CHAPTER 86 FUNDAMENTALS OF MECHANICAL VENTILATION
Mechanical ventilation is often required to manage trauma or critical illness, whether for airway protection, administration of general anesthesia, or management of acute respiratory failure (ARF) (Table 1). New technology now provides several modes by which a patient may be ventilated, with the goals of improved gas exchange, better patient comfort, and rapid liberation from the ventilator. Moreover, noninvasive positive-pressure ventilation permits some cases of ARF to be managed without insertion of an artificial endotracheal airway, and some patients who are extubated with marginal reserves to avoid reintubation. Nearly all ventilators can be set to allow full support of the patient on the one hand, and periods of exercise on the other. Thus, the choice of ventilator settings is a matter of physician preference for the majority of patients (Table 2). Controlled ventilation with suppression of spontaneous breathing leads rapidly to respiratory muscle atrophy; therefore modes of assisted ventilation are preferred wherein machine-delivered breaths are triggered by the patient’s own inspiratory efforts. Basic modes of assisted ventilation include assist-control ventilation (ACV), synchronized intermittent mandatory ventilation (SIMV), and pressure support ventilation (PSV).
Table 1 General Indications for Mechanical Ventilation
Table 2 Glossary of Basic Terminology of Mechanical Ventilation
Control: regulation of gas flow |
Cycling: ventilator switching from inhalation to exhalation after volume or pressure target (or limit) has been reached |
Triggering: causes the ventilator to cycle to inhalation |
Pressure-triggered—ventilator senses the patient’s inspiratory effort from a decrease of airway pressure |
Breaths: cause the ventilator to cycle from inhalation to exhalation |
Assisted—examples: assist control, synchronized intermittent mandatory ventilation, pressure support |
Flow pattern: constant, decelerating, or sinusoidal |
Constant— flow continues at a constant rate until the set tidal volume is delivered, seldom used in practice |
Mode (breath pattern) |
Controlled mechanical ventilation—controlled ventilation, without allowances for spontaneous breathing, typical of anesthesia ventilators Intermittent mandatory ventilation—admixes controlled and spontaneous breaths, which may also be synchronized to prevent “stacking” |
NONINVASIVE VENTILATION
PRESSURE SUPPORT VENTILATION
Positive end-expiratory pressure (PEEP, also called continuous positive airway pressure [CPAP]) is added to restore functional residual capacity (FRC) to normal for the patient. When lung volumes are low, the work of breathing during early inhalation is reduced. Noncompliant lungs require higher intrapleural pressures to inflate to a normal VT, even with CPAP. The addition of pressure support (PS) assists the patient to move up the pressure–volume curve (larger changes in volume for a given applied pressure, i.e., increased lung compliance). The term “pressure support ventilation” describes the combination of pressure support and PEEP (or CPAP). Although useful in the patient breathing spontaneously, PS may be used to assist spontaneous breaths in SIMV. Weaning may be facilitated using this combination, as the backup (SIMV) rate is weaned initially, and then the PS.
MODES OF MECHANICAL VENTILATION
Positive End-Expiratory Pressure
The ideal level of PEEP is controversial (Table 3). It may be that which prevents derecruitment of the majority of alveoli, while causing minimal overdistension; alternatively, application of PEEP is a recruitment maneuver, arguing for higher pressures to be applied to overcome alveolar collapse. Applying PEEP to put the majority of lung units on the favorable part of the pressure–volume curve will maximize gas exchange and minimize overdistention, but is easier said than done because the lower inflection point is sometimes indistinct. Undoubtedly, the combination of PEEP and low VT prevents volutrauma, but the exact amount of PEEP to apply is controversial. The reason for this is hysteresis—the tendency of the lungs, due to surfactant, to exist at higher volumes in exhalation than in inhalation.
Table 3 Protocol Summary for Institution of Mechanical Ventilation for Acute Lung Injury/Acute Respiratory Distress Syndrome
PEEP, Positive end-expiratory pressure.
Adapted from Nathens AB, Johnson JL, Mine JP, et al. Inflammation and the Host Response to Injury Investigators: Inflammation and the host response to injury, a large-scale collaborative project: patient-oriented research core—standard operating procedures for clinical care. I. Guidelines for mechanical ventilation of the trauma patient. J Trauma 59:764–769, 2005.
Routine Settings
Ventilator settings are based on the patient’s ideal body mass and medical condition. The risk of oxygen toxicity from prolonged exposure to a fraction of inspired oxygen (FIO2) greater than 60% is minimized by using the lowest FIO2 that can oxygenate arterial blood satisfactorily (e.g., arterial oxygen tension [PaO2] of 60 mm Hg or an oxygen saturation [SaO2] of 88%) (see Table 3).
The normal lung (e.g., during general anesthesia) may be ventilated safely with VT 8–10 ml/kg for prolonged periods. Historically, critically ill patients with acute lung injury (ALI)/acute respiratory distress syndrome (ARDS) have been ventilated with VT 10–15 ml/kg of ideal body mass, which is now considered inappropriate due to convincing data from experiments indicating that alveolar overdistention can produce endothelial, epithelial, and basement membrane injuries associated with increased microvascular permeability and iatrogenic lung injury (VILI). Direct monitoring of alveolar volume is not feasible. A reasonable substitute is to estimate peak alveolar pressure as obtained from the plateau pressure (Pplat) measured in a relaxed patient by occluding the ventilator circuit briefly at end-inspiration. In patients with pulmonary dysfunction, there is a growing tendency to reduce the VT delivered to 4–6 ml/kg or less in order to achieve a Pplat no higher than 35 cm H2O. The incidence of VILI increases markedly when Pplat is high. Low VT ventilation may lead to an increase in PaCO2. Acceptance of elevated CO2 tension in exchange for controlled alveolar pressure is termed “permissive hypercapnia.” It is important to focus on pH rather than PaCO2 if this approach is employed. If the pH falls below 7.25, increase VE or administer NaHCO3.

Full access? Get Clinical Tree
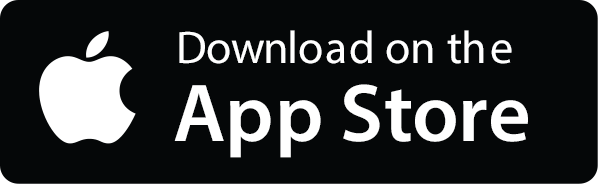
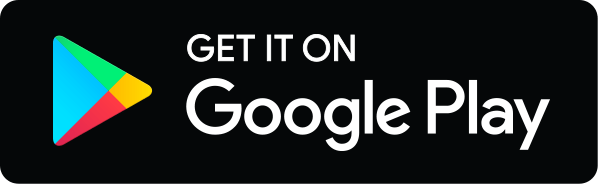