of Venous Gas Embolism | |
---|---|
|
Some of the more unusual cases of traumatic embolization include reports of self-induced VGE due to urethral insufflation with an atomizer bulb, scrotal injection of air with a bicycle pump, and attempted suicide in hospital by forcible breathing into an intravenous line [1].
Diagnostic and Therapeutic Procedures
Air embolism in the setting of central venous catheterization has an unknown overall incidence, probably because the diagnosis is made only with large emboli. This fact also impacts the reported mortality rate in the literature of 29% to 43%, and it is possibly as high as 50% [1]. Morbidity is also significant because 42% of all survivors of recognized VGE were left with neurologic deficits. Air can enter the central venous system in several different ways: (a) during needle/wire/catheter insertion; (b) with fracture of the catheter, malfunction of a self-sealing diaphragm, or detachment of external connections; (c) after removal of a catheter that has been in place for several days, such that air is “sucked” into an open subcutaneous tissue tunnel that has formed a skin tract; and (d) as a result of a piggyback infusion running dry [2].
Thoracoscopy may produce VGE presumably due to the associated pneumothorax. Lung biopsy by percutaneous or bronchoscopic techniques creates a direct traumatic opening at the blood–air interface. Significant embolization might result whenever a medium-sized vein is exposed [1].
Gas insufflation procedures have been associated with gaseous embolization [1]. Diagnostic procedures involving the female genital tract, urethra, urinary bladder, kidney, retroperitoneal and perirenal spaces, peritoneal and pleural cavities, joints, cerebral ventricles, epidural space, and paranasal sinuses all carry a risk for VGE. To minimize this risk, the volume of gas introduced, the pressure resulting within the cavity, and the rate of injection should always be as low as possible. It has been suggested that carbon dioxide (CO2) should be used as the insufflating agent whenever possible due to its high blood solubility and rapid clearance. This last recommendation begs a word of caution, however, because VGE-associated deaths have been reported even with the use of CO2 [1].
Placement of epidural catheters for anesthesia has been noted prospectively to be associated with Doppler-detectable VGE [1]. In pregnant women placed in the left lateral decubitus position, VGE was noted to occur in 43%, almost half of whom were at least briefly symptomatic. The underlying mechanism relates to the rich plexus of veins of the epidural space, mostly anterior and lateral to the spinal cord. These veins are susceptible to trauma from a needle if the puncture is not directly in the midline or if the needle is rotated once in the epidural space. Because there are no valves in this plexus of veins, the intravascular pressure likely closely follows the central venous pressure. In the left lateral decubitus position, the site of puncture is above the level of the right atrium (RA), and, in pregnant women, uterine compression of the inferior vena cava is relieved, both of which serve to create a subatmospheric pressure in the epidural venous plexus.
Thermal tissue-ablation procedures using application of heat or cold have been associated with VGE [1]. Laser ablation/coagulation of tissues requires a continuous method for cooling of the laser tip. In general, these methods have involved using liquid (saline) or gas [air, nitrogen (N2), CO2]. Reports exist in the literature of the entry of these compressed gases into the venous circulation due to opening of vascular channels during the ablative procedure [1]. At the other temperature extreme, cryosurgery with instillation of liquid N2 is used to extend the surgical margin of excision in many cancer operations. Direct contact between the tissues and the liquid N2 may lead to entry of N2 into the circulation in the gaseous state [1]. N2 gas expands as it is warmed to a volume of greater than 500 times that it occupied in the liquid state. Gas emboli of this magnitude could be rapidly fatal.
Use of hydrogen peroxide (H2O2) in closed spaces or body cavities has been shown to result in VGE [1]. Animal and human studies have shown that H2O2 is readily absorbed from the intestines and the peritoneum. Oxygen (O2) emboli arise from the systemic absorption of H2O2 as catalase-induced decomposition causes release of water and molecular O2. One milliliter of a 3% H2O2 solution releases an estimated 10 mL of O2 on contact with catalase [1], which is abundant in human blood.
Case reports of VGE during blood donation and insertion of peripheral intravenous catheters illustrate that there are no circumstances in which a vein is exposed to atmospheric pressure that the hazard of embolization is nonexistent [1].
Pathophysiology
Entry of Gas into the Circulation
VGE has been shown to occur with patients in essentially any position [1]. The critical factor common to all VGE lies in the
pressure gradient created between the right side of the heart and the level of the open vessel. Any increase in the distance of the open vessel above the level of the heart or any decrease in intrathoracic pressure would increase the likelihood of air entering the venous circulation and traveling to the heart. For each 5 in. in vertical height above the level of the RA, there is an approximately 9.3 mm Hg decrease in local blood pressure. Any decrease in mean intrathoracic pressure or mechanisms resulting in a contracted blood volume or low central venous pressure will tend to enhance any existing venous pressure gradient.
pressure gradient created between the right side of the heart and the level of the open vessel. Any increase in the distance of the open vessel above the level of the heart or any decrease in intrathoracic pressure would increase the likelihood of air entering the venous circulation and traveling to the heart. For each 5 in. in vertical height above the level of the RA, there is an approximately 9.3 mm Hg decrease in local blood pressure. Any decrease in mean intrathoracic pressure or mechanisms resulting in a contracted blood volume or low central venous pressure will tend to enhance any existing venous pressure gradient.
Large amounts of gas can rapidly pass into the venous system under the proper conditions. Calculations indicate that approximately 100 mL of air per second would enter a vessel via a No. 14-gauge needle with only a 5 cm H2O pressure gradient across it.
Travel of Gas to the Heart
Once gas has entered the venous circulation, it travels toward the point of lower pressure until it reaches an obstruction. Animal studies have found that passage of air emboli through the superior vena cava can be retarded or the air even retained at sites proximal to the superior vena cava for an indefinite period [1].
Large venous gas emboli are capable of lodging and then obstructing blood flow in the heart and the pulmonary vasculature [1]. Grossly, these events have been observed to cause immediate dilation of the RA, the right ventricle (RV), and the pulmonary outflow tract. A rapidly expanding zone of RV ischemia follows soon thereafter. Functional obstruction of the RV outflow tract may result due to an “air lock” phenomenon. A blood-froth mixture results from systolic compression of the compressible gas phase with the noncompressible whole-blood phase. This concoction is then able to expand during diastole, the net result being an inadequate pumping action of the RV. It has been postulated that turbulent blood flow results from this “whipping” type of action or from vortex flow around partially obstructing collections of air bubbles. This whipping subsequently enhances fibrin formation, platelet aggregation, and coalescence of intravascular fat.
Smaller collections of air may not impair the heart and they may pass directly to the pulmonary arteries. Larger collections enter the pulmonary arteries with associated collections of fat and fibrin emboli.
Fate of Gas Emboli
Bubbles with the smallest initial radii have the shortest life span and are occasionally seen to pass directly through a capillary bed after attaining a radius of approximately 5 μm [1]. The bulk of excretion of gaseous emboli is accounted for by molecular diffusion across the arteriolar wall into the alveolar spaces. The rate of washout is related to RV performance and mean pulmonary artery (PA) pressure [1].
Surface-tension relationships, vascular pressures, and the size range of the bubbles are several additional interacting factors that may influence passage of emboli across the lungs. Also, the composition of the gas influences the size of the bubbles and the rate of dissolution in the blood. Bubbles of air or N2 are expected to remain in the blood for longer periods of time than O2 or CO2, especially if the ventilatory gases resemble room air composition. This relationship is due to the similarity in the partial pressures of the gases inside the bubbles with those of the surrounding blood, as well as to the different solubilities of the gases. Tonic factors affecting the diameter of pulmonary vessels (e.g., anesthetic agents, neurogenic or hypoxic pulmonary vasoconstriction, arterial tension of CO2, endogenous mediators) may also influence the passage of bubbles across the lungs.
Cardiopulmonary Consequences of Embolization
Pulmonary vascular obstruction is a major consequence of VGE and can lead to death. Obstruction to blood flow through the RV and through the pulmonary vascular system results from pulmonary vasoconstriction and from the mechanical impediment to flow imposed by the gas bubbles [1]. The change in PA pressures depends on whether the gas emboli are the result of a slow continuous infusion or a rapid bolus injection. In the first instance, a brisk increase in PA pressure to a level of up to 300% of baseline is seen [1]. This rapid increase phase is believed to be due to pulmonary vascular vasoconstriction and is followed by a plateau phase. The plateau response likely represents the opening of anatomic intrapulmonary shunts or a balance between the rate of gas infusion and rate of elimination. In contrast, when approximately 100 mL of air is injected as a bolus, PA pressure declines by as much as 20%, as the right heart is acutely stressed beyond its capabilities. Larger bolus injections (125 to 200 mL) are consistently fatal.
Pulmonary edema from VGE has been described anecdotally in humans [1]. Increased hydrostatic pulmonary vascular pressures (from mechanical occlusion of the PA and from induced vasoconstriction) and increased capillary permeability have been suggested as mechanisms for edema formation [1]. Regardless, the edema proves to be transient and reverses as the gas emboli are rapidly absorbed.
Maldistribution in ventilation–perfusion ([V with dot above]/[Q with dot above]) matching is the major factor leading to hypoxemia and changes in CO2 concentrations. With small amounts of continuous gas bubble infusion (0.2 mL per minute per kg) into the venous circulation, there is an increase in high [V with dot above]/[Q with dot above] areas in the lung. With larger volume gas emboli (0.75 to 2 mL per kg), however, shunting and an increase in the physiologic dead space have been shown to occur and to increase proportionally as the volume of embolic gas increases. This effect can involve as much as 35% of the total cardiac output, and it may be severe enough to cause CO2 retention in addition to hypoxemia.
The end-tidal CO2 concentration (ETCO2) decreases during VGE as a result of the increase in dead space caused by vascular obstruction. More simply, ETCO2 decreases as CO2 is “washed out” of alveoli that are ventilated but not perfused adequately. Inadequate [V with dot above]/[Q with dot above] matching may further worsen in the setting of a reduced cardiac output, resulting directly from VGE or indirectly as a consequence of non–embolic-related events (e.g., blood loss, myocardial ischemia, vasoactive medications). Any reduction in pulmonary blood flow decreases the delivery of air in the venous blood to the alveoli, thereby further decreasing the ETCO2.
Paradoxic Embolism
A paradoxic embolism may occur in the presence or absence of an anatomic intracardiac shunt. A gas embolism may elevate right-sided heart pressures, thus facilitating right-to-left shunting through a patent foramen ovale (PFO). Autopsy studies of patients with no history of cardiac disease document the presence of a probe-patent PFO in 25% to 35% of the general population [1].
Considered an anatomic variant, a probe-patent PFO is generally 1 to 10 mm in diameter and it remains functionally closed as long as left atrial pressure exceeds RA pressure. A reversal of the normal interatrial pressure gradient might be expected to increase the risk of paradoxic embolization. RA pressure has been demonstrated to be higher than left atrial pressure in the seated position in up to 54% of adult humans monitored during neurosurgical procedures [1]. The critical pressure necessary for gas bubbles to be forced through a probe PFO is not known, but it is likely to be small. After cardiac surgery, it has been shown that as little as a 4 mm Hg gradient can produce a 50% right-to-left intracardiac shunt [1].
Clinically, it may be important to distinguish between an anatomic PFO and functional PFO because it is the latter that has an impact on any morbidity and mortality experienced. It has been reported that paradoxic embolization occurs in only 15% to 25% of patients with a PFO [1]. Contrast echocardiography using agitated sterile saline given as a rapid intravenous bolus has documented a lower prevalence of functional PFO (i.e., 10% to 20%) [1], as compared to the known prevalence of 25% to 35% for anatomic PFO. The amount of contrast material crossing from the right heart to the left heart does not correlate with the magnitude of shunt flow, nor does the Valsalva maneuver provoke shunting in all patients with PFO [1]. The question remains as to whether this finding represents a low sensitivity of this method for detecting PFO or whether there are some anatomic PFOs that have no functional role. Consensus opinion is that all PFOs should be considered to have the potential for allowing paradoxic embolization. Multiple reports have documented that the absence of any agitated saline or color flow through the interatrial septum by echocardiography does not exclude the presence of a PFO; it only excludes the presence of a right-to-left interatrial shunt at that moment in time [1].
Bubble passage through the pulmonary circulation has been shown to occur in the absence of intracardiac communications when the rate of venous air infusion exceeds the rate of pulmonary filtration and excretion [1]. Paradoxic air embolization during cardiopulmonary bypass has been reported to occur in the absence of an intracardiac defect when the mean PA pressure exceeds approximately 30 mm Hg [1]. Animal research suggests the existence of this same “critical value” of PA pressure that, once exceeded, dramatically increases the tendency for paradoxic embolization [1]. This increase occurs presumably on the basis of direct arteriovenous anastomoses in the lung (seen only rarely, but may be as large as 500 μm in diameter), bronchopulmonary anastomoses (i.e., flow is from the PA to the bronchial veins and then to the pulmonary veins), or by routine transpulmonary passage of the gas across the capillary beds.
Factors Affecting Mortality
The size of the embolus, its rate of delivery, and the final destinations of the gaseous emboli are the most important factors influencing the severity of injury produced by VGE. In humans, accidental bolus injections of 100 and 300 mL of air have been reported to be fatal [1]. In critically ill patients with minimal cardiopulmonary reserve, smaller emboli could be expected to have a greater morbidity.
In the context of equal volumes, mortality is decreased if the embolism is of CO2 rather than air or O2. Animal work indicates that CO2 may be injected to 5 times the volume of O2 before symptoms of embolism appear, presumably due to its greater solubility in blood [1]. Although tolerated to a larger extent, it must be remembered that CO2 emboli are not entirely benign and may lead to similar clinical consequences as air embolization.
When nitrous oxide (N2O) is used for anesthesia, mortality is increased in the setting of VGE [1]. N2O attains a high blood concentration because of its high solubility (approximately 20-fold that of O2 and 34-fold that of N2). Because a large concentration gradient would exist between this blood and any air embolus, N2O would be expected to diffuse from the blood into the embolus. As a result, the embolus increases geometrically in size in direct relation to the partial pressure of N2O because the N2O molecules can diffuse from the blood into the air embolus much more rapidly than the N2 can be removed. The end result is a potential worsening of any generated physiologic abnormalities or delay in the ultimate resolution of the embolus. The presence of N2O in the anesthetic mixture has been shown to reduce the median lethal dose of a given volume of air by a factor of 3.4 [1].
Diagnosis
Clinical Manifestations
The symptoms of VGE are generally nonspecific. Patients may report feeling faint or dizzy, express a fear of impending doom, or even complain of dyspnea or substernal chest pain. This presentation, with or without paradoxic embolism, may mimic an acute cardiopulmonary or central nervous system (CNS) event. Severe VGE may present dramatically with elevated neck veins, “clear lungs,” and hypotension, and it may be rapidly followed by altered mental status and death. Because signs and symptoms are nonspecific, the importance of a detailed history, familiarity with the clinical situations in which VGE occurs, and a high degree of clinical suspicion cannot be overemphasized if one is to make an accurate diagnosis.
Physical Examination
Physical examination is usually not helpful in making the diagnosis. The only “specific” sign attributed to VGE is the classic mill-wheel murmur, otherwise reported only to occur in the rare syndrome of hydropneumopericardium. This murmur has been described as the rhythmic splashing or churning sound generated by the agitation of gas trapped with fluid in a closed space. Most often, it is only audible transiently and is heard infrequently at best, even in severe VGE. With large emboli and resultant cardiovascular collapse, a sound resembling the “squeezing of a wet sponge” has been described over the precordium [1].
VGE may occur without any change in vital signs. Wheezing as a result of acute bronchospasm may occasionally be heard. In a prospective study of seated neurosurgical patients, marked hypotension was noted in 78%, respiratory changes in 61%, and ventricular ectopy in 50% [1].
Laboratory Data
Abnormal results may include electrocardiogram (ECG) changes consistent with myocardial ischemia or acute cor pulmonale, premature ventricular contractions, and/or arterial blood gas findings of hypoxemia and hypercapnia.
Radiographic Findings
Chest radiography may verify the presence of VGE, but it should not be relied on for the diagnosis, especially in emergent situations. Air in the main PA is pathognomonic of pulmonary VGE, and it is recognized as a characteristic bell-shaped lucency in the distal main PA. This sign is seen very infrequently, especially in supine patients. Other patterns seen are focal upper-lung zone oligemia, central PA dilation, and air in the systemic veins or the arterial circulation [1]. Pulmonary edema ranging from hilar haziness to generalized vascular redistribution may occur soon after VGE, and it usually persists for at least 16 to 24 hours [1]. Noncardiogenic pulmonary edema has been reported [1], is usually self-limited, and resolves over several days. Progression of noncardiogenic pulmonary edema to full-blown acute respiratory distress syndrome has also been described [1].
Ventilation–Perfusion Lung Scans
VGE may produce patterns consistent with “high probability” for pulmonary venous thromboembolism interpretations. Prompt and complete resolution of these scintigraphic perfusion defects within 24 hours has been documented [1], and
it is probably characteristic for VGE. In contrast, perfusion defects produced by venous thromboembolism are known to resolve more slowly over a period of weeks to months and they may not ever resolve completely. Areas of [V with dot above]/[Q with dot above] matching (i.e., “indeterminate probability”) may coexist with [V with dot above]/[Q with dot above] mismatches, and they are believed to represent reflex bronchoconstriction in conjunction with occlusion of the PA or its branches. The decreased ventilation is apparently due to the release of bronchoconstricting agents, such as serotonin, from the occluded segments of the PA. This phenomenon is readily reversible within several hours if the PA occlusion is transient, and it is related to rapid resolution of the gaseous emboli.
it is probably characteristic for VGE. In contrast, perfusion defects produced by venous thromboembolism are known to resolve more slowly over a period of weeks to months and they may not ever resolve completely. Areas of [V with dot above]/[Q with dot above] matching (i.e., “indeterminate probability”) may coexist with [V with dot above]/[Q with dot above] mismatches, and they are believed to represent reflex bronchoconstriction in conjunction with occlusion of the PA or its branches. The decreased ventilation is apparently due to the release of bronchoconstricting agents, such as serotonin, from the occluded segments of the PA. This phenomenon is readily reversible within several hours if the PA occlusion is transient, and it is related to rapid resolution of the gaseous emboli.
Detection and Monitoring Method
Precordial Doppler monitoring is generally considered one of the more sensitive techniques for detecting emboli. Because a gas–blood interface is an excellent acoustic reflector, when an ultrasonographic beam strikes a moving gas bubble, a distinctive and characteristic artifact is heard above the background flow signal.
VGE may be missed by this technique due to changes in the position of the detector or blood pressure. False-positive reports of VGE may arise due to arrhythmia. The sound pattern induced by a junctional rhythm may easily mimic changes produced by VGE. With a junctional rhythm, cannon A-waves may be present due to contraction of the RA against a closed tricuspid valve. The resultant turbulence in the RA is detected and confused for VGE.
Serial measurements of PA pressure should be a useful monitoring technique due to the fact that even small emboli may produce significant increases in PA pressures, major increases in PA pressure do not occur unless at least 10% of the vasculature is obstructed, the rise in PA pressure is roughly proportional to embolus size, and the likelihood of paradoxic embolism increases above a mean PA pressure of 30 mm Hg [1].
ETCO2 and N2 levels fluctuate with VGE. Because these changes probably result primarily from significant mismatch, it would be anticipated that they would detect emboli later than Doppler techniques or changes in PA pressure and that they would be more likely to miss small emboli. Like PA pressure changes, however, variations in ETCO2 stay abnormal longer, and they are more closely related to the volume of gas embolized [1]. Potential confounding factors exist that may also cause a reduction in ETCO2 in the absence of a VGE-related event, and they include any set of circumstances that result in an acute decrease in cardiac output, increases in alveolar ventilation, or increases in alveolar dead space.
Consideration of the advantages and disadvantages of the available VGE detection technology suggests that a combination of transesophageal echocardiography or precordial Doppler ultrasonography with PA pressure, ETCO2, or transcutaneous O2 devices would provide the sensitivity, the quantitative determination, and the physiologic response monitoring necessary. Across the United States, use of ETCO2 monitoring in combination with precordial Doppler ultrasonography has become the primary, if not the standard, approach for VGE detection perioperatively.
Treatment
Because a fatal outcome may occur long before any diagnostic confirming tests can be performed, treatment must be initiated promptly at the earliest suspicion of gas embolization. Although no systematic studies comparing treatment modalities have been reported, improved detection of VGE appears to have decreased its severity. In combined retrospective and prospective analyses of seated neurosurgical procedures, a significant beneficial role was found for the use of routine precordial Doppler monitoring [1]. Before the advent of routine Doppler monitoring, VGE was clinically detected less often (5.7% before vs. 32% after), but the episodes noted had more severe sequelae. Once precordial Doppler monitoring became standard, the morbidity and mortality directly related to venous or arterial emboli was documented to be 0.5%. This improvement in event detection and reduction in the severity of VGE was ascribed to earlier recognition, allowing for earlier institution of therapy and prevention of further occurrences.
Routine Treatment Measures
Immediate measures should include identification of the site of gas entry and prevention of further gas entry, cessation or correction of exacerbating factors, administration of 100% O2, and changing position to the left lateral decubitus position. In most patients, the site of gas entry is readily apparent. Failure to stop gas entry in a timely fashion may be fatal. If there is suspicion of a low central blood volume, volume should be rapidly repleted. Immediate cessation of delivery of N2O and ventilating with 100% O2 facilitates resolution of any gas emboli experienced during anesthesia with this agent.
Because air emboli are composed of approximately 79% N2 and 21% O2, any maneuver that rapidly increases the elimination of dissolved N2 should decrease the size of the embolus. Administration of 100% O2 achieves this goal by washing N2 out of the alveoli and by creating a favorable gradient for N2 to cross into the alveolus from the blood.
Placing patients in the left lateral decubitus position may facilitate movement of any air obstructing the pulmonary outflow tract toward the apex of the RV, thereby relieving the obstruction and improving survival.
Aspiration and Dislodgement
In patients with witnessed gas embolism or in whom monitoring techniques suggest that the gas is still trapped in the heart, attempts can be made to aspirate or dislodge the gas. Gas may be aspirated from the heart by placing a central venous catheter into the RA or RV or pulling back a PA catheter and then aspirating serially from each successive heart chamber [1]. In unwitnessed gas embolism, this early phase has usually passed before the embolism is detected, and these interventions may result in more harm than benefit. Closed-chest compression may dislodge the embolus from the RV.
Hyperbaric Oxygen
When available, use of hyperbaric oxygen (HBO) may be helpful. HBO is the only therapy demonstrated to have any benefit well after VGE has been clinically established [1]. Even after emboli of 150 to 500 mL, HBO produced rapid improvement of all cardiopulmonary and neurologic abnormalities despite delays in initiating therapy of up to 20 hours. The most common HBO treatment protocols in use today are the U.S. Navy Treatment Tables 5, 6, and 6A [3]. Use of these Tables is discussed later in the Treatment section of Decompression Sickness. Although it is accepted that HBO should be instituted as early as possible, the literature supports that special consideration be given to this modality at late stages, even in a seemingly irrecoverable situation [1].
Managing Unwitnessed Venous Gas Embolism
Given that VGE may mimic or cause a clinical presentation that is difficult to distinguish from venous pulmonary thromboembolism (PE), RV infarct, myocardial infarction (MI), or stroke, clinicians may frequently feel reluctant to consider the difficult-to-establish diagnosis of VGE and to begin treatment until other causes are ruled out. The simple measures indicated
for the immediate management of VGE outlined herein are not contraindicated in the management of any of the other conditions typically in the differential diagnosis.
for the immediate management of VGE outlined herein are not contraindicated in the management of any of the other conditions typically in the differential diagnosis.
Little has been published on the clinical management of cardiovascular consequences of VGE. Because myocardial and RV infarct may frequently accompany large VGE, urgent, routine evaluation with ECG and echocardiography would be indicated in most cases. Because myocardial ischemia and subsequent MI in VGE probably result from hypoxemia, the effects of massive overdistention of the ventricle, and perhaps direct embolization of coronary vessels, the value of traditional management techniques for MI is not clear. There are no theoretical contraindications to the use of nitrates, aspirin, beta-blockers, calcium-channel blockers, or vasodilators in patients with ECG changes. The role of thrombolytic therapy in VGE is unclear.
Discrimination between PE and VGE in patients with unwitnessed, unmonitored events can be difficult. Patients at risk for VGE are frequently at risk for PE as well. Once gas has left the RV, changes in PA pressures and ETCO2 values may be similar in both conditions. As noted, the radiologic findings may be similar, but they may resolve within 24 hours with VGE. If the clinical suspicion of PE is high, there is no known contraindication to initiating appropriate anticoagulation in patients with VGE.
Prevention
Preventive measures are likely the most valuable management strategy for VGE. All patients undergoing the procedures listed in Table 61.1 [1] should be considered at high risk. In addition, hyperventilation, obstructive lung disease, and hypovolemia are common clinical conditions that increase the natural pressure gradient between atmospheric air and the central venous compartment; they may, therefore, also increase the chances of VGE during predisposing manipulations. Patients with a known PFO, pulmonary hypertension, previous MI with markedly reduced RV function, known right-to-left shunts, or congenital heart disease with any of the mentioned abnormalities should also be considered at high risk, not for experiencing an embolism per se, but for being susceptible for increased morbidity and mortality of a paradoxic embolism. A high false-negative rate (sensitivity, 64%) limits the usefulness of preoperative transthoracic echocardiography with Valsalva maneuver in predicting the presence of PFO and the risk of paradoxic emboli [1].
In general, patients should have procedures performed in a supine rather than upright position, and the point of potential air entry should be kept lower than the RA. Placement, manipulation, and removal of subclavian and internal jugular venous catheters are probably the most common clinical procedures during which specific measures can be performed to prevent substantial air embolization [2]. All patients should be placed in the Trendelenburg position, and they should be asked to perform the Valsalva maneuver or to hold their breath during needle/wire/catheter insertion. The operator should completely occlude the hub of the needle during manipulations to prevent open communication with atmospheric pressure. During removal of central catheters, patients should also be placed in the Trendelenburg position, the entry site should be compressed, and an occlusive dressing applied.

Full access? Get Clinical Tree
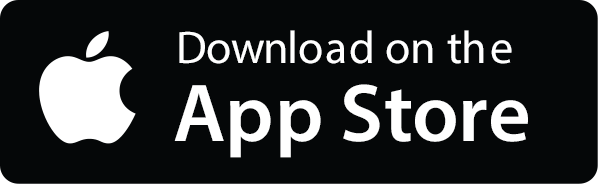
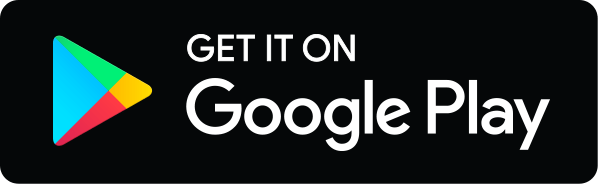
