The four-column model of patient blood management (PBM) intended to avoid or minimize allogeneic transfusion in elective surgery, in order to improve the surgical patient’s postoperative outcome.
Management of intraoperative blood losses
An acute surgical blood loss will not immediately be compensated by the transfusion of red blood cells (RBC) and/or plasma. More likely, the shed blood will initially be replaced by infusion of RBC-free crystalloid and/or colloid solutions. This procedure is intended to maintain a normal circulating intravascular volume (i.e. normovolemia); at the same time, the dilution of all circulating blood components (normovolemic hemodilution) is tolerated.
Tolerance of dilutional anemia
Under general anesthesia, “normovolemic hemodilution” is tolerated down to very low Hb concentrations and hct values, without any risk for tissue perfusion, tissue oxygenation, and organ function. This reflects the large natural anemia tolerance of the human body.
The physiological mechanisms enabling this anemia tolerance consist in:[7]
(1) the increase of cardiac output (CO) – initially via the increase of ventricular stroke volume and later via additional tachycardia – depending on the degree of hemodilution;
(2) the increase of total body oxygen (O2) extraction;
(3) the physiological difference between macro- and microvascular (capillary) hct (“luxury hct”); the microvascular hct falls below its normal value only after a 30–50% reduction of the macrovascular hct;
(4) the physiological over-supply of organ tissues with O2 (“luxury” O2 delivery, DO2). Under normal conditions, DO2 exceeds tissue O2 requirements by a factor of 3 to 4 (point (1) in Figure 6.2). Thus DO2 can be reduced over a wide range without impairment of tissue oxygenation. Tissue O2 demand is satisfied, and tissue O2 consumption (VO2) remains constant (so-called O2 supply-independency of VO2) (Figure 6.2, segment (2)).
The compensatory mechanisms described are decisive for the extent of anemia tolerance. Several studies show that these mechanisms likewise exist in infants,[8] children,[9,10] elderly patients,[11] patients with cardiopulmonary disease,[12] and patients under pharmacological beta-receptor blockade.[13]
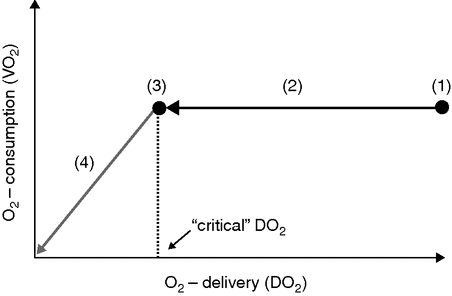
Schematic depicting the course of whole-body O2 delivery (DO2) and O2 consumption (VO2) during normovolemic hemodilution (e.g. replacement of intraoperative blood loss with red-blood-cell-free infusion solutions); modified according to [14]). The graphic has to be read from the right side (starting with normal DO2) to the left (increasing dilutional anemia). Explanations in text.
Limits of the natural anemia tolerance: concept of the “critical DO2” (DO2crit)
At an extreme degree of hemodilution, O2 demand will finally equal DO2 (Figure 6.2, point (3)). The corresponding DO2 is called “critical,” DO2crit. With ongoing hemodilution, DO2 falls below DO2crit, and the amount of O2 delivered to the tissues becomes insufficient to meet their O2 demand. As a consequence, the previously stable VO2 starts to decline (so-called O2 supply-dependency of VO2) (Figure 6.2, segment (4)) [14]. This sudden decrease of VO2 indirectly reflects the limit of anemia tolerance and the onset of tissue hypoxia. The energy needs of the body are now mainly met by anerobic glycolysis, and the serum lactate concentration therefore starts to rise. In analogy to DO2crit the Hb and hct values corresponding to the inflection point of VO2 are called Hbcrit and hctcrit, respectively. Without treatment (transfusion, hyperoxic ventilation) the persistence of the critical DO2, Hb, or hct leads to death within a short period of time.[15,16]
The whole body’s anemia tolerance may assume impressive proportions: in healthy awake volunteers, DO2crit was not met even after hemodilution to Hb 48 g/l.[17] In healthy anesthetized animals and patients, the limit of dilutional anemia was reported at hct values between 12% and 3% (Hb 33 and 11 g/l) (Table 6.1). Infants (1–7 months) [8] and children (12.5 years) [10] tolerated Hb of 30 g/l and lower without falling below their DO2crit. In pregnant sheep, fetal tissue oxygenation was preserved down to a maternal hct of 15% (Hb 50 g/l).[18]
The limit of anemia tolerance: overview of critical O2 transport parameters (critical hemoglobin concentration, Hbcrit, and critical hematocrit, hctcrit) determined in different species during extreme normovolemic hemodilution
Author | Species | Anesthesia | FiO2 | Blood exchange fluid | Identification of DO2crit | hctcrit(%) | Hbcrit (g/l) |
---|---|---|---|---|---|---|---|
Fontana et al. [10] | Man (child) | Isoflurane Sufentanil Vecuronium | 1.0 | Albumin | ST-segment depression | 21 | |
van Woerkens et al. [15] | Man (84 years) | Enflurane Fentanyl Pancuronium | 0.4 | Gelatin | Drop in VO2 | 12 | 40 |
Zollinger et al. [47] | Man (58 years) | Propofol Fentanyl Pancuronium | 1.0 | Gelatin | ST-segment depression | ∼11 | |
Cain [14] | Dog | Pentobarbital | 0.21 | Dextran | Drop in VO2 | 98 | 33 |
Perez-de-Sá et al. [48] | Pig | Isoflurane Fentanyl Midazolam Vecuronium | 0.5 | Dextran | Drop in VO2 | 23 ± 2 | |
Meier et al. [16] | Pig | Propofol Fentanyl | 0.21 | HES | Drop in VO2 | 31 ± 4 | |
Pape et al. [49] | Pig | Propofol Fentanyl Pancuronium | 0.21 | HES | Drop in VO2 | 24 ± 4 | |
Kemming et al. [21] | Pig | Midazolam Morphine Pancuronium | 0.21 | HES | ST-segment depression | 72 ± 12 | 26 ± 3 |
Meisner et al. [50] | Pig | Diazepam Morphine Pancuronium | 0.21 | Albumin | ST-segment depression | 61 ± 18 | 20 ± 8 |
Meier et al. [51] | Pig | Propofol Fentanyl Pancuronium | 0.21 | HES | Drop in VO2 | 24 ± 5 |
HES: hydroxyethylstarch.
Unfortunately, it is impossible to provide clinicians with universal numerical values for the aforementioned critical O2 transport parameters. DO2crit, Hbcrit, and hctcrit vary in and between individuals and depend on a variety of determining factors: adequate depth of anesthesia, hyperoxemia, muscular relaxation, and mild hypothermia increase anemia tolerance (see below); hypovolemia, restricted coronary reserve, heart failure, profound anesthesia, multiple trauma, and sepsis reduce anemia tolerance.
Moreover, it cannot be excluded that single organs will meet their DO2crit at a higher Hb (hct) than the whole organism and develop tissue hypoxia prior to the drop of global VO2. This would challenge whole-body global VO2 as a global monitoring parameter for tissue oxygenation.
In anesthetized subjects, the anemia tolerance of the whole body and the brain,[19] the myocardium with intact coronary perfusion,[20,21] or the splanchnic system [19] have been found to be equal. Kidney and skeletal muscle seem to have a lower anemia tolerance.[22] The situation is also different for the compromised heart with restricted coronary reserve. In anesthetized dogs with an experimental 50–80% coronary artery stenosis, signs of myocardial ischemia and/or functional deterioration appeared at Hb 70–100 g/l.[23] In a retrospective cohort-analysis of cardiac risk patients undergoing non-cardiac surgery and refusing allogeneic transfusion for religious reasons (affiliation to Jehovah’s Witnesses), a significantly higher 30-day mortality was found if the postoperative Hb fell below 80 g/l.[24] In otherwise healthy anesthetized rats, the limit of renal anemia tolerance was identified between Hb 4 and 70 g/l,[25] and in patients undergoing cardiac surgery at Hb between 70 and 80 g/l.[26,27]
In clinical practice it is difficult to identify the limit of the individual anemia tolerance of a single patient. Continuous measurement of VO2 is technologically complex, costly, and therefore restricted to scientific questions. Indirect clinical signs reflecting DO2crit, such as ECG changes (ST-segment deviation, arrhythmia), echocardiographic regional myocardial wall motion disturbance, lactacidosis and a decrease of mixed-venous or central-venous O2 saturation, are uncertain.
Support in estimating the significance of perioperative dilutional anemia quoad vitam is provided with the results of large clinical studies (generally performed on Jehovah’s Witnesses) investigating the relation between postoperative anemia and mortality. Until a postoperative Hb of 80 g/l no statistical relation between anemia and mortality could be detected even in older patients with pre-existing cardiopulmonary disease [24,28,29] and intensive care patients with multiple morbidity.[1,30,31] In anemic patients (Hb < 80 g/l) whose death was causally related to anemia, the Hb was always found to be below 50 g/l.[32] At Hb 30 g/l, mortality has been shown to increase up to 50% without transfusion.[33] Nevertheless, in individual cases much lower Hb (15 g/l and lower) has been survived without transfusion.
Therapeutic increase of anemia tolerance
In cases of unexpected massive blood losses and/or logistic difficulties impeding an immediate start of transfusion, the anemia tolerance of the patient can be effectively increased by the following measures:[34]
(1) Restoration and/or maintenance of normovolemia: the basic prerequisite for the effective compensation of dilutional anemia is normovolemia. In “hypovolemic hemodilution” the whole body’s O2 demand increases, mediated by catecholamines. Under hypovolemic conditions, DO2crit is met at higher values than under normovolemia, and anemia tolerance is reduced.
(2) Myocardial function: another basic prerequisite for optimal cardiac compensation of dilutional anemia is the increase of myocardial blood flow (MBF) realized by maximal coronary dilation as well as by the maintenance of adequate coronary perfusion pressure (CPP). Situations accompanied by an increase in myocardial O2 demand (tachycardia, increased ventricular wall tension, increased myocardial contractility) must be avoided. The same applies for a decrease in diastolic aortic pressure due to reduction of systemic vascular resistance. Continuous application of noradrenaline increases whole-body anemia tolerance. Sympathicolysis via beta-receptor blockade or thoracic epidural anesthesia leaves anemia tolerance unaffected.[35]
(3) Inspiratory oxygen fraction (FiO2): ventilation with supranormal FiO2 (hyperoxic ventilation) increases the physically dissolved part of arterial O2 content. Physically dissolved plasma O2 is biologically highly available and covers up to 75% of whole-body O2 demand in conditions of extreme dilutional anemia. Experimental and clinical studies clearly demonstrate that hyperoxic ventilation effectively increases anemia tolerance and creates an important margin of safety for global, myocardial, gastrointestinal, and cerebral tissue oxygenation.
(4) Muscular relaxation: striated skeletal muscles amount to about 30% of the total body mass. Muscular relaxation significantly reduces the whole body’s O2 demand and increases anemia tolerance.
(5) Body temperature: hypothermia reduces the whole body’s O2 demand. In an animal experiment, anesthetized hypothermic pigs died at lower Hb than normothermic control animals. Owing to the negative impact of hypothermia on coagulation, however, the intentional induction of hypothermia in bleeding patients in order to increase anemia tolerance cannot be recommended.
(6) Choice of anesthetic drugs and depth of anesthesia: almost all anesthetic drugs investigated suppress the CO response to dilutional anemia. In animal experiments, a dose-related reduction in anemia tolerance could be demonstrated for halothane, enflurane, isoflurane, ketamine, propofol, etomidate, and pentobarbital. Inadequately profound anesthesia has to be avoided to the same extent as too light anesthesia (increased sympathetic tone and O2 consumption).
(7) Choice of the infusion solution: the choice of the solution infused to compensate blood losses seems to influence anemia tolerance. While 3% gelatin and 6% hydroxyethyl starch (HES) 200.000/0.5 did not affect anemia tolerance in animals, an advantage could be demonstrated for 6% HES 130.000/0.4 over 3.5% gelatin, 6% HES 450.000/0.7, and Ringer’s lactate. Application of infusion solutions with intrinsic O2 transport capacity (artificial O2 carriers based on human or bovine Hb; perfluorocarbons) increased anemia tolerance in animals and patients.[36] Unfortunately, no artificial O2 carrier is approved in the United States or Europe.
Coagulation management
In analogy to dilutional anemia, the replacement of blood losses with crystalloid and/or colloid infusion solutions causes dilution of all components of the coagulatory and fibrinolytic system. This finally results in “dilutional coagulopathy.”
It has been demonstrated in animal experiments and in human patients that the first procoagulatory factor to decrease below a level requiring substitution is fibrinogen (i.e. <1.5 g/l), followed by the activity of coagulation factors of the prothrombin complex, and finally the platelet count.[37,38] Fibrinogen and fibrin are essential for clot formation at the site of the vessel damage. The lack of fibrinogen results in the formation of unstable clots that are unable to resist blood flow and to stop bleeding.[39] In addition, plasma fibrinogen concentration is reduced by infusion of HES-based colloidal infusion solutions.[40] Because of problems related to its processing, fresh frozen plasma (FFP) contains coagulation factors at a reduced concentration. This means that FFP has to be infused in large quantities to stabilize or improve coagulation, often resulting in transfusion-related cardiac overload (TACO). The infusion of small amounts of FFP to improve coagulation has to be considered meaningless.[41]
In the situation of the massively bleeding patient, it is difficult or even impossible to monitor and detect the “critical” reduction of the different components of the coagulation system. Moderate dynamics of blood losses allow differentiated coagulation management on the basis of results obtained from viscoelastic coagulation tests (thromboelastometry/-graphy),[42] but the lack of this analytic measure and/or the presence of more severe blood losses require “empirical” coagulation management based on the intensity and dynamics of the blood loss and the quality of bleeding (“diffuse” bleeding tendency, rebleeding of formerly “dry” wounds).
After the establishment of procoagulatory general conditions (e.g. normothermia, normal pH) the following measures are taken, following a graduated scheme (Figure 6.3): the early and calculated administration of fibrinogen concentrate, prothrombin complex concentrate (PCC), vitamin-K-dependent coagulation factor concentrate (PPSB), FFP, and antifibrinolytic drugs (e.g. tranexamic acid).[43] The administration of desmopressin [44] mobilizes stocks of factor VIII, increases the activity of von Willebrand factor, and stimulates aggregatory platelet function. Clot stability may be additionally increased by application of factor XIII concentrate.[45] In cases of massive bleeding, the early administration of recombinant human factor VIIa (“off-label use”) may be considered [46] (Table 6.2). However, the maximum effectiveness of this substance depends on stable general conditions for coagulation, in particular normothermia, normal pH, and adequate platelet count.
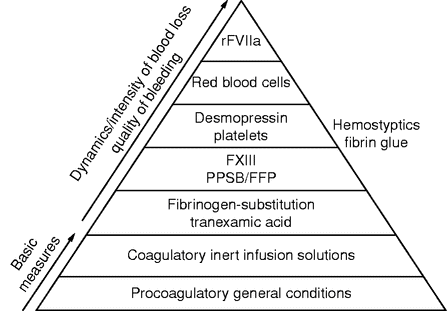
Graduated scheme (“coagulation pyramid”) for the stabilization of coagulation in cases of major blood loss. The time sequence of therapeutic interventions beyond the basic measures is based on the intensity and dynamics of blood losses and on the quality of bleeding.

Full access? Get Clinical Tree
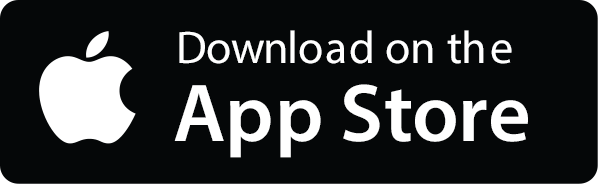
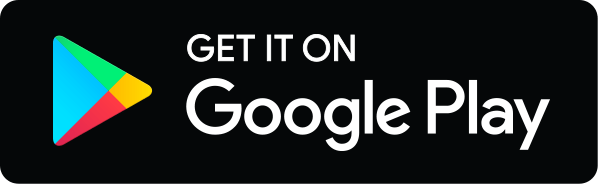